Untitled
BioFactors 25 (2005) 97–107
Coenzyme Q10 protects SHSY5Y neuronalcells from beta amyloid toxicity andoxygen-glucose deprivation by inhibiting theopening of the mitochondrial permeabilitytransition pore
Geng Lia
,∗, Liang-Yu Zoua
,b, Chun-Mei Caoc and Edward S. Yanga
a
The Jockey Club MRI Centre, The University of Hong Kong, Hong Kong, China
b
Department of Neurology, The First Affiliated Hospital, Harbin Medical University, China
c
Institute of Molecular Medicine, Peking University, China
Abstract. Coenzyme Q10 (CoQ10) is an essential biological cofactor which increases brain mitochondrial concentration and
exerts neuroprotective effects. In the present study, we exposed SHSY5Y neuroblastoma cells to neurotoxic beta amyloid
peptides (A
β) and oxygen glucose deprivation (OGD) to investigate the neuroprotective effect of 10
μM CoQ10 by measuring
(i) cell viability by the MTT assay, (ii) opening of the mitochondrial permeability transition pore via the fluorescence intensity
of calcein-AM, and (iii) superoxide anion concentration by hydroethidine. Cell viability (mean
± S.E.M.) was 55.5
± 0.8%
in the group exposed to A
β + OGD, a value lower than that in the A
β or OGD group alone (
P < 0.01). CoQ10 had no
neuroprotective effect on cell death induced by either A
β or OGD, but increased cell survival in the A
β + OGD group to 57.3
± 1.7%, which was higher than in the group treated with vehicle (
P < 0.05). The neuroprotective effect of CoQ10 was blockedby administration of 20
μM atractyloside. Pore opening and superoxide anion concentration were increased in the A
β + OGDgroup relative to sham control (
P < 0.01), and were attenuated to the sham level (
P > 0.05) when CoQ10 was administered.
Our results demonstrate that CoQ10 protects neuronal cells against A
β neurotoxicity together with OGD by inhibiting theopening of the pore and reducing the concentration of superoxide anion.
Keywords: Coenzyme Q10, Beta amyloid, oxygen-glucose deprivation, mitochondrial permeability transition pore, superoxideanion
Stroke and Alzheimer's disease (AD) are the most prevalent age-related neurological diseases in
humans. The mechanisms of neuronal injury and neuronal death overlap in both diseases [9,21,34].
Invivo studies have demonstrated that susceptibility to ischemic brain damage increases in transgenic miceoverexpressing amyloid precursor protein (APP) or with a presenilin-1 (PS1) mutation [31,51]. Increased
∗Address for correspondence: Geng Li, The Jockey Club MRI Centre, The University of Hong Kong, Room 204, Chow Yei
Ching Bldg., Pokfulam Road, Hong Kong. Tel.: +852 2293 0305; Fax: +852 2540 6215; E-mail:
[email protected].
0951-6433/05/$17.00 2005 – IOS Press and the authors. All rights reserved
G. Li et al. / Coenzyme Q10 protects SHSY5Y neuronal cells from beta amyloid toxicity
oxidative stress and impairment of mitochondrial energy metabolism are involved in the pathogenesis ofAD and cerebral ischemia [1,3,25,29,40,47,49,50]. Reduced activities of complex I, II/III and IV of themitochondrial electron transport chain were observed in AD patients and in ischemic conditions [2,32,49]. Coenzyme Q10 (CoQ10) is an essential cofactor produced endogenously and also provided in thefood chain. Mitochondrial CoQ10 level is correlated with complex I, II/III and IV activities [43], whileadministration of CoQ10 increases brain mitochondrial concentration and exerts neuroprotective effectsin some neurodegenerative diseases and in cerebral ischemia [10,15,30,38,39,44,48]. To date, there arefew reports of therapeutic effects of CoQ10 in AD, although mitochondrial abnormalities have beenfound in this disease [47]. In the present study, we exposed SHSY5Y neuroblastoma cells to neurotoxicbeta amyloid peptides (A
β) and oxygen-glucose deprivation (OGD) to build an
in vitro model of ADwith ischemia [8,13], and investigated the neuroprotective effect of the antioxidant CoQ10 by measuringcell viability, opening of the mitochondrial permeability transition pore (abbreviated to "the pore" below)and the concentration of the superoxide anion radical.
2. Material and methods
2.1. Materials and chemicals
All culture medium supplements (minimum essential medium (MEM), Dulbecco's modified Eagle
medium with high glucose (DMEM), glucose-free DMEM, penicillin, streptomycin, fetal bovine serum(FBS), Ham's F12 medium, 0.5% trypsin, 0.53 M ethylenediaminetetraacetic acid (trypsin-EDTA)and trypan blue) were obtained from Life Technologies (Gibco BRL, MD, USA). Dimethyl sulfox-ide (DMSO), poly-L-lysine, paraformaldehyde, A
β1–42, atractyloside (Atr), the lactate dehydrogenase(LDH) kit and coenzyme Q10 were obtained from Sigma-Aldrich (St. Louis, MO, USA). Hydroethidine(HEt) was purchased from Molecular Probes and calcein-AM was from Biotium Inc. The cell prolifer-ation kit with 3-[4,5-dimethylthiazol-2-yl]-2,5-diphenyltetrazolium bromide (MTT) was obtained fromRoche Diagnostics. The human neuroblastoma cell line SH-SY5Y was purchased from ATCC (VA,USA).
2.2. Cell culture
The stock vial of the SHSY5Y cell line was kept in liquid nitrogen at vapor phase. The vial of cells
was first thawed at 37
◦C, then transferred to a 75 cm2 culture flask containing 12.15 mL of culturemedium and cultured for 1 to 2 weeks [37]. When the cells became confluent, they were dissociatedusing trypsin-EDTA and sub-cultured at a density of 105 cells/well. The culture medium was changedtwice a week. For the cell viability experiment, cultured cells were reseeded into 96-well culture platesat a density of 105 cells/well. All cultures were incubated at 37
◦C under 5% CO2 and 95% air (v/v) at90% humidity.
2.3. Experimental protocols
A
β1–42 was dissolved at 50
μM in sterile ultra-pure water (Milli-Q standard; Millipore, Watford,
UK) and kept frozen until use; the final concentration of A
β was 5
μM [46]. CoQ10 was dissolved at1.0 M in 100% (w/v) DMSO and kept at 4
◦C until use; the final concentration was 10
μM [33]. Atr wasdissolved at 1.0 M in sterile ultra-pure water and kept at 4
◦C; the final concentration was 20
μM [6].
G. Li et al. / Coenzyme Q10 protects SHSY5Y neuronal cells from beta amyloid toxicity
Fig. 1. Experimental protocols. Atr: 20
μM atractyloside; A
β: 5
μM beta amyloid; OGD: oxygen-glucose deprivation; Q10:10
μM CoQ10; LDH: lactate dehydrogenase.
The experimental groups and protocols are summarized in Fig. 1. Cells were first exposed to 5
μM A
β(A
β and A
β + OGD groups) or to sterile ultra-pure water (sham and OGD groups) for 24 hr. Then cellsin the OGD and A
β + OGD groups were exposed to OGD (glucose-free DMEM plus anoxia) for 1 hr,while the sham and A
β groups were left alone. During the process of OGD, A
β was added to the A
β andA
β + OGD groups, and sterile ultra-pure water was added to the sham and OGD groups. Pore openingand superoxide anion concentration were measured at the end of OGD exposure. After 1 hr of OGD, allcells were returned to normal, normoxic medium and incubated for a further 24 hr, at which time cellviability was measured. For the CoQ10 treatment, all the above procedures were followed, except thatvehicle (2% DMSO), 10
μM CoQ10 or 10
μM CoQ10 + 20
μM Atr were added to the four groups fromthe beginning of exposure to A
β to the end of OGD.
2.4. In vitro OGD model of ischemia
Oxygen-glucose deprivation was achieved using published methods [13]. Briefly, 4 days after subcul-
ture, pretreatments (described in the experimental protocols) were carried out for 24 hr. Then the culturemedium of cells in the OGD and A
β + OGD groups was changed to glucose-free DMEM containing thedrugs and placed in an anaerobic chamber flushed with 5% CO 2 and 95% N2 (v/v). The same anaerobicgas mixture was bubbled through the glucose-free DMEM to "deoxygenate" the medium. The cellcultures within the anaerobic chamber were kept in a humidified incubator at 37
◦C for 1 hr. The oxygenconcentration was
< 0.8% throughout OGD, as monitored by an oxygen meter (OX 2000, Oldham,Arras Cedex, France). To terminate OGD, the culture medium was changed to normal medium beforereturning the cells to the normoxic incubating conditions. In the sham and A
β groups, the cell cultureswere subjected to the same experimental procedures, but were not exposed to glucose-free DMEM plusanoxia.
2.5. Assessment of cell viability
Cellular viability was assessed using the mitochondrial assay kit according to the manufacturer's
instructions (Boehringer Mannheim) and published methods [18]. Briefly, 10
μl of the MTT labeling
G. Li et al. / Coenzyme Q10 protects SHSY5Y neuronal cells from beta amyloid toxicity
reagent at a final concentration of 0.5 mg/mL was added to each well 24 hr after the termination of OGDand the cells were incubated at 37
◦C under 5% CO2 and 95% air (v/v) at 90% humidity for 4 hr to allowformation of purple formazan crystals. Four hours later, 100
μl of the solubilization reagent was addedto each well before overnight incubation. Finally, the spectrophotometric absorbance of the solubilizedpurple formazan crystals was measured using a microplate reader (Model 550, Bio-Rad, Hercules, CA,USA) at an absorbance wavelength of 570 nm and a reference wavelength of 670 nm [18]. All MTTresults were normalized and expressed as percentages of the average optical density reading from thesham-OGD group.
2.6. Determination of neuronal injury by LDH efflux
The culture medium was collected after 24 hr of normoxia, and LDH was spectrophotometrically
assayed using a kit. LDH activity was expressed as units per milliliter [6].
2.7. Measurement of mitochondrial permeability transition pore opening and superoxide anion
Cells were counted and plated at a density of 2
× 105 cells per well on 24-well plates with 12 mm glass
coverslips precoated with poly-L-lysine (10
μg/mL). Cells were pretreated as described above. Afterpretreatment, culture medium was removed and cells were washed in PBS. Then the cells were incubatedwith 10
μM HEt [22] or 2
μmol/L calcein-AM in the presence of 4 mmol/L of cobalt chloride (CoCl2) [12]for 30 min at 37
◦C in a dark and humidified incubator under an atmosphere of 5% CO 2 to measure theintracellular concentration of superoxide anion radicals or pore opening. After loading, the cells werewashed three times with medium, followed by 1 hr of OGD. As a negative control, cells were incubatedin medium lacking the fluorescent dye. After OGD, the cells were incubated for 1 hr in 4% (wt/vol)paraformaldehyde in PBS for fixation [28]. After washing, the cells on the coverslips were mounted ontoglass slides using permount. These slides were examined with a microscope (Axioskop 2 plus, Zeiss,Thornwood, NY, USA) under fluorescent light. The fluorescence (red signal) from superoxide anionswas examined at an excitation wavelength of 510–550 nm and an emission wavelength
> 580 [4]. Thecalcein-AM was exited with 488 nm light and the emitted fluorescence (green signal) was measuredat 510–525 nm; increased fluorescence intensity indicated enhanced opening of the pore [19]. Afterpreparation, digital images of cells were taken using a video camera connected to the microscope. Allthe images from each experiment were captured under identical settings of exposure time, gain andoffset, and further analyzed using an image analysis system. The fluorescence intensity values fromthree different fields of view per slide were determined using MetaMorph software (MetaMorph imagingsystem 4.01) and the mean values were calculated [22]. The mean intensity represents the total intensityminus background, divided by the total target area. Background was calculated as the mean of all pixelsoutside a cell with the lowest optical density. Values are the result of the analysis of at least six images perexperimental condition, with at least 20 cells per experimental condition. Each condition was repeatedtwice. The results are expressed as relative fluorescence intensity, which represent the percentages ofoptical densities relative to sham control.
2.8. Data analysis
Numerical data are expressed as mean
± S.E.M. Data were pooled from five independent sets of
studies for cultured SHSY5Y neuroblastoma cells. The data were analyzed using one-way ANOVAfollowed by the Student-Newman-Keuls'
post hoc test. A two-tailed P value of 0.05 or less was taken toindicate statistical significance.
G. Li et al. / Coenzyme Q10 protects SHSY5Y neuronal cells from beta amyloid toxicity
it/mnU 30DH (L 20
Fig. 2. LDH measurements in SHSY5Y cells. A
β: 5
μM beta amyloid; Q10: 10
μM CoQ10; OGD: oxygen-glucose deprivation.
3. Results
3.1. Neuroprotective effect of CoQ10 against OGD and Aβ neurotoxicity in SH-SY5Y cells
Compared with the sham group, A
β, OGD and A
β + OGD had no effect on LDH release from
neuronal cells (
P > 0.05) (Fig. 2). However, A
β, OGD, and A
β + OGD decreased the cell viability (asmeasured by the MTT value) to 63.0
± 3.9%, 68.3
± 6.6% and 55.5
± 0.8%, respectively. Comparedwith the groups exposed to either A
β or OGD, OGD combined with A
β induced more neuron death(
P < 0.01) (Fig. 3A). When 10
μM CoQ10 was administered to the A
β + OGD group, the cell viabilitywas 57.3
± 1.7%, higher than that in the vehicle group (54.6
± 0.8%,
P < 0.05). Nevertheless, the cellviability decreased to 54.9
± 0.9% when 20
μM atractyloside was administered to the A
β + OGD grouptogether with 10
μM CoQ10, i.e. not different from vehicle control (
P > 0.05) (Fig. 3B).
3.2. Effect of CoQ10 on pore opening (
calcein-AM fluorescence)
in SH-SY5Y cells
Compared with sham control, A
β or OGD had no effect on the relative calcein-AM fluorescence
intensity (
P > 0.05), while A
β + OGD significantly increased the intensity (
P < 0.01) (Fig. 4A, B).
However, when 10
μM CoQ10 was administered, the relative calcein-AM fluorescence intensity in theA
β + OGD group and vehicle control decreased to the level of the sham control (
P > 0.05) (Fig. 5A,B).
3.3. Effect of CoQ10 on superoxide anion concentration (
HEt fluorescence)
in SH-SY5Y cells
Compared with sham control, A
β + OGD significantly increased the relative HEt fluorescence intensity
(
P < 0.01). Whereas when 10
μM CoQ10 was administered, the intensity decreased to the level of thesham control (
P > 0.05) (Fig. 6A, B).
In vivo studies have shown that susceptibility to cerebral ischemia increases in transgenic mouse
models of AD [31,51]. Mattson and colleagues also found that the presenilin-1 mutation increasedneuronal vulnerability to hypoxia and glucose deprivation in cell culture [31]. In the present study, an
invitro model of AD combined with ischemia was developed by exposing SHSY5Y human neuroblastoma
G. Li et al. / Coenzyme Q10 protects SHSY5Y neuronal cells from beta amyloid toxicity
Fig. 3. Cell viability. A, SHSY5Y cells treated with A
β, OGD or A
β + OGD relative to sham control = 100%.
∗∗P < 0.01,as compared with the A
β + OGD group. B, SHSY5Y cells treated with A
β + OGD and vehicle, A
β + OGD and CoQ10, orA
β + OGD with CoQ10 + Atr relative to sham. #
P < 0.05, as compared with the vehicle group. Atr: 20
μM atractyloside;A
β: 5
μM beta amyloid; Q10: 10
μM CoQ10.
cells to neurotoxic beta amyloid peptides and oxygen-glucose deprivation [8,13]. Similar to the previousstudy, A
β or OGD alone did not enhance LDH release from neuronal cells [14,42]. In the present study,a combination of the A
β and OGD also had no effect on LDH release. A
β increased the vulnerabilityof the cells to ischemia as manifested by reduced viability. LDH is a cytoplasmic enzyme whosepresence in the culture medium reflects the loss of plasma membrane integrity [24], while MTT assayis to detect the metabolic activity of cells, resulting in different observation of the cell damage causedby a combination of the A
β and OGD. Possible mechanisms include increased oxidative stress andimpairment of mitochondrial energy metabolism [1]. Since CoQ10 is neuroprotective against externaloxidative stress [45], it was administered to cells undergoing A
β treatment and/or OGD to investigate itspotential for neuroprotection in AD with cerebral ischemia. In the present study, although CoQ10 did notprotect SHSY5Y neuroblastoma cells against either A
β neurotoxicity or OGD alone, the viability of cellsexposed to A
β + OGD was significantly increased by treatment with 10
μM CoQ10. The mechanism ofthis neuroprotective effect was investigated by measuring the opening of the mitochondrial permeabilitytransition pore. Pore opening limits mitochondrial calcium load, mediates mitochondrial reactive oxygenspecies (ROS) signaling [12], and triggers apoptosis/necrosis [7,27]. We measured pore opening usingthe calcein/Co2+ imaging technique. Previous studies have investigated the occurrence and the mode ofopening of the MPTP directly in intact cells by monitoring the fluorescence of mitochondrial entrappedcalcein-AM when the cells are coloaded with cobalt chloride and calcein-AM, and exposed to cobaltchloride throughout the experiment [12]. In the current study, the cells were first coloaded with cobaltchloride and calcein-AM to quench the cytoplasmic and nucleic fluorescence. After washing, the cellswere then exposed to OGD and/or A
β treatment for 1 hour, which caused the calcein-AM remained in
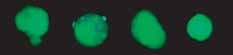
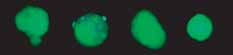
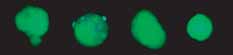
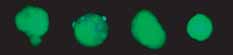
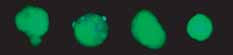
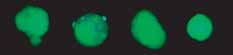
G. Li et al. / Coenzyme Q10 protects SHSY5Y neuronal cells from beta amyloid toxicity
Fig. 4. Calcein fluorescence intensity. A, Photomicrographs showing calcein fluorescence intensity in SHSY5Y single cells fromsham control, 5 μM beta amyloid (Aβ), oxygen-glucose deprivation (OGD), and Aβ + OGD groups. B, Calcein fluorescenceintensity measurements from the groups in A relative to sham control. ∗∗P < 0.01, as compared with the sham group.
the mitochondria to outflow into the cytoplasm. In the sham group, self-quenching induced outflowingof calcein-AM from the mitochondria to the cytoplasm [41]. Warm incubation with calcein-AM can leadto almost exclusive cytosolic distribution of calcein in both rat hepatocytes and rabbit cardiacmyocytes,and under this condition, mitochondria are visualized by confocal microscopy as round and oval voidsabout a micron in diameter in images of green calcein fluorescence [35,36]. Therefore the calcein-AM fluorescence intensity measured after OGD and/or Aβ treatment was the calcein-AM signal in thecytoplasma which had outflown from mitochondria during treatment. The difference of the calcein-AMfluorescence intensity between the treatment and sham group was used to measure the opening of MPTPcaused by treatment. Aβ or OGD alone did not alter the calcein-AM fluorescence intensity, while Aβ
+ OGD increased it. Moreover, the rise in fluorescence intensity decreased significantly when CoQ10was administered. This suggests that inhibition of pore opening is a key point for the neuroprotectiveeffect of CoQ10. Previous studies on heart have showed that the pore opens during the first few minutesof reperfusion and results in myocardial injury [16,17,19,20]. The pore opening was measured at theend of the OGD in this study, thereafter, OGD alone did not enhance pore opening. However, poreopening occurred earlier in OGD if Aβ was present (Fig. 4). At the end of OGD, the CoQ10 treatmentwas completed, so it had no effect on the pore opening caused by the return to normoxia (analogous toreperfusion), but did affect the earlier opening of the pore caused by Aβ + OGD. This may explain whyCoQ10 was not neuroprotective against ischemia, but did protect the cells against OGD + Aβ. In orderto test the hypothesis that CoQ10 protects cells from Aβ + OGD via inhibition of pore opening, wetreated the cells undergoing this protocol with 20 μM atractyloside, the pore opener. Opening the porewith Atr abolished the neuroprotective effect of CoQ10 (Fig. 3B), supporting the hypothesis.
Disruption of the mitochondrial respiratory chain induces overproduction of reactive oxygen species
(ROS), leading to oxidative stress and the activation of apoptotic mediators [5]. The overproduced ROS,including the superoxide anion, are involved in neuronal cell death in acute injury of central nervoussystem and in chronic neurodegenerative diseases [11,26]. In the present study, we observed an increase insuperoxide anion production with Aβ + OGD, and this overproduction decreased significantly by CoQ10administration. Past research has shown that mitochondria actively generate ROS such as superoxide
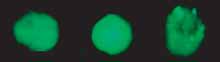
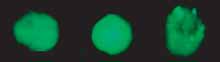
G. Li et al. / Coenzyme Q10 protects SHSY5Y neuronal cells from beta amyloid toxicity
Fig. 5. Calcein fluorescence intensity and CoQ10. A, Photomicrographs showing calcein fluorescence intensity in sham control,5 μM beta amyloid (Aβ) with oxygen-glucose deprivation (OGD) and vehicle, and Aβ + OGD with 10 μM CoQ10 groups.
B, Calcein fluorescence intensity values from the groups in A relative to sham control. ∗∗P < 0.01, as compared with shamgroup.
Fig. 6. Hydroethidine (HEt) fluorescence intensity. A, Photomicrographs showing calcein fluorescence intensity in singleSHSY5Y cells from sham control, 5 μM beta amyloid with oxygen-glucose deprivation (Aβ + OGD) and vehicle, and Aβ +OGD with 10 μM CoQ10 groups. B, Calcein fluorescence intensity values from treatment groups in A relative to sham control.
∗∗P < 0.01, as compared with the sham group.
anions and hydrogen peroxide [23], and CoQ10 protects cells at the mitochondrial level [45]. Therefore,CoQ10 may protect cells from Aβ + OGD by inhibiting pore opening, which reduces the production ofsuperoxide anions and thereafter attenuates the oxidative stress and the activation of apoptotic mediators.
In summary, CoQ10 offers neuroprotection at the mitochondrial level against beta amyloid neuro-
toxicity and ischemia-like damage caused by oxygen-glucose deprivation. The underlying mechanismsinclude inhibition of the opening of the mitochondrial permeability transition pore, which reduces the
G. Li et al. / Coenzyme Q10 protects SHSY5Y neuronal cells from beta amyloid toxicity
production of superoxide anion. CoQ10 can therefore be considered as a potential therapeutic agent forAD with cerebral ischemia.
This study was partially supported by Rejuvenis and the Hong Kong Jockey Club Charities Trust. We
thank Dr. Iain Bruce for reading the manuscript.
A.Y. Abramov, L. Canevari and M.R. Duchen, Beta-Amyloid Peptides Induce Mitochondrial Dysfunction and Oxidative
Stress in Astrocytes and Death of Neurons through Activation of NADPH Oxidase, J. Neurosci. 24 (2004), 565–575.
K.L. Allen, A. Almeida, T.E. Bates and J.B. Clark, Changes of respiratory chain activity in mitochondrial and synapto-
somal fractions isolated from the gerbil brain after graded ischaemia, J. Neurochem. 64 (1995), 2222–2229.
G. Benzi and A. Moretti, Are reactive oxygen species involved in Alzheimer's disease? Neurobiol. Aging 16 (1995),
661–674.
V.P. Bindokas, J. Jordan, C.C. Lee and R.J. Miller, Superoxide production in rat hippocampal neurons: selective imaging
with hydroethidine, J. Neurosci. 16 (1996), 1324–1336.
C.D. Bortner and J.A. Cidlowski, Cellular mechanism for the repression of apoptosis, Annu. Rev. Pharmacol. Toxicol. 42
(2002), 259–281.
C.M. Cao, Q. Xia, Q. Gao, M. Chen and T.M. Wong, Calcium-activated potassium channel triggers cardioprotection of
ischemic preconditioning, J. Pharmacol. Exp. Ther. 312 (2005), 644–650.
M. Crompton, The mitochondrial permeability transition pore and its role in cell death, Biochem. J. 341 (1999), 233–249.
S. Estus, C.V. Rooyen, S. Wright, E.F. Brigham, M. Wogulis and R.E. Rydel, Aggregated Amyloid-b Protein Induces
Cortical Neuronal Apoptosis and Concomitant "Apoptotic" Pattern of Gene Induction, J. Neurosci. 17 (1997), 7736–7745.
D.A. Evans, H.H. Funkenstein, M.S. Albert, P.A. Scherr, N.R. Cook, M.J. Chown, L.E. Hebert, C.H. Hennekens and J.O.
Taylor, Prevalence of Alzheimer's disease in a community population of older persons. Higher than previously reported,
JAMA 262 (1989), 2551–2556.
R.J. Ferrante, O.A. Andreassen, A. Dedeoglu, K.L. Ferrante, B.G. Jenkins, S.M. Hersch and M.F. Beal, Therapeutic
effects of coenzyme Q10 and remacemide in transgenic mouse models of Huntington's disease, J. Neurosci. 22 (2002),
1592–1599.
M. Gerlach, P. Riederer and M.B. Youdim, Molecular mechanisms for neurodegeneration. Synergism between reactive
oxygen species, calcium, and excitotoxic amino acids, Adv. Neurol. 69 (2005), 177–194.
T. Gillessena, C. Grasshoffb and L. Sziniczb, Mitochondrial permeability transition can be directly monitored in living
neurons, Biomed. Pharmacother. 56 (2002), 186–193.
M.P. Goldberg and D.W. Choi, Combined oxygen and glucose deprivation in cortical cell culture: calcium-dependent
and calcium-independent mechanisms of neuronal i, J. Neurosci. 13 (1993), 3510–3524.
J.S. Gong, N. Sawamura, K. Zou, J. Sakai, K. Yanagisawa and M. Michikawa, Amyloid beta-protein affects cholesterol
metabolism in cultured neurons: implications for pivotal role of cholesterol in the amyloid cascade, J. Neurosci. Res. 70
(2002), 438–446.
P. Grieb, M.S. Ryba, J. Sawicki and S.J. Chrapusta, Oral coenzyme Q10 administration prevents the development of
ischemic brain lesions in a rabbit model of symptomatic vasospasm, Acta Neuropathol. (Berl) 94 (1997), 363–368.
E.J. Griffiths and A.P. Halestrap, Mitochondrial non-specific pores remain closed during cardiac ischaemia, but open
upon reperfusion, Biochem. J. 307 (1995), 93–98.
A.P. Halestrap, K.P.M., J.S., K. Woodfield, Elucidating the molecular mechanism of the permeability transition pore and
its role in reperfu-sion injury of the heart, Biochim. Biophys. Acta. 1366 (1998), 79–94.
M.B. Hansen, S.E. Nielsen and K. Berg, Re-examination and further development of a precise and rapid dye method for
measuring cell growth/cell kill, J. Immunol. Methods 119 (1989), 203–210.
D.J. Hausenloy, H.L. Maddock, G.F. Baxter and D.M. Yellon, Inhibiting mitochondrial permeability transition pore
opening: a new paradigm for myocardial preconditioning? Cardiovasc. Res. 55 (2002), 534–543.
D.J. Hausenloy, A. Tsang and D.M. Yellon, The reperfusion injury salvage kinase pathway: a common target for both
ischemic preconditioning and postconditioning, Trends Cardiovasc. Med. 15 (2005), 69–75.
L.E. Hebert, L.A. Beckett, P.A. Scherr and D.A. Evans, Annual incidence of Alzheimer disease in the United States
projected to the years 2000 through 2050, Alzheimer Dis. Assoc. Disord. 15 (2001), 169–173.
G. Li et al. / Coenzyme Q10 protects SHSY5Y neuronal cells from beta amyloid toxicity
S.V. Kalivendi, S. Kotamraju, S. Cunningham, T. Shang, C.J. Hillard and B. Kalyanaraman, 1-Methyl-4-phenylpyridinium
(MPP+)-induced apoptosis and mitochondrial oxidant generation: role of transferrin-receptor-dependent iron and hy-
drogen peroxide, Biochem. J. 371 (2003), 151–164.
K. Kannan and S.K. Jain, Oxidative stress and apoptosis, Pathophysiology 7 (2000), 153–163.
I. Kansau, C. Berger, M. Hospital, R. Amsellem, V. Nicolas, A.L. Servin and M.F. Bernet-Camard, Zipper-like internal-
ization of Dr-positive Escherichia coli by epithelial cells is preceded by an adhesin-induced mobilization of raft-associated
molecules in the initial step of adhesion, Infect. Immun. 72 (2004), 3733–3742.
R. Katzman, Alzheimer's disease, N. Engl. J. Med. 314 (1986), 964–973.
J.P. Kehrer, Free radicals as mediators of tissue injury and disease, Crit. Rev. Toxicol. 23 (1993), 21–48.
G. Kroemer, D.B., M. Resche-Rigon, The mitochondrial death/life regulator in apoptosis and necrosis, Annu. Rev. Physiol.
60 (1998), 619–641.
M.O. Lopez-Figueroa, C. Caamano, R. Marin, B. Guerra, R. Alonso, M.I. Morano, H. Akil and S.J. Watson, Characteri-
zation of basal nitric oxide production in living cells, Biochimica. et Biophysica. Acta (BBA) -Molecular Cell Research
1540 (2001), 253–264.
M.P. Mattson, Cellular actions of beta-amyloid precursor protein and its soluble and fibrillogenic derivatives, Physiol.
Rev. 77 (1997), 1081–1132.
R.T. Matthews, L. Yang, S. Browne, M. Baik and M.F. Beal, Coenzyme Q10 administration increases brain mitochondrial
concentrations and exerts neuroprotective effects, Proc. Natl. Acad. Sci. USA 95 (1998), 8892–8897.
M.P. Mattson, H. Zhu, J. Yu and M.S. Kindy, Presenilin-1 mutation increases neuronal vulnerability to focal ischemia in
vivo and to hypoxia and glucose deprivation in cell culture: involvement of perturbed calcium homeostasis, J. Neurosci.
20 (2000), 1358–1364.
I. Maurer, S. Zierz and H.J. Moller, A selective defect of cytochrome c oxidase is present in brain of Alzheimer disease
patients, Neurobiol. Aging 21 (2000), 455–462.
T. Menke, G. Gille, F. Reber, B. Janetzky, W. Andler, R.H. Funk and H. Reichmann, Coenzyme Q10 reduces the toxicity
of rotenone in neuronal cultures by preserving the mitochondrial membrane potential, Biofactors 18 (2003), 65–72.
J.C. Monfort, A population-based study of dementia in 85-year-olds, N. Engl. J. Med. 329 (1993), 63–64.
A.L. Nieminen, A.K. Saylor, S.A. Tesfai, B. Herman and J.J. Lemasters, Contribution of the mitochondrial permeability
transition to lethal injury after exposure of hepatocytes to tbutylhydroperoxide, Biochem. J. 307 (1995), 99–106.
H. Ohata, E. Chacon, S.A. Tesfai, I.S. Harper, B. Herman and J.J. Lemasters, Mitochondrial Ca21 transients in cardiac
myocytes during the excitation-contraction cycle: effects of pacing and hormonal stimulation, J. Bioenerg. Biomembr.
30 (1998), 207–222.
G. Olivieri, M. Novakovic, E. Savaskan, F. Meier, G. Baysang, M. Brockhaus and F. Muller-Spahn, The effects of beta-
estradiol on SHSY5Y neuroblastoma cells during heavy metal induced oxidative stress, neurotoxicity and beta-amyloid
secretion, Neuroscience 113 (2002), 849–855.
R.P. Ostrowski, Effect of coenzyme Q(10) on biochemical and morphological changes in experimental ischemia in the
rat brain, Brain Res. Bull. 53 (2000), 399–407.
R.P. Ostrowski, Effect of coenzyme Q10 (CoQ10) on superoxide dismutase activity in ET-1 and ET-3 experimental
models of cerebral ischemia in the rat, Folia. Neuropathol. 37 (1999), 247–251.
W.D. Parker, Jr., J. Parks, C.M. Filley and B.K. Kleinschmidt-DeMasters, Electron transport chain defects in Alzheimer's
disease brain, Neurology 44 (1994), 1090–1096.
V.G. Petronilli, M. Miotto, M. Canton, M. Brini, R. Colonna, P. Bernardi and F. Di Lisa, Transient and long-lasting
openings of the mitochondrial permeability transition pore can be monitored directly in intact cells by changes in
mitochondrial calcein fluorescence, Biophys. J. 6 (1999), 725–734.
A. Scorziello, C. Pellegrini, L. Forte, A. Tortiglione, A. Gioielli, S. Iossa, S. Amoroso, R. Tufano, G. Di Renzo and L.
Annunziato, Differential vulnerability of cortical and cerebellar neurons in primary culture to oxygen glucose deprivation
followed by reoxygenation, J. Neurosci. Res. 63 (2001), 20–26.
C.W. Shults, R.H. Haas, D. Passov and M.F. Beal, Coenzyme Q10 levels correlate with the activities of complexes I and
II/III in mitochondria from parkinsonian and nonparkinsonian subjects, Ann. Neurol. 42 (1997), 261–264.
C.W. Shults, D. Oakes, K. Kieburtz, M.F. Beal, R. Haas, S. Plumb, J.L. Juncos, J. Nutt, I. Shoulson, J. Carter, K.
Kompoliti, J.S. Perlmutter, S. Reich, M. Stern, R.L. Watts, R. Kurlan, E. Molho, M. Harrison and M. Lew, Effects
of coenzyme Q10 in early Parkinson disease: evidence of slowing of the functional decline, Arch. Neurol. 59 (2002),
1541–1550.
M. Somayajulu, S. McCarthy, M. Hung, M. Sikorska, H. Borowy-Borowski and S. Pandey, Role of mitochondria in
neuronal cell death induced by oxidative stress; neuroprotection by Coenzyme Q10, Neurobiol. Dis. 18 (2005), 618–627.
K.C. Suen, K.F. Lin, W. Elyaman, K.F. So, R.C. Chang and J. Hugon, Reduction of calcium release from the endoplasmic
reticulum could only provide partial neuroprotection against beta-amyloid peptide toxicity, J. Neurochem. 87 (2003),
1413–1426.
G. Li et al. / Coenzyme Q10 protects SHSY5Y neuronal cells from beta amyloid toxicity
P.A. Trimmer, P.M. Keeney, M.K. Borland, F.A. Simon, J. Almeida, R.H. Swerdlow, J.P. Parks, W.D. Parker, Jr. and J.P.
Bennett, Jr., Mitochondrial abnormalities in cybrid cell models of sporadic Alzheimer's disease worsen with passage in
culture, Neurobiol. Dis. 15 (2004), 29–39.
Y. Tsukahara, A. Wakatsuki and Y. Okatani, Antioxidant role of endogenous coenzyme Q against the ischemia and
reperfusion-induced lipid peroxidation in fetal rat brain, Acta Obstet. Gynecol. Scand. 78 (1999), 669–674.
K. Veitch, A. Hombroeckx, D. Caucheteux, H. Pouleur and L. Hue, Global ischaemia induces a biphasic response of the
mitochondrial respiratory chain. Anoxic pre-perfusion protects against ischaemic damage, Biochem. J. 281(Pt 3) (1992),
709–715.
D.S. Warner, H. Sheng and I. Batinic-Haberle, Oxidants, antioxidants and the ischemic brain, J. Exp. Biol. 207 (2004),
3221–3231.
F. Zhang, C. Eckman, S. Younkin, K.K. Hsiao and C. Iadecola, Increased susceptibility to ischemic brain damage in
transgenic mice overexpressing the amyloid precursor protein, J. Neurosci. 17 (1997), 7655–7661.
Source: http://www.antiaging.org.hk/research/bio00826.PDF
Journal of Evolutionary Biology Research Vol. 2 (1), pp. 7-14, December 2010 Available online at http://www.academicjournals.org/jebr ISSN 2141-6583 ©2010 Academic Journals Ful Length Research Paper The effect of magnetic field on the physical, chemical and microbiological properties of the lake water in Saudi Arabia
HHS Public AccessAuthor manuscript Eur J Pharm Biopharm. Author manuscript; available in PMC 2016 May 09. Published in final edited form as: Eur J Pharm Biopharm. 2015 September ; 95(Pt B): 239–249. doi:10.1016/j.ejpb.2015.02.013. Intracellular delivery of dendrimer triamcinolone acetonide conjugates into microglial and human retinal pigment epithelial