The title of your paper goes here
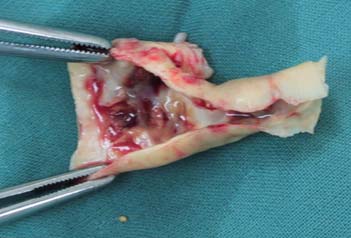
Fifth International Conference on CFD in the Process Industries
CSIRO, Melbourne, Australia
13-15 December 2006
A STUDY OF FLUID AND STRUCTURE INTERACTION IN A CAROTID
BIFURCATION
Kurt LIFFMAN1 and Anh BUI2
1 School of Mathematical Sciences, Monash University, Clayton, Victoria
2 CSIRO Manufacturing & Materials Technology, Highett, Victoria
ABSTRACT
intima. The shear stress causes micro-hemorrhages in the
In this work a study of the deformation of a carotid
sub-intimal planes and it is the healing of these that leads
bifurcation model subjected to blood pressure is presented.
to lesions subject to repeated insult and growth. The
A realistic model of the carotid bifurcation wall consisted
integrity and inherent health of the wall of the intima
of two layers to represent the multiplayer structure
involving all of the above theories of atherogenesis, then
(intima/media/adventitia) of a typical elastic artery was
contributes to acceleration of the lesions or rate
developed. The flow inside the carotid bifurcation and the
modification with therapy.
blood pressure on the arterial wall were altered by an
Some plaques are stable and asymptomatic whilst others
obstacle located in the large bulb and blocking 20% of the
are more likely to produce symptoms due to acute local
cross-section area. A three-dimensional finite element
changes such as plaque rupture ulceration or
model of the carotid bifurcation was created. The relative
hemorrhage.10 Fatigue fractures due to wall stress leading
motion of the layers upon bifurcation deformation was
to intramural haematoma formation for plaque fracture
investigated in this study.
play a role in clinical events. Progression of the atheroma at the plaque site represents an underlying cause for
instability and acute change to plaque morphology. Shear stress points, for example the carotid bifurcation, the
Atherosclerosis is a major cause of morbidity and
origin of vessels and the adductor canal, are recognised
mortality in our society representing up to 50 % of all
sites for the formation of occlusive atheroma related to
deaths in the western world.1 The risk factors for the
clinical events and common sites for treatment (Figure 1).
development of atherosclerosis are well recognised2 as is the inflammatory process within the arterial wall.3,4,5,6
This study looks at the effect of an obstacle which is
However, the initiating process and ongoing stimulus
initiated inside a carotid bifurcation on the flow and the
which perpetuates the repair process and leads to the
biomechanical stress strain behaviour to determine its
formation of the atherosclerotic plaques still requires
influence on atherosclerosis and plaque instability. The
investigation. Better understanding of the precursors is
obstacle represents a stage of the process growth of This
the key to improving prophylactic and management
phenomenon is studied using finite element computational
fluid dynamics (CFD) and stress/strain analysis techniques commonly used in engineering which has been adapted for
There are various hypotheses put forward to explain the
the evaluation of biomechanical and haemodynamic
development of atherosclerosis such as the organised
stresses on the cardiovascular system.
thrombus hypothesis, the monoclonal cell growth hypothesis, the infection-inflammation hypothesis and the
The carotid bifurcation was selected as it is a common
response to injury hypothesis.3 The cause of
region for atherosclerosis with often serious
atherosclerosis is most likely multifactorial with
consequences. It also represents a region of which we
contributions from genetics the environment and local
have intimate knowledge as it is frequently imaged and
factors at the arterial wall interface including
surgically explored.
It is thought that haemodynamics contribute significantly to atherosclerosis as the major areas of plaque formation are at vessel branches. At these branch points there is prolonged turbulent flow1 and low wall shear stress.7,8 These factors allow for prolonged exposure to the damaging effects of turbulence and of atherogenic agents to the endothelium such as lipoproteins which may, when trapped and incorporated into the vessel wall, contribute to the formation of atheromatous plaques.9
The underlying cause for instability and acute changes to
Figure 1: Subintimal haemorrhages at the carotid
plaques is likely to be part of a cycle or an ongoing
bifurcation - causal consequence of atheroma (Image
mechanism from plaque formation to plaque disintegration
courtesy of Professor John Harris, Royal Prince Alfred
and clinical events. This study is based upon the
Hospital, Sydney, Australia).
hypothesis that the known susceptible sites are subject to intramural shear stresses that lead to wall injury, akin to fatigue fractures, that cause bleeding from the penetrating vasa vasorum leading from the adventitial layer to the
MODEL DESCRIPTION
At very high stress/strain above the toe (low strain) and
The analyses of the flow and the structure in this work
stiff (medium strain) regions, individual collagen fibers of
were conducted with use of the so-called ‘one-way
the blood vessel begin to rupture and the vessel
coupling' fluid /structure interaction (FSI) model.
deformation becomes irreversible (plastic deformation).
Initially, a fluid-dynamics simulation was conducted to
The stress/strain values at which the blood vessel rupture
obtain the stress distribution on the carotid wall. The
begins are referred to as ultimate stress/strain. Like the
obtained fluid stresses was subsequently imported into and
stress/strain relationship, the ultimate stress/strain vary
used in a structural analysis.
depending on age, disease history, and mechanical load. The ultimate stress/strain also depends on the dynamics of the mechanical load.
Uniaxial tension tests by Mohan et al. (1982) (performed on human thoracic aorta) gave an approximate value of tensile failure strength in the range from 1.47 MPa to 5.07 MPa. In the work by Monson (2001), ultimate stretch (ratio) of 1.35:1.5 and failure stress of 1.06 to 3.4 MPa were reported for cerebral blood vessels).
The finite element model of the carotid bifurcation has been built based on the measurement data reported by Delfino et al., 1997:
The diameter of the common carotid is 8.92 mm;
One branch of the carotid bifurcation has a large bulb
Figure 2: Carotid bifurcation model with an obstacle
with inner diameter of 9.24 mm. To the outlet of this
blocking the flow in the large bulb.
branch, the artery diameter gradually decreases to
Typical arterial walls consist of three layers: the intima,
media and adventitia, where the predominant connective
In the smaller branch, the carotid diameter slightly
tissue components are smooth muscle, collagen and
decreases from 6.46 mm to 6.38 mm;
elastin. The influence of smooth muscle in determining
The angle between the axes of the internal and
the form of stress strain relationship is relatively small. At
external carotids is 50o;
different levels of stress, the elastin and collagen fibers contribute differently to the total structural function of the
The total arterial wall thickness is 0.7 mm throughout
artery wall, which leads to the so called "biphasic" artery
the carotid bifurcation;
structural behaviour. That is linear isotropic stress-strain
The carotids are "thin-walled" and no residual stress
dependency at low stress and highly non-linear and
in the carotid wall is present in unloaded state.
anisotropic (viscoelastic ) behaviour at high stress (Figure
The modelling and numerical analyses have been carried
2). The mechanical behaviour of a typical abdominal
out using the commercial finite-element ANSYS 6.0 code.
aorta exhibits this " biphasic" nature.13
The fluid-dynamics simulation was carried out using
To qualitatively describe the blood vessel mechanical
53,705 FLUID142 elements. The carotid bifurcation
properties, the vessels can be modelled as purely elastic,
model consists of two layers: the inner one to represent
pseudoelastic, or viscoelastic material. The purely elastic
the combination of tunica intima and media, and the outer
(linear isotropic) assumption is most commonly used to
one to represent tunica adventitia. The inner layer of the
reduce the model complexity, but it can not describe non-
carotid bifurcation wall which includes the obstacle was
linear mechanical behaviour of the artery wall. The
built with 6720 elastic SHELL63 elements. The outer
pseudo-elastic material model is usually based on the use
layer was represented by 6652 SHELL63 elements.
of hyperelastic strain energy functions, which are given in
SHELL63 is a 4-node finite strain element with six
terms of either Green-Lagrangian strain components or
degrees of freedom at each node - translation in the x,y,z
strain invariants. Many experimental measurements (both
directions and rotation about the x,y,z axes. This type of
in-vivo and simulated) have been conducted to determine
element has both bending and membrane capabilities and
the parameters of the strain energy correlations, which
is able to deal with large (geometrically non-linear)
were found to change significantly through aging, disease
displacement. The contact between the two layers was
and change in mechanical load.
modeled using 13,304 ANSYS contact element pairs. In total, 26,676 finite elements were used to model the carotid bifurcation wall and obstacle.
Figure 3: Typical structural behaviour of a blood vessel.
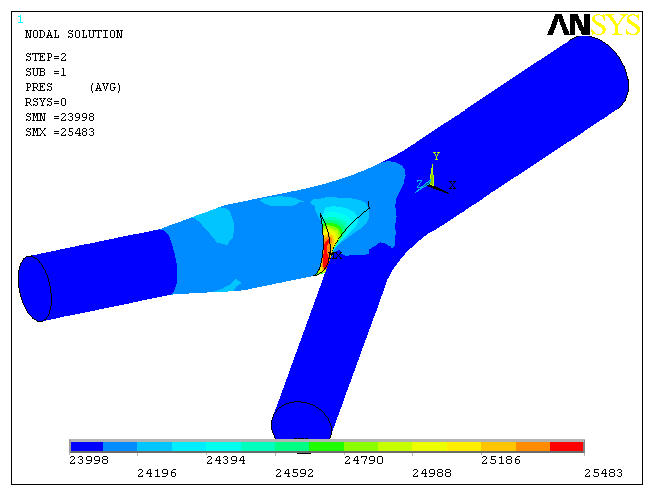
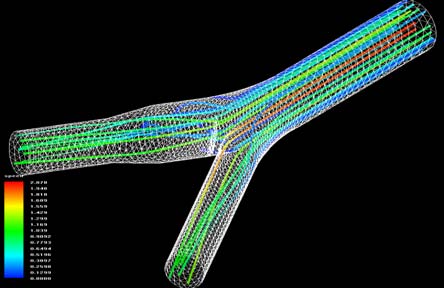
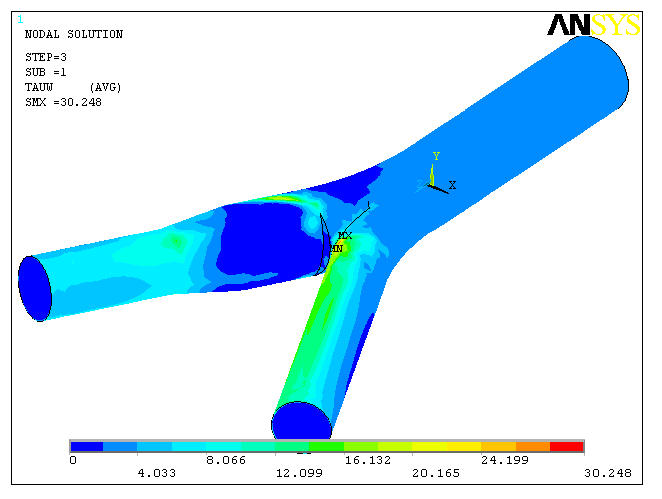
Figure 6: Flow streamlines
Figure 4: Computational mesh used in the CFD study.
Appropriate longitudinal displacement constrains are also applied at the ends of the carotid bifurcation section. The pressure load was initially equal to 1% of the full load and was gradually ramped up to 100%.
CFD simulation
The average blood velocity in the common carotid was assumed to be 1 m/s. Constant systolic pressure of 24 kPa (180 mmHg) was set at the branch outlets. The blood density and viscosity were specified as 1060 kg/m3 and 0.0036 Pa.s, respectively. The flow Reynolds number was about 2650.
Figure 7: Distribution of the wall shear stress.
The computational results are shown in Figures 5-7. The
Structural analysis
obstacle is seen to have a significant effect on the flow
In the realistic elastic arteries (aorta, carotids), the
and the distribution of wall pressure. In figure 5,
thicknesses of the media and adventitia layers are
significant pressure build-up is observed in the flow
comparable. In this analysis, the thicknessses of both inner
before the obstacle. Lower pressure is seen in the flow
and outer layers are assumed to be equal to 0.35mm which
behind the obstacle which can be explained by the
is a half of the total thickness (0.7 mm). In this work,
formation of an area with slow recirculation. The
media and adventitia were modeled as isotropic, linear
computational results indicate that the wall shear stress is
elastic materials. The structural properties reported in the
small compared with the wall pressure. Therefore, only
work by Finet et al., 2002, and Ohayon et al., 2005, were
fluid pressure was used in the following structural
used and listed in Table 1. In this analysis, the carotid
bifurcation is modeled using thin (and plane) shell elements and, hence, the effect of cross-plane deformation is ignored. The stiffness of the obstacle is set to a relatively high value so that its deformation is small.
Estimated equivalent
single layer properties
Table 1: Structural properties used in the analysis (Finet
et al., 2002).
Two simulations were conducted: one with different (case 1) and the other with equivalent single-layer (case 2) structural properties of the wall. Appropriate displacement constrains were applied at the ends of the carotid bifurcation section to prevent its movement. The pressure
Figure 5: Distribution of the blood pressure on the carotid
load was initially set equal to 1% of the full load and
bifurcation wall.
gradually ramped up to 100%. Careful control of ramping step was required to obtain convergence. To accurately predict the deformation of the thin shells the option of "Large Displacement solution" was activated in all simulations.
Figure 8: Von-Mises stress in the inner layer.
Figure 10. Von-Mises stress in the obstacle.
Figure 9. Von-Mises stress in the outer layer.
Figure 11.
Sliding distance.
The structural analysis results shown in Figures 7-11 can be summarized as follows:
The results show that the stress distributions in the
1 The von-Mises equivalent stress, σe is defined from the three
layers are different and depend on the layer structural
principal stresses σ1, σ2, and σ3 , as follows:
properties. In case 2 where the layers have similar
stiffness, deformation of the outer layer is small
([σ −σ + σ −σ + σ −σ
compared to the inner layer and, hence, the stress in
the outer layer is lower than that in the inner layer. In
atherosclerosis measured at multiple sites in the arterial
case 1, the outer layer is much stiffer than the inner
tree. The Rotterdam Study. Stroke", Ahead of print.
one and the stress in the outer layer is seen to be
KADAR, A., and GLASZ, T., (2001), "Development of
higher than that in the inner layer.
atherosclerosis and plaque biology", Cardiovascular
The presence of an obstacle in the large branch of the
Surgery, 9, 109-121.
carotid bifurcation significantly alters the flow in the
ROSS, R., (1997), "Cellular and molecular studies of
surrounding area. The obstacle is seen to be subjected
atherogenesis", Atherosclerosis, 131, Suppl. S3-S4.
to a force resulted from the difference in the flow
VAN DER WAL, A., BECKER, A., VAN DER LOOS,
pressure before and after the obstacle. This force
et al., (1994), "Site of intimal rupture or erosion of
which is much greater than the normal shear stress is
thrombosed coronary atherosclerotic plaques is
seen to transfer to the inner layer wall and causes
characterized by an inflammatory process irrespective of
much higher contact stress and larger sliding between
the dominant plaque morphology", Circulation, 89, 36-44.
the layers compared to the case without an obstacle.
LIBBY, P., (2003), "Vascular biology of
The contact stress and sliding distance are bigger in
atherosclerosis: overview and state of the art", Am J
case 1 when the stiffness of the inner layer is an order
Cardiol. 6, 3A-6A.
of magnitude smaller than that of the outer layer.
KU, D., ZARINS, C., GIDDENS, D., et al., (1985),
"Pulsatile flow and atherosclerosis in the human carotid bifurcation: positive correlation between plaque
CONCLUSION
localisation and low and oscillating shear stress",
In this work a combined study of the flow inside and
Atherosclerosis, 5, 293-302.
structural behaviour of a carotid bifurcation with an
ZARINS, C., GIDDENS, D., BJARADAJ, B., et al.,
obstacle initiated in its large branch was conducted. This
(1983), "Carotid bifurcation atherosclerosis. Quantitative
combined CFD and a finite element stress/strain study
correlation of plaque localisation with flow velocity
was carryout out with use of a realistically-scaled three-
profiles and wall shear stress", Circulation Research, 53,
dimensional model of carotid bifurcations and ‘one-way'
coupling of the fluid and structure analyses. A two layer
STARY, H., BLAKENHORN, D., CHANDLER, A., et
arterial wall model was devised to represent the interface
al., (1992), "A definition of the intima of human arteries
between the adventitia and media and then exposed to
and of its atherosclerosis-prone regions", Circulation, 85,
finite element stress/strain analysis.
This model revealed significant pressure difference in the
FALK, E., (1992), "Why do plaques rupture?",
flow before and after the obstacle which in turn subjected
Circulation, 86, Suppl. III30-III42.
the obstacle to a force much larger than the normal shear
STEINMAN, D., VORP, D., and ETHIER, C., (2003),
stress. This force is seen to cause increased stress in and
"Computational modeling of arterial biomechanics:
between the arterial wall layers. In addition, the presence
Insights into pathogenesis and treatment of vascular
of an obstacle which narrowed the artery could also
disease", J Vasc Surg, 37, 1118-1128.
elevate the flow speed and the wall shear stress in the
VORP, D., STEINMAN, D., and ETHIER, C., (2001),
vicinity of the obstacle. Therefore, an obstacle which is
"Computational modelling of arterial biomechanics",
initiated by a damage in the blood vessel can cause
Computing in Science and Engineering, Sept/October,51-
increases of the mechanical load on the arterial wall
around the obstacle which may lead to further damage of
HOZAPFEL, G., GASSER, T., and STADLER, M. A.,
the wall (and consequent further growth of the obstacle)
(2002), "Structural model for the viscoelastic behavior of
both by its direct effect on wall structure and also by
arterial walls, Continuum formulation and finite element
producing shearing forces between vessel wall layers. The
analysis", Eur J Mechanics a-solids, 21, 441-463.
stress/strain may also exceed the vessel threshold leading
XIE, J., ZHOU, J., FUNG, Y., (1995), "Bending of
to vessel fracture, which may result in endothelial
blood vessel wall: stress-strain laws of the intima-media
disruption or plaque rupture. Alternatively or in addition,
and adventitial layers", J Biomech Eng, 117, 136-145.
shearing between adventitia and media may tear the vasa
ANGOURAS, D., SOKOLIS, D., DOSIOS, T., et al.,
vasorum producing haemorrhage into a plaque which
(2000), "Effect of impaired vasa vasorum flow on the
may produce embolisation and/or local thrombosis all of
structure and mechanics of the thoracic aorta: implications
which may lead to an acute event.
for the pathogenesis of aortic dissection", Eur J Card Thor
Surg, 17, 468-73.
Success of this model allowed us to expand its application
GLAGOV, S., BASSIOUNY, H., GIDDENS, D., et al.,
to try to more accurately represent the in vivo arterial
(1995), "Pathobiology of plaque modelling and
complication", Surg. Clin. North America, 75, 545-556.
POLDERMANS, D., BOERSMA, E., BAX, J., et al.,
REFERENCES
(1999), "The effect of bisoprolol on perioperative
ROSS, R., (1993), "The pathogenesis of atherosclerosis:
mortality and myocardial infarction in high risk patients
a perspective for the 1990s", Nature, 362, 801-809.
undergoing vascular surgery", N. Engl. J. Med., 341,
VAN DER MEER, I., IGLESIAS DEL SOL, A., HAK,
A., et al., (2003), "Risk factors for progression of
MANGANO, D., LAYUG, E., WALLACE, A., et al.,
(1996), "Effect of atenolol on mortality and cardiovascular morbidity after non cardiac surgery", N.
Engl. J. Med., 335, 1713-1720.
2 Vasa vasorum arise from the adventitia to supply the media and
HOOGWERF, B., and YOUNG, J., (2000), "The HOPE
study. Ramipril lowered cardiovascular risk, but vitamin E
did not", Cleve Clin J Med., 67, 287-293.
MOHAN, D., MELVIN, J., (1982), "Failure properties
of passive human aortic tissue, I-uniaxial tension tests", J.
Biomech., 15, 887-902.
MONSON, K.L., (2001), "Mechanical and failure
properties of human cerebral blood vessels", PhD thesis, UC Berkeley.
BENHAM, P.P., CRAWFORD, R. J., ARMSTRONG
C.G., (1996), "Mechanics of Engineering Materials", 2nd Edition, Prentice Hall.
DELFINO, A., STERGIOPULOS, N., MOORE, J.E.,
and MEISTER, J.J., (1997), "Residual Strain Effects On
the Stress Field In a Thick Wall Finite Element Model Of
the Human Carotid Bifurcation", J. Biomech., 30, pp.777-
768.
FINET, G., RIOUFOL, G., and OHAYON, J., (2002),
"Computational Structural Analysis Based On In Vivo Intravascular Ultrasound Imaging", Proceedings of 2002 Symposium on Endocoronary Biomechanics and Restenosis, Marseille, France.
OHAYON, J., FINET, G., TREYVE, F., RIOUFOL, G.,
and DUBREUIL, O., (2005), "A Three-Dimensional Finite Element Analysis of Stress Distribution in A Coronary Atherosclerotic Plaque: In-Vivo Prediction of Plaque Rupture Location", Book chapter in Biomechanics Applied to Computer Assisted Surgery.
STANLEY, B.M., BUI, A., LIFFMAN, K., SEMMENS,
J.B., and LAWRENCE-BROWN, M.M., (2002), "Shear stress and fatigue fractures in the arterial wall cause intramural micro-hemorrhages that initiate and propagate atheroma: a stress/strain finite element study of the carotid bifurcation", in print.
Source: http://www.cfd.com.au/cfd_conf06/PDFs/049Lif.pdf
ERT Conditions for Productive Learning in Networked Learning Environments: Leadership Report Main author: Lone Dirckinck-Holmfeld (UAalborg) Nature of the milestone: Report Dissemination level: Public Planned delivery date: November 2007 No part of this document may be distributed outside the consortium / EC without
Agentschap voor Buitenlandse Handel Flanders Investment and Trade Montoyerstraat 3 Gaucheretstraat 90 Tél. + 32 2 504 87 11 Verantwoordelijk uitgever : Marc Bogaerts Auteur : François Bries Grafische vormgeving en uitvoering: Image Plus - communicatie corporate Agence Wallonne à l'Exportation Louizalaan 500, bus 4 et aux Investissements Etrangers Saincteletteplein 2