Pii: s0967-0637(00)00042-x
Deep-Sea Research I 48 (2001) 405}437
Physical-biological coupling in the Algerian Basin
(SW Mediterranean): In#uence of mesoscale instabilities
on the biomass and production of phytoplankton
and bacterioplankton
XoseH Anxelu G. MoraHn
*, Isabelle Taupier-Letage,
Evaristo VaHzquez-DommHnguez , SimoHn Ruiz, Laura Arin ,
Patrick Raimbault, Marta Estrada
Dept. Biologia Marina i Oceanograx
a, Institut de Cie%
ncies del Mar, CSIC, Pg. Joan de Borbo&
, s/
n, E-08039 Barcelona, Spain
LOB CNRS, Antenne de Toulon, BP 330, F-83507 La Seyne, France
Dept. Geologia Marina i Oceanograx
a Fn&
sica, Institut de Cie%
ncies del Mar, CSIC, Pg. Joan de Borbo&
,
s/
n, E-08039 Barcelona, Spain
Laboratoire d'
Oce&
anographie et de Bioge&
ochimie, UMR 6535, Centre d'
Oce&
anologie de Marseille, Campus de Luminy,
F-13288 Marseille Ce&
dex 09, France
Received 28 July 1999; received in revised form 1 February 2000; accepted 30 March 2000
The biomass and production of phytoplankton and bacterioplankton was investigated in relation to the
mesoscale structures found in the Algerian Current during the ALGERS'96 cruise (October 1996). Biologicaldeterminations were carried out in three transects between 03 and 23E aimed at crossing a so-called
event,formed by a coastal anticyclonic eddy associated with an o!shore cyclonic eddy to the west. The concentra-tion of chlorophyll
a (Chl) was maximum ('1.2 mg m ) within the cyclonic eddy and at the frontal zonesbetween the Modi"ed Atlantic Water (MAW) of the Algerian Current and the Mediterranean waters furthernorth. Chl (total and '2 lm) was signi"cantly correlated with proxies of nutrient #ux into the upper layers.
Autotrophic picoplankton and heterotrophic bacterial abundance and production presented clear di!er-ences between MAW and Mediterranean water, with higher values at those stations under the in#uence ofthe Algerian Current. In general, greater di!erences were observed in production than in biomass variables.
The photosynthetic parameters (derived from
P}
E relationships) and integrated primary production (range189}645 mg m d ) responded greatly to the di!erent hydrological conditions. The mesoscale phenomenainducing fertilization caused a 2 to 3-fold increase in primary production rates. The relatively high values
* Corresponding author. Tel.: #34-93-2216416; fax: #34-93-2217340.
E-mail address: [email protected] (X.A.G. MoraHn).
0967-0637/01/$ - see front matter 2000 Elsevier Science Ltd. All rights reserved.
PII: S 0 9 6 7 - 0 6 3 7 ( 0 0 ) 0 0 0 4 2 - X
X.A.G. Mora&
n et al. /
Deep-Sea Research I 48 (2001) 405}
437
found within the cyclonic eddy suggest that, although short-lived in comparison with anticyclonic eddies,these eddies may produce episodic increases of biological production not accounted for in previous surveysin the region. 2000 Elsevier Science Ltd. All rights reserved.
Keywords: Phytoplankton; Bacteria;
P}
E relationships; Primary production; Bacterial production; Mesoscale; Mediter-ranean; Algerian basin
The Algerian Current (Millot, 1985; Arnone et al., 1990) is formed by the jet of Modi"ed Atlantic
Water (MAW) that runs along the African continental slope after leaving the Alboran Sea (Heburnand La Violette, 1990) along the usually well-de"ned AlmermHa-Oran front with the Mediterraneanwaters northeast (TintoreH et al., 1991). In this area, surface MAW typically is (37 salinity, whereasMediterranean waters are characterized by values '37.5 (Lacombe and Richez, 1982). It is nowwell established that the Algerian Current (&50 km wide) is not a steady #ow, but one thatpermanently creates mesocale instabilities like meanders and eddies with the potential to in#uencestrongly the general circulation of the di!erent water masses in the Western Mediterranean Basin(Millot, 1999). As a general scheme, eddies (&50 km in diameter) propagate eastward along theAlgerian coast at a few km d , sometimes for months. A major type of instability, recently called
event, is described by Millot (1994) as a meander of the Algerian Current, of a size about100}150 km at its crest, enclosing a deep anticyclonic eddy, its diameter the size of the meander.
Coastal upwelling cells associated with these anticyclonic eddies have been reported (Millot, 1985;Arnone and La Violette, 1986; Millot et al., 1990). Cyclonic eddies are often observed upstream ofthe meander (Obaton et al., in press), showing mushroom-like signatures in SST images. However,such cyclonic circulations are relatively shallow (few 10s to few 100s of m), and last only for a fewdays to a few weeks (Taupier-Letage and Millot, 1988; Millot et al., 1990), whereas anticycloniceddies are likely to last for months up to one year or more (ELISA project, unpublished results).
In order to take into account its mesoscale variability, measurements in the Algerian Basin
require a high temporal and spatial resolution. This is why observations at mesoscale are notnumerous in this area, and why many questions remain unsolved. Although some de"nite resultshave been obtained on physico-chemical aspects of the Algerian Current instabilities (Font et al.,1998; Salas et al., submitted; see Millot (1999) for a review), very few data concerning their e!ect onthe biomass and production of planktonic organisms have been reported. Based on remotelysensed images, Arnone and La Violette (1986), Arnone et al. (1990) and Millot et al. (1990) predicteda strong physical}biological coupling in the area. Since the works of Lohrenz et al. (1988a, b), whoprovided the "rst direct measurements of primary production in the Algerian Basin, no otherresults on the in#uence of Atlantic and Mediterranean type waters on primary production havebeen published to date. Some results on autotrophic plankton distribution were reported byRaimbault et al. (1988a,b; 1993) and Chepurnova and Gutveyb (1988), but the abundance andproduction of other planktonic groups such as heterotrophic bacteria is virtually unknown, withthe exception of some results of bacterial production recently included in a paper by Conan et al.
(1999).
X.A.G. Mora&
n et al. /
Deep-Sea Research I 48 (2001) 405}
437
The ALGERS'96 cruise, carried out in October 1996, was one of the "rst to speci"cally address
the e!ects of the Algerian eddies on biological variables. During it, a so-called
event (Millot, 1994)was detected by satellite imagery (Fig. 1A), and sampling focused on this structure. It consisted ofa meander of MAW enclosing an anticyclonic eddy of &100 km diameter and a cycloniccirculation associated on its western side. The structure was crossed in a series of transectsperpendicular to the Algerian coast (Font et al., 1998). One of the aims was to determine its e!ectson the distribution of physico-chemical and biological properties of the water column, withemphasis on photosynthesis-irradiance relationships as expressed by
P}
E curves (Platt et al., 1980).
Photosynthesis}irradiance relationships are frequently preferred to in situ incubations for estima-ting primary production rates in mesoscale surveys, because incubations can be made on boardwhile the ship is moving and recording continuous data. Although some further acclimation mayoccur during the incubation (Sakshaug et al., 1997), the photosynthetic parameters derived from
P}
E relationships indicate the photoacclimational status of phytoplankton assemblages, and henceprovide a better tool for studying the e!ects of hydrodynamic phenomena than the global valuesobtained from traditional in situ or simulated in situ measurements (Co(teH and Platt, 1984). In thisregard, one should expect the
P}
E relationships to show di!erences re#ecting the upwelling ordownwelling e!ects produced by di!erent types of eddies.
Here, we report the "rst data on phytoplankton and bacterioplankton biomass and production
for mesoscale eddies of the Algerian Basin. Selected stations within three transects perpendicular tothe coast crossing the Algerian Current were sampled for estimating primary production, auto-trophic and heterotrophic picoplankton abundance and bacterial heterotrophic production.
A more detailed description of the vertical distribution of phytoplankton biomass * measured astotal and picoplanktonic ((2 lm) chlorophyll
a * was related to some proxies of nutrient inputto the euphotic layer of the water column. The results provide insight into the structure andfunctioning of microbial food webs in relation to mesoscale instabilities of the Algerian Current.
2. Material and methods
The ALGERS'96 cruise, on board the R/V
Hespe&
rides, took place between 15 and 21 October
1996. Five transects perpendicular to the Algerian coast (with CTD and/or XBT plus ADCPmeasurements) were made, together with an east}west section of expendable CTDs (XCTD), aimedat crossing the two mesoscale eddies identi"ed from satellite images at the beginning of the cruise(Fig. 1A). Biological determinations were made in three of the transects, which will be hereafterreferred to, from west to east, as AC (Algerian Current), CE (Cyclonic eddy) and AE (Anticycloniceddy). In each transect stations were given consecutive numbers starting at the coastal end(Fig. 1B).
2.1. Optics and hydrography
Photosynthetically active radiation (PAR) was measured with a spherical quantum sensor
(LiCor LI-193SA) at 5}10 m depth intervals at the primary production stations (Table 1), except atst. AE8, immediately after water sampling. After visual inspection of changes in the slope of thelog-transformed PAR values versus depth, each pro"le was considered to consist of one or two
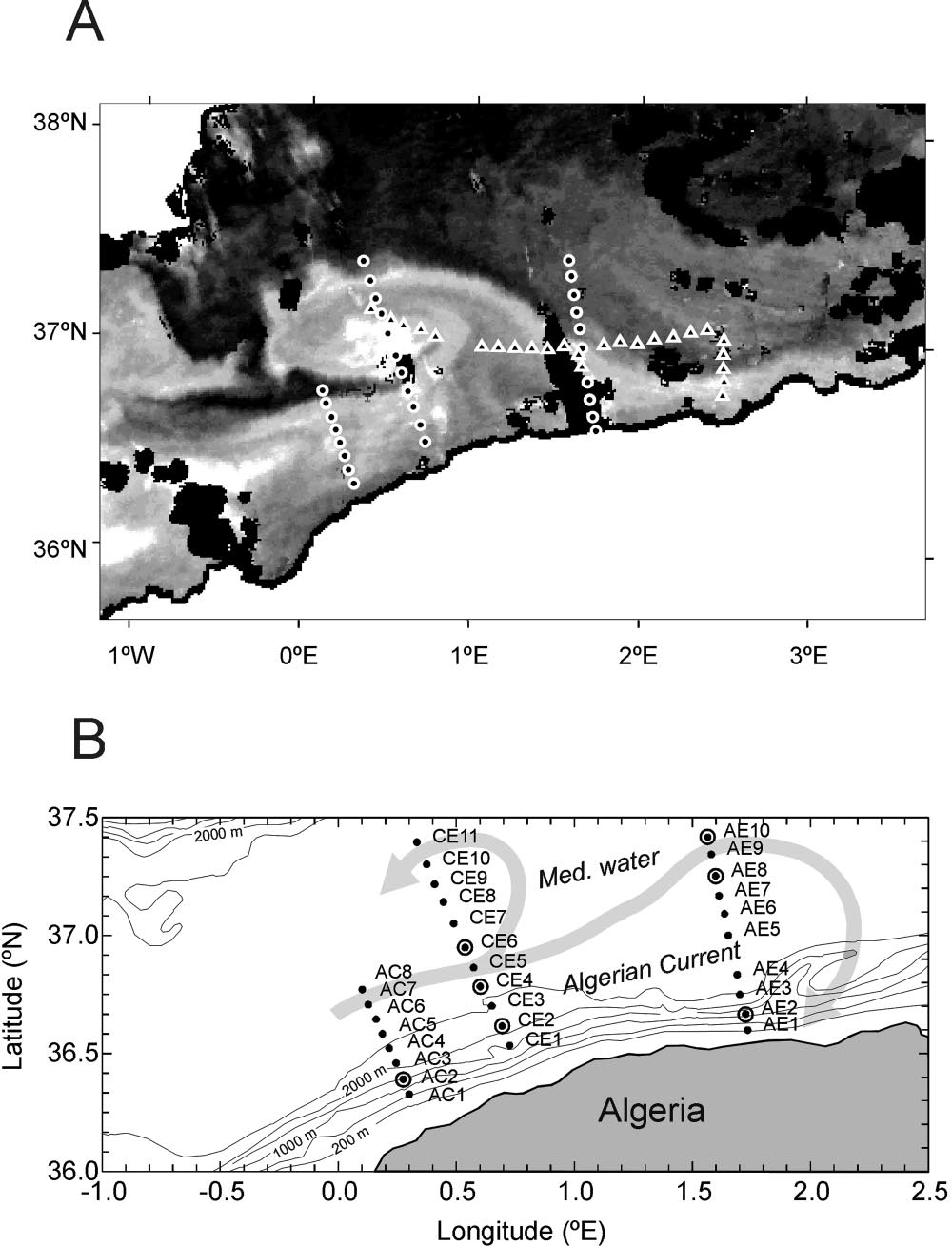
X.A.G. Mora&n et al. / Deep-Sea Research I 48 (2001) 405}437
Fig. 1. (A) NOAA AVHRR image (16-10-96) showing the cyclonic (west) and anticyclonic (east, partially covered withclouds) eddies and the stations sampled. Circles, CTD casts; triangles, XCTDs. (B) Map showing the stations of the threebiological transects: AC, Algerian Current; CE, cyclonic eddy; AE, anticyclonic eddy. The stations where primaryproduction or bacterial biomass and production were determined are surrounded with circles. The grey line shows theapproximate limits of the front between the Modifed Atlantic Water of the Algerian Current and the Mediterraneanwater, and the position of both eddies when they were sampled.
X.A.G. Mora&n et al. / Deep-Sea Research I 48 (2001) 405}437
Table 1Stations where biological determinations other than chlorophyll a were performed
Z NO Z PO
P}E, BHP, BN, PN
P-E, BHP, BN, PN
P}E, BHP, BN, PN
P}E, BHP, BN, PN
P}E, photosynthesis}irradiance relationships; BHP, bacterial heterotrophic production; BN: heterotrophic bacterial
abundance; PN: autotrophic picoplankton abundance. Sampling depths are given for the P}E experiments. Also shownare the depths of the upper mixed layer (Z), the euphotic zone (Z 1%E) and the nutricline for nitrate (Z NO,
'1 lmol l ) and phosphate (Z PO, '0.1 lmol l ). Details are explained in the text. *: not determined.
The same PAR pro"le of station CE4.
vertical layers for which vertical light attenuation coe$cients (K) were calculated. These K's wereused for the calculation of the depth of the 1% light level (Z 1% E). Total incident irradiance was
continuously measured on deck with a cosine corrected sensor LI-190SZ connected to a LiCorLI-1000 Data Logger.
A Neil Brown Mark III CTD was used simultaneously to obtain pro"les of temperature, salinity
and density. The depth at which the change of density (p) was *0.05 kg m over a 5 m depth
interval was considered as the lower limit of the upper mixed layer (Z) after Mitchell and
Holm-Hansen (1991). Visual inspections of the CTD data plots were used when this criterion wasnot clear. Water samples were collected at 10}12 discrete depths with 12 l Niskin bottles froma rosette, for nutrient and biological determinations.
The data set analyzed to obtain the horizontal distributions of diverse physico-chemical
variables included 31 CTD and 25 XCTD casts. 10 m depth averaged temperature and salinitypro"les were used to compute the rest of the variables: density, dynamic height, geostrophicvelocity and relative vorticity. The last parameter was used to identify convergence and divergenceareas. In order to obtain a diagnosis of sampled and derived variables, a technique of objectiveanalysis based on successive corrections (Pedder, 1993) was used. This technique includes "lteringof structures not well resolved by the sampling distribution. Brie#y, this method estimates values atpoints in a de"ned grid (18 km;8 km in this case) and at stations after n iterations. The weightgiven to an observation is dependent on the distance between the observation and the gridpoint.
The estimated value at a gridpoint (
KE) and at a station (
KG) can be expressed as:
E "
KL
KL
G "
KL
KL
X.A.G. Mora&n et al. / Deep-Sea Research I 48 (2001) 405}437
where wEG, wGH is the weighting function, which was chosen as a Gaussian function with a correla-
tion scale (25 km) computed from observational data. A cuto! length scale of 90 km (approx.
double the average distance between stations) was used to eliminate unresolved structures.
2.2. Chlorophyll a and nutrients
Chlorophyll a (Chl) concentration was estimated #uorometrically after extraction of pigment in
methanol as described in Herbland et al. (1985) and Raimbault et al. (1988a). At each station anddepth (typically at 10 m intervals), 250 ml samples were "ltered through Whatman GF/F glass "ber
"lters (25 mm) under vacuum pressures )100 mm Hg. The "lters were placed in a glass tube with
5 ml of pure methanol and kept in the dark at 43C for 30 min. The #uorescence of the extract wasmeasured with a Turner Designs model 10-005 R #uorometer. At 14 stations of transects CE andAE (see Table 2), picoplanktonic Chl (i. e. smaller than 2 lm) was also measured following the sameprotocol as for the total, but prior to "ltering through Whatman GF/F "lters, 250 ml samples were
"ltered through 2 lm Nuclepore polycarbonate "lters (47 mm). Higher percentages of Chl '2 lmwere expected as a consequence of possible fertilization events in the area.
Nutrient samples (NO, NO and PO) were collected into polyethylene #asks and immediately
poisoned with mercury chloride (20 lg HgCl ml ) according to Kirkwood (1992). Samples were
stored in the dark at 43C until analysis back in laboratory, within 1 month from the end of thecruise. Nutrient concentrations were determined by automated colorimetry on a multichannelTechnicon autoanalyzer (TreHguer and Le Corre, 1975). The depths of the nutriclines for NO
(Z NO) and PO (Z PO) were de"ned as the shallowest depths at which concentrations were
higher than 1 lM and 0.1 lM, respectively.
For a more detailed look at the physical-biological coupling within the deep chlorophyll
maximum, averaged concentrations of Chl and nutrients were calculated for the 20}60 depth range(Chl}, NO }, NO }, PO }), as this range roughly corresponded to the layer of
maximum Chl (see Results).
2.3. Autotrophic and heterotrophic picoplankton abundance
Samples for enumeration of cyanobacteria (Synechococcus), prochlorophytes (Prochlorococcus)
and small eukaryotes were taken at the stations shown in Table 1. At each station, 10 depths weresampled from 5 to 90 m. 2 ml water samples were immediately "xed with paraformaldehyde (1%
"nal concentration) and stored frozen with liquid N until analysis. To obtain the cyanobacteria
and prochlorophytes abundance, subsamples of 200 ll were counted within 3 months after the endof the cruise with a FACScalibur (Becton and Dickinson) #ow cytometer, equipped with a laseremitting at 488 nm. Fluorescent 1 lm Polysciences latex beads were added as an internal standard.
Bead standard concentration was determined with epi#uorescence microscopy. Cyanobacteria andprochlorophytes #uorescence could be easily identi"ed in plots of side scatter (SSC) versus FL2(orange #uorescence, 580 nm) or FL3 (red #uorescence, 640 nm) respectively. 10000 events wereacquired at low #ow rate (&12 ll min ) for every sample.
Heterotrophic bacterial abundance and production were measured at the same stations and
depths as for autotrophic picoplankton. 30 ml samples were collected and preserved with glutaral-dehyde (1% "nal concentration). Within the same day of collection, 10 ml subsamples were stained
X.A.G. Mora&n et al. / Deep-Sea Research I 48 (2001) 405}437
with DAPI at 5 lg ml "nal concentration (Sieracki et al., 1985) following the technique ofPorter and Feig (1980). Stained samples were "ltered through 0.2 lm black polycarbonate
"lters, which were mounted on microscopic slides and
stored frozen (!203C) until analysis.
Abundance of heterotrophic bacteria (BN) was measured at 1250; with a NikonLabophot 2 microscope under UV excitation. At least 200 cells were counted in 20 random "elds(Kirchman et al., 1992). For the measurement of biovolume, 10 images of each sample werecaptured with an image analysis system described by Massana et al. (1997), and a minimumof 100 heterotrophic bacteria per sample were sized. Bacterial biomass was calculated with thecarbon to volume relationships derived by Norland (1993) from the data of Simon and Azam(1989):
pg C cell "0.12;(lm cell )
Bacterial biomass (BB, mgC m ) was calculated as the product of BN and bacterial carboncontent.
2.4. Bacterial heterotrophic production
Bacterial heterotrophic production (BHP) was estimated by the method of H-leucine incorpo-
ration as described in Smith and Azam (1992). Commercial leucine solution (Amersham) wasbrought to 1 lM with 0.2 lm "ltered and autoclaved MilliQ water and mixed with non-radioactiveleucine (10% hot : 90% cold). Next, 40 nM leucine was added to 1.5 ml seawater sample inEppendorf vials; this concentration was chosen because it had been shown to be saturating forMediterranean waters (PedroHs-AlioH et al., 1999). Three replicates and an immediately killed controlwere incubated in the dark at temperatures as close as possible to those of seawater. Incubationslasted approx. 3 h in accordance with previous results of linearity experiments (Gasol, pers. comm).
All samples were killed with 50% TCA (5% TCA, "nal concentration) and stored at ambienttemperature until processing according to Smith and Azam (1992). 0.5 ml of scintillation cocktailwere added to the vials and counted on board in a Beckman LS6000 (LL) liquid scintillationcounter. Disintegrations per minute (dpm) were calculated with the external standard method (Hnumber). Finally, BHP was calculated by multiplying leucine incorporation rates by a conversionfactor (CF) of 0.94 kgC mol , which was the average of empirically determined CF's for twoo!shore stations of the NW Mediterranean (PedroHs-AlioH et al., 1999). Bacterial daily speci"cgrowth rates (k) were calculated as:
2.5. P}E relationships
Photosynthesis}irradiance experiments were carried out at six selected stations (Fig. 1B). Table
1 shows the time and depth of sampling. At each experiment, water was taken from the surface(5 m) and the deep chlorophyll maximum (DCM), except at stations 77 and 85, which did not showa clear DCM. At these stations, deep samples * which will also be referred to as DCM samples forconvenience * were taken from 40 and 20 m, respectively. 70 ml water aliquots were transferredinto sterile polystyrene tissue culture bottles (Corning). For each experiment and depth, fourteen
X.A.G. Mora&n et al. / Deep-Sea Research I 48 (2001) 405}437
light plus one dark (wrapped in aluminium foil) bottles were incubated in closed linear incubatorswith circulating water at the in situ sampling temperature.
Before starting the experiments, all bottles were spiked with 3.54 ;10}3.65;10 Bq
(9.6}9.9 lCi) of C-bicarbonate (VKI, Denmark). Illumination for the surface samples wasprovided
4}2000 lmol m s . A slide projector, which gave a range of 0.9}280 lmol m s , was usedfor the DCM samples. Irradiance (PAR) was measured prior to the experiments with a smallspherical quantum sensor (Illuminova AB, Sweden) inside each bottle. After 2}3 h incubation,water was "ltered ((100 mm Hg) through glass "ber Whatman GF/F "lters of 25 mm indiameter. The measurement of labelled organic carbon retained on glass "ber "lters gives anestimate of primary production that may lie closer to total than to the particulate fraction (MoraHnet al., 1999), because of adsorption of photosynthate released as dissolved organic carbon. Filterswere exposed to fuming HCl (35%) for ca. 12 h and left to dry prior to being placed into vials. 4.5 mlof Beckman Ready Safe liquid scintillation cocktail were added to the vials. Radioactivity retainedon "lters was measured in a Beckman LS6000 (LL) liquid scintillation counter and dpm's werecalculated after quenching correction with the external standard method. Before further calcu-lations, black bottle values were subtracted from the light bottle values for correction of non-photosynthetic C "xation.
Surface and deep samples were clearly di!erent with respect to photoinhibition: none of the
surface samples showed it, whereas all but one of the deep samples did; therefore di!erent versionsof the model describing the P}E relationship were used. Fitting was by non-linear least-squaresregression. Surface data were "tted by the model of Webb et al. (1974):
P "P [1!exp(!aE/P )]
where P (mg C mg Chl h ) is the chlorophyll a-normalized photosynthetic rate, P (mg C mg
a [mg C mg Chl h (lmol photons m s ) ] is the initial slope of the P}E curve.
DCM data were "tted by the model of Platt et al. (1980):
P "P [1!exp(!aE/P )][exp(!bE/P )]
where P (mg C mg Chl h ) is the maximum chlorophyll a-normalized photosynthetic rate
without photoinhibition and b (same units as a) is the photoinhibition parameter. The rest of theparameters are like those in the previous model. If b becomes 0 (i.e. no photoinhibition), P in the
Platt et al. model would then be equivalent to P in the model proposed by Webb et al.
The relationship of the photosynthetic parameters P , a and b with physical variables and
nutrient concentration was explored by a matrix of correlations.
2.6. Integrated phytoplankton and bacterial biomass and production
Integrated values of biomass (in carbon units) for the phytoplankton and heterotrophic bacteria
were calculated for the 0}100 depth range. Phytoplankton carbon content was estimated byconverting mg Chl to mg C with a C : Chl ratio of 40 (Li et al., 1993) for the whole depth range,although this assumption should be taken with caution, as the C : Chl ratio usually decreases withdepth (e.g. Taylor et al., 1997).
X.A.G. Mora&n et al. / Deep-Sea Research I 48 (2001) 405}437
The P}E parameters, together with the depth pro"les of PAR and chlorophyll a, and the daily
irradiance were used to estimate the daily rates of primary production (PP ); integration down to
100 m depth was performed by the trapezoidal method. The same method was adopted forintegrated bacterial heterotrophic production (BHP ). For the estimation of PP at st AE8,
where no PAR depth pro"le was available, that of station CE4 was used as both stations presentedvery similar Chl vertical pro"les. A linear regression between the surface and deep P}E values ateach station was used for obtaining an estimate of their variation through the corresponding depthrange. The deep P}E values were kept for the rest of the water column. For comparison, PP was
also calculated in the usual way, i.e. with a cuto! between two constant (surface and deep)situations, made at the Z or by visually inspecting the p and Chl pro"les. Finally, an estimate of
the gross #ux of organic carbon through heterotrophic bacteria (g-BHP ) was calculated by using
a bacterial growth e$ciency of 0.20.
3.1. Optics and hydrography
Daily irradiance conditions at the investigated stations were rather similar. Mean ($SD) value
for total incident irradiance was 25.4$3.7 mol m d during the cruise. Vertical light extinctioncoe$cients (K) varied little (0.047$0.012 m ), hence rendering very similar compensation depths(Z 1% E), around 95 m (Table 1).
Horizontal distributions of temperature, salinity and density at 10 and 50 m are shown in Fig. 2.
Dynamic height, geostrophic velocity and geostrophic relative vorticity distributions at the samedepths are shown in Fig. 3. The mesoscale instability of the Algerian Current was developingaround 13E and appeared as a meander of the current surrounding a coastal anticyclonic eddy,associated with a secondary cyclonic eddy upstream of it (Font et al., 1998). A detailed study ofLagrangian buoy trajectories and infrared images (Salas et al., submitted) con"rmed that thesemesoscale phenomena corresponded to a so-called event (Millot, 1994). Maximum geostrophicvelocites (&35 cm s ) were found associated with the anticyclonic circulation. The cyclonicmotion was not as evident as the anticyclonic on the geostrophic velocity "eld (Fig. 3). There aretwo possible reasons: "rst, and possibly more important, coverage of data was poor in the areawhere the cyclonic eddy was developing; and second, the e!ect of the mathematical "lter used onthe analysis could have smoothed the structure. In spite of that, the western part of the area stillshowed signs of cyclonic circulation, clearly seen in the relative vorticities. The cyclonic eddy wase!ectively associated with an area of positive vorticity, whereas the anticyclonic presented negativevalues.
The e!ect of the eastward #owing Algerian Current was easily seen as a downward sloping of
isopycnals towards the coast in the three transects (Fig. 4), mostly due to the lower salinity contentof the MAW (surface salinity (37; Lacombe and Richez, 1982) as compared to the Mediterraneanwaters further north (surface salinity '37). The transect AC shows the characteristic, undisturbedpattern of the Algerian Current (Arnone et al., 1990). Most of the stations in the three transects fellunder the in#uence of the MAW, except the most o!shore stations AC8, CE11 and AE10, locatedat approx. 70, 125 and 100 km from the coast, which could be clearly adscribed to Mediterranean
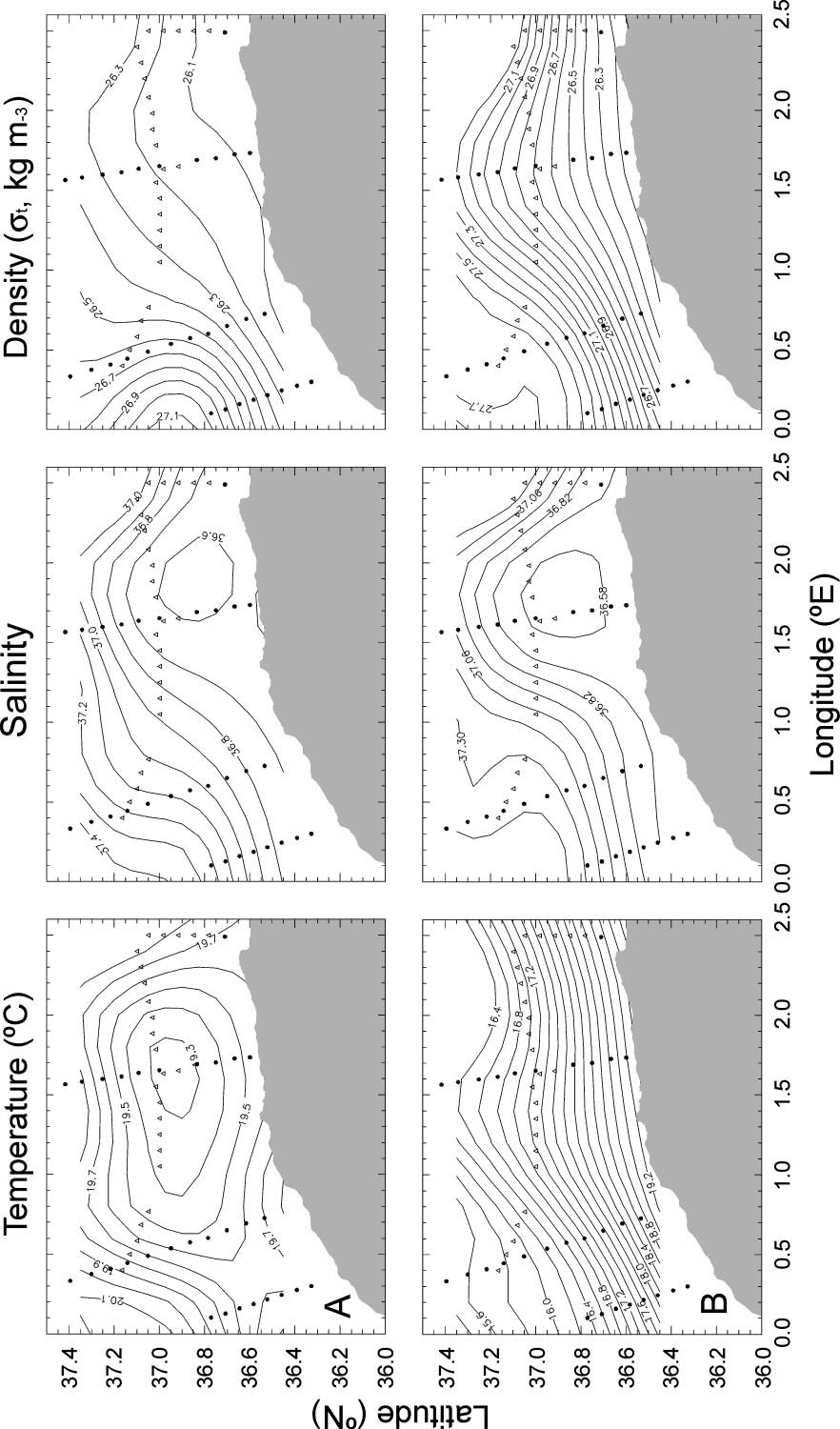
X.A.G. Mora&n et al. / Deep-Sea Research I 48 (2001) 405}437
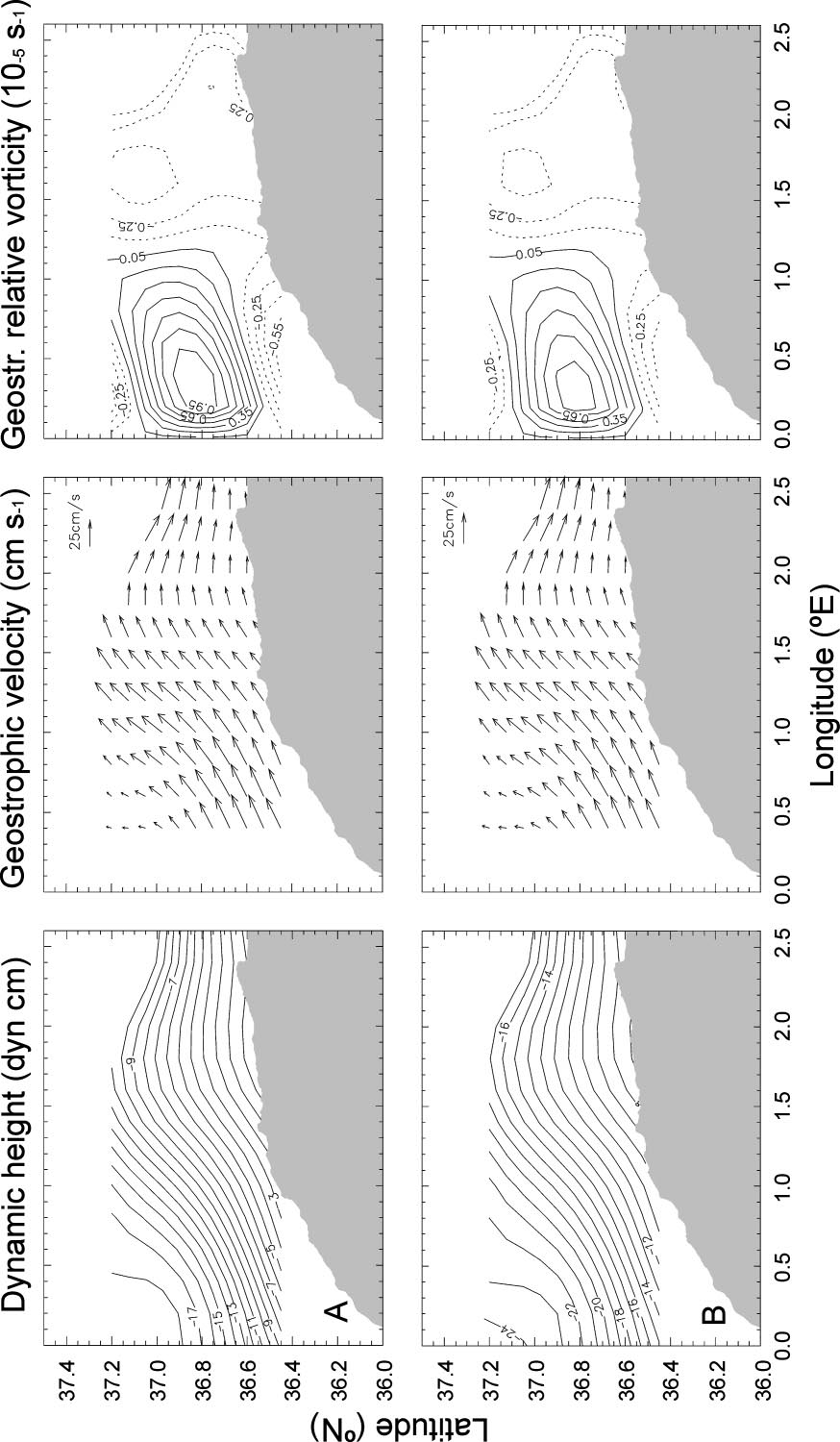
X.A.G. Mora&n et al. / Deep-Sea Research I 48 (2001) 405}437
X.A.G. Mora&n et al. / Deep-Sea Research I 48 (2001) 405}437
waters. In the CE transect, the e!ect of the cyclonic eddy, centered at stations CE6-CE7, wasdetected as a slight doming of the isopycnals (Fig. 4). Roughly, the limits of the cyclonic gyre couldbe considered to be around stations CE3-CE4 and CE9-CE10. The anticyclonic eddy crossed intransect AE produced a relative deepening of the isopycnals, isohalines and isotherms (Fig. 4) and,because of the displacement of the Algerian Current, extended the in#uence of the MAW as far as90 km north of the coastline.
3.2. Chlorophyll a and nutrients
The highest concentrations of total Chl ('1 mg m ) in the three transects were observed
between approximately 20 and 60 m depth (Fig. 5), de"ning a conspicuous deep chlorophyllmaximum (DCM). High values were found at the frontal zone between MAW and Mediterraneanwaters, represented by stations AC7 in transect AC and AE9 in transect AE, where the DCM wasalso shallower, specially in transect AC. The vertical distribution of Chl in transects AC and AEpresented a similar pattern, consisting of an intermediate layer of relatively high concentrationsthat became gradually shallower as it approached the frontal zones, where it also reachedmaximum values. Within the cyclonic eddy, three distinct areas of increased Chl concentrationswere found, coinciding with the central part of the eddy and its northern and southern edges. At thecoastal edge of this eddy, the position of the DCM was very shallow (&25 m) as compared to therest of the stations and showed the maximum concentrations found during the survey('1.4 mg Chl m ). Conversely, the most remarkable e!ect of the anticyclonic eddy was todisplace o!shore the shallowing of the DCM, so that values '0.8 mg m were not found until&70 km away from the coast (Fig. 5).
Integrated values of total Chl down to 100 m depth (Chl ) varied little, with an average of
42 mg Chl m and a range of 28 to 63 mg Chl m . Overall, transect AE showed slightly lowervalues of Chl than AC or CE (Table 2). Chl was on average almost evenly distributed in the two
size-classes considered: ' and (2 lm (Table 2), with a mean percentage of picoplanktonic Chl(Chl (2 lm) of 51%. The highest values of Chl '2 lm for each transect were found at the
stations that also showed higher total values (CE3, AE9). The slope of the log}log regressionbetween Chl and Chl (2 lm (Fig. 6) was signi"cantly lower than 1.0 (p"0.0025), indicating
that the higher the total Chl content in the water column the lower the picoplanktonic contribu-tion.
Nitrate and phosphate distributions in the upper 120 m of the transects are shown in Fig. 5. The
depths of both nutriclines generally followed the same pattern as the density, salinity andtemperature distributions, i.e. became shallower at the frontal zones, and in general they were alsoshallower in transect CE (mean Z NO"38 and Z PO"43) as compared with the other two
transects (Fig. 5), re#ecting the in#uence of the cyclonic eddy. When combined in a single data set,both nutricline depths were signi"cantly and negatively correlated with the percentage ofChl '2 lm (r"!0.57; p"0.042; n"13) and the maximum Chl concentration (Chl"!+) at
each station (Table 3). Used as an upwelling proxy, the depth of the 37 isohaline (Z ) was also
negatively correlated with Chl"!+ (Table 3).
The depth of the DCM was shallower the higher the averaged nitrate and phosphate concentra-
tions for the 20}60 depth range (Table 3). Shallower DCMs were also associated with higher Chlconcentrations at the surface as indicated by the correlation between the two variables (Table 3).
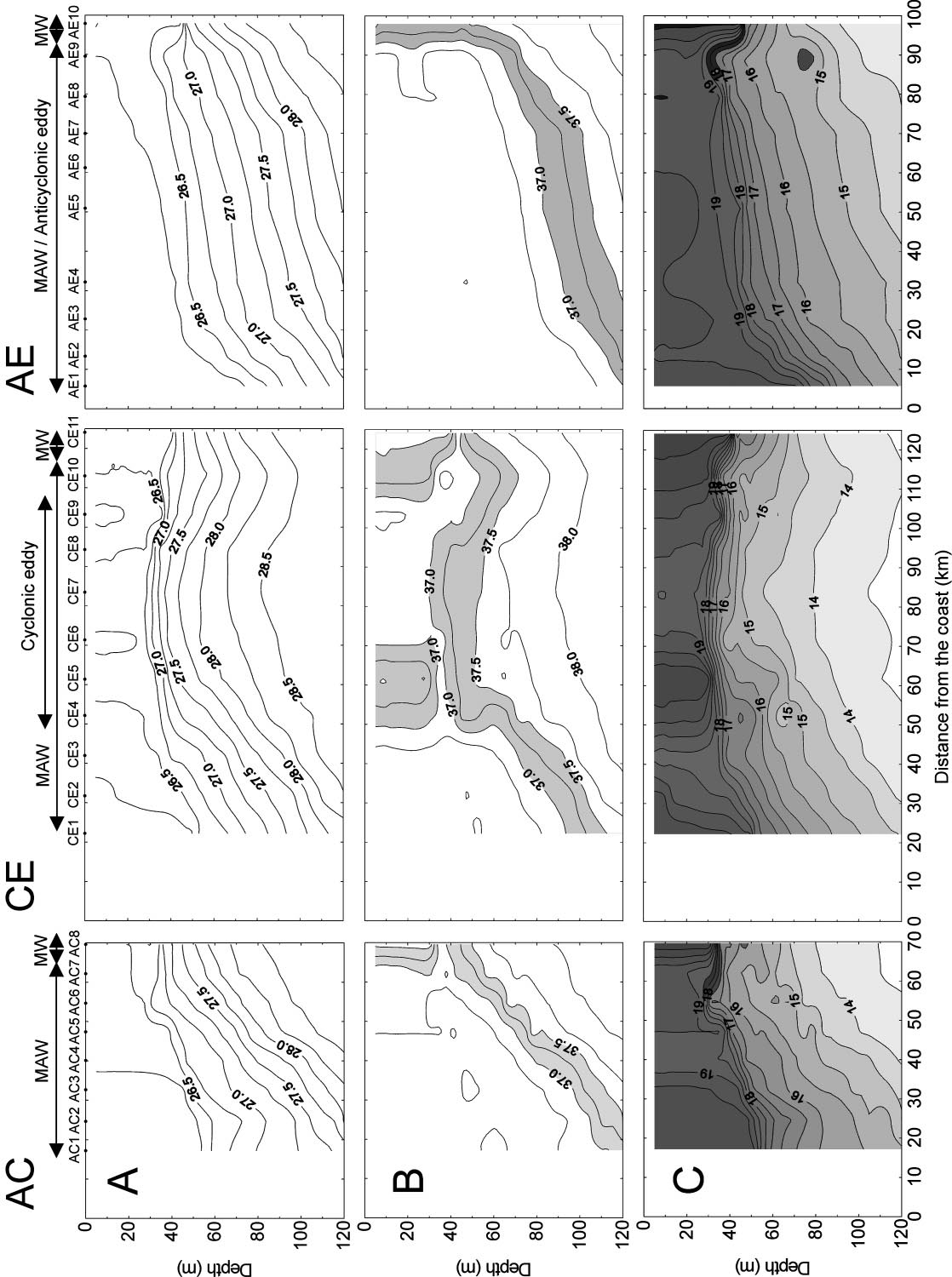
X.A.G. Mora&n et al. / Deep-Sea Research I 48 (2001) 405}437
g,k(p R rature
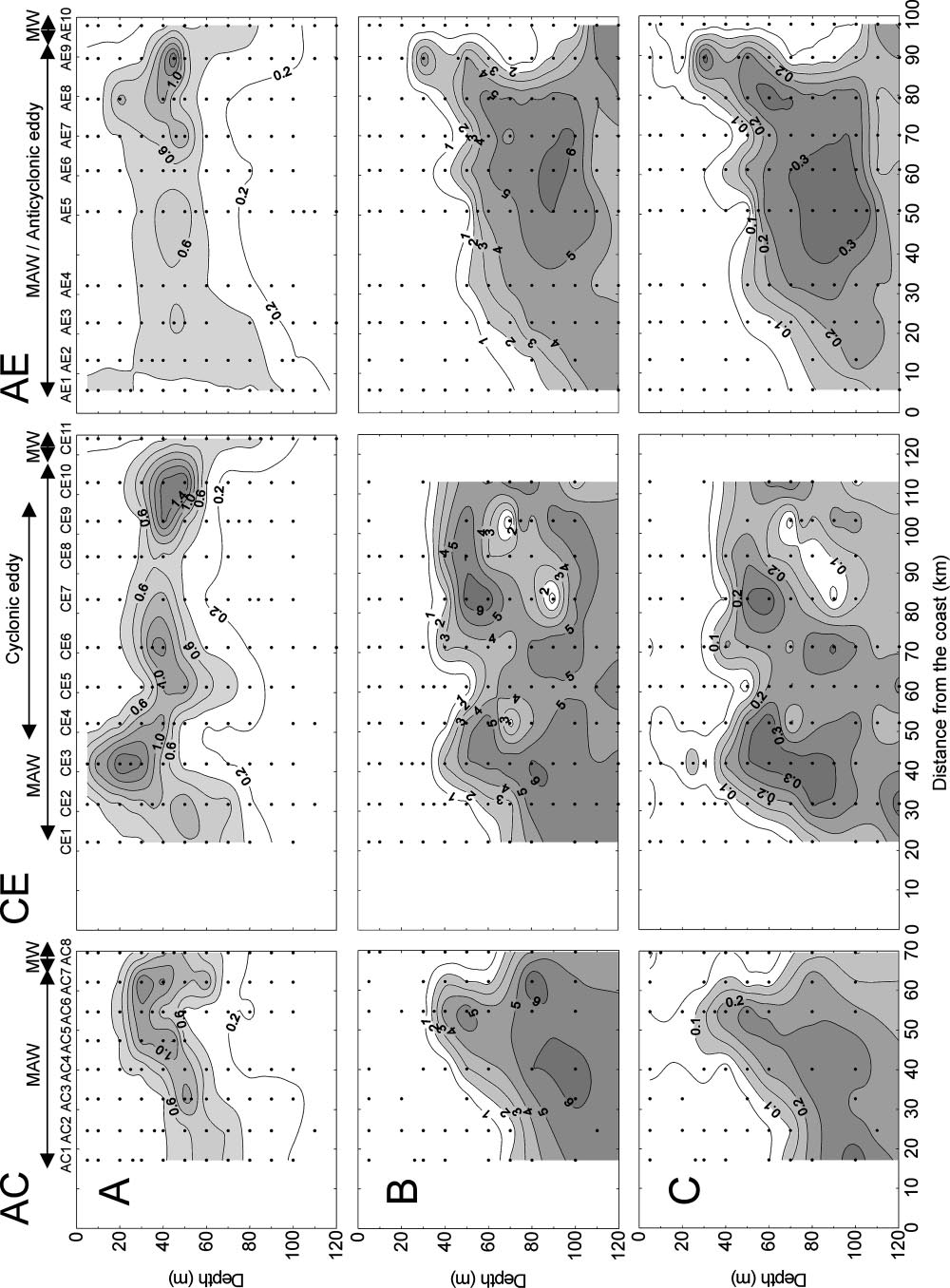
X.A.G. Mora&n et al. / Deep-Sea Research I 48 (2001) 405}437
X.A.G. Mora&n et al. / Deep-Sea Research I 48 (2001) 405}437
Table 2Depth of the DCM (Z"!+), concentration of Chl at the surface (Chl) and integrated values down to 100 m depth of total
Chl (Chl ), Chl smaller than 2 lm (Chl (2 lm) and percent chlorophyll larger than 2 lm (%Chl '2 lm) for all the
Integrated to 60 m, not included in the mean values provided.
X.A.G. Mora&n et al. / Deep-Sea Research I 48 (2001) 405}437
Fig. 6. Relationship between integrated (down to 100 m) values of total Chl (Chl ) and Chl(2 lm (Chl (2 lm)
for all the available stations. Linear regression: log Chl (2 lm"0.42#0.56 log Chl ; R"0.71; p"0.00014;
Chl was only marginally correlated with PO } and NO } concentrations. Higher
correlations were observed between both nutrients and Chl}'2 lm or its percentage
(%Chl '2 lm). The e!ect of nutrient upwelling on the buildup of phytoplankton biomass (as
measured by Chl) in the DCM for the whole area was supported by the fact that the depths of thenutriclines, specially that of phosphate, were negatively correlated with Chl} and
Chl}'2 lm. NO } was correlated only with Chl smaller than 2 lm.
3.3. Autotrophic and heterotrophic picoplankton abundance
Vertical pro"les of the abundance of autotrophic picoplankton (prochlorophytes and cyanobac-
teria) and heterotrophic bacteria (BN) are shown in Fig. 7. Table 4 shows their weightedconcentrations in the 0}90 m depth range of the water column. Maximum numbers were found atstation AC2 for heterotrophic bacteria (9.0;10 cells ml ) and at AE8 for picoeukaryotes andcyanobacteria (2.1;10 and 2.4;10 cells ml , respectively). Station AE10 showed the lowestnumbers of these groups but maximum abundance of prochlorophytes (7.8;10 cells ml ).
Vertical distributions of BN were rather uniform except at stations CE6 and AE8, where theydeclined approx. 2- and 3-fold, respectively, between surface and 90 m depth. The vertical distribu-tions of prochlorophytes and cyanobacteria were comparable at the "ve stations, with a steadydecrease with depth, and similar numbers except at station AE10, where prochlorophytes weremore abundant than cyanobacteria at all depths. Two di!erent populations of Synechococcus-likecells were detected according to their FL2 and FL3 signals in the #ow cytometer, which we calledSynechococcus A (SynA, average FL2(10 and FL3 &1;10, arbitrary units) and SynechococcusB (SynB, average FL2 &3 ;10 and FL3 &8;10). SynA was generally one order of magnitudeless abundant than SynB and appeared only at shallow depths, exceptionally reaching 70 m atstation AC2. The contribution of SynA to total Synechococcus cell numbers was nevertheless low
X.A.G. Mora&n et al. / Deep-Sea Research I 48 (2001) 405}437
l "!+ l l 2
X.A.G. Mora&n et al. / Deep-Sea Research I 48 (2001) 405}437
Fig. 7. Vertical distribution of the abundance of heterotrophic bacteria (䊏) and autotrophic picoplankton: cyanobacteria(䢇) and prochlorophytes (*) at the "ve sampled stations. See the text for details.
(Table 4), and decreased from the coast (7% at station AC2) to o!shore waters (0.1% at stationAE8 and absent at station AE10).
Mean biovolume of heterotrophic bacteria varied little (Table 4), ranging from 0.035 to
0.044 lm. Accordingly, heterotrophic bacterial biomass (BB) varied less than bacterial numbers,and ranged from 8.19 to 11.20 mg C m , with maximum values at station CE6.
3.4. Bacterial heterotrophic production
Weighted values of BHP in the 0}90 m depth range for the stations investigated are shown in
Table 4. They followed a decreasing pattern along the inshore-o!shore gradient (see Fig. 1B) andranged over one order of magnitude, with maximum values at station AC2 (4.50 mg C m d )and minimum at station AE10 (0.32 mg C m d ). Vertical pro"les were rather variable (datanot shown), but in general showed higher values in the upper layers. Corresponding speci"c growthrates (Table 4) gave duplication times ranging from 2 (station AC2) to 17 d (station AE10).
3.5. P}E relationships
The photosynthetic parameters for the six stations where P}E experiments were carried out are
shown in Table 5 and selected P}E curves are shown in Fig. 8. All the parameters showedsigni"cant di!erences between surface and DCM waters: t-tests (n"6) for P (p"0.0009),
a (p"0.0148) and E (p"0.0001). At each station maximum photosynthetic rates (P ) were
always higher in surface waters (average 2.83 mg C mg Chl h ) than at the DCM (average0.74 mg C mg Chl h ). Maximum values of P at surface were found at stations AC2, AE8 and
AE10 ('3 mg C mg Chl h ), whereas maximum values at the DCM were found at stationsCE4 and CE6. The maximum light utilization coe$cient or initial slope of the P}E curve (a) was onaverage 4.8-fold higher at the DCM than at the surface (Table 5).The light saturation parameter
X.A.G. Mora&n et al. / Deep-Sea Research I 48 (2001) 405}437
X.A.G. Mora&n et al. / Deep-Sea Research I 48 (2001) 405}437
Fig. 8. Photosynthesis}irradiance relationships for stations AC2 (coastal, MAW), CE6 (cyclonic eddy, MAW) and AE10(o!shore, Mediterranean water).
(E) was also always higher at the surface and well above 100 lmol photons m s , with
the minimum value found at station CE6, and it was correlated with depth (Table 6), ranging from5 to 55 lmol photons m s . Photoinhibition was observed in all the DCM samples except atstation AE2 (40 m). Station CE6 showed the highest value of b [0.0014 mg C mg Chl h (lmolphotons m s ) ]. The rest of the stations gave values an order of magnitude lower.
As could be expected from the above mentioned results, most parameters were correlated with
depth or a covarying variable such as temperature or p (Table 6). Thus, a was more correlated with
X.A.G. Mora&n et al. / Deep-Sea Research I 48 (2001) 405}437
X.A.G. Mora&n et al. / Deep-Sea Research I 48 (2001) 405}437
Table 6Matrix of correlations between P}E parameters and integrated primary production and diverse physico-chemicalvariables
Z NO Z"!+
Temp, Salt, Dens, NO, PO, NO: in situ values. PP , integrated primary production down to 100 m depth;
PP}, integrated primary production for the 20}60 depth range; Temp, surface (5 m) temperature; Salt, surface
salinity; Dens, surface density. More details are given in the text. n"12 for P , a and b; n"5 for E; n"6 for PP and
temperature, P with depth and E with p. The correlation of b with in situ PO or NO
concentrations probably re#ected their parallel change with depth.
3.6. Integrated phytoplankton and bacterial biomass and production
Biomass and production of algae and heterotrophic bacteria integrated down to 100 m depth are
shown in Table 7. Integrated biomass for the two groups was rather constant, varying by a factor of1.7 and 1.4, respectively. Highest values of phytoplankton biomass (PB ) were found for stations
CE2 (primary production not measured) and CE6, whereas for bacterial biomass (BB ) stations
AC2, CE2 and CE6 yielded similarly high values. Minimum values of both variables were foundo!shore, at station AE10.
In contrast, daily rates of primary production (PP and bacterial heterotrophic production
(BHP ) were very variable. PP ranged from 186 to 636 mg C m d when the linear
interpolation approach was used (PP 1, see Table 7). Di!erences between these values and those
obtained assuming two sets of constant values for the water column were not signi"cant (pairedt-test; p"0.92; n"6). Highest values were found at stations AC2 and CE6, and similarly lowvalues were found at stations AE2 and AE10. There was no signi"cant relationship between PP
and Chl (either integrated or at surface) for the six stations, or with any physical or nutrient variable,except for a close to signi"cant correlation with surface temperature (Table 6). The relationship withsurface temperature became signi"cant when the primary production at the DCM only(i.e., between 20 and 60 m depth) was considered (Table 6, Fig. 9). Primary production at
X.A.G. Mora&n et al. / Deep-Sea Research I 48 (2001) 405}437
X.A.G. Mora&n et al. / Deep-Sea Research I 48 (2001) 405}437
Fig. 9. Relationships between surface temperature and the depth of the PO nutricline ('0.1 lmol l ) and integrated
primary production (PP ) in the 0}100 m and the 20}60 m depth ranges.
the DCM was also marginally correlated with the depth of the nutricline for PO (Fig. 9) and the
depth of the Chl maximum. BHP showed a noticeable 12-fold variation at the "ve stations shown
in Table 7, and ranged from 33 to 384 mg C m d . No signi"cant relationship was foundbetween BHP and the physico-chemical variables shown in Table 6.
Several ratios of phytoplanktonic and bacterial production and biomass are presented in Table
7. On average, BB was 57% of PB and was less variable than the ratio of BHP to PP
(average of 25%). The integrated gross #ux of organic carbon through heterotrophic bacteria(g-BHP ), calculated as BHP /BGE with a bacterial growth e$ciency (BGE) of 0.20, was higher
than PP at station AC2 (by a factor of &3).
X.A.G. Mora&n et al. / Deep-Sea Research I 48 (2001) 405}437
The general hydrological characteristics of a section through the Algerian Current were repre-
sented by transect AC (Fig. 4), which showed a gradual northward loss of the current character-istics due to mixing with Mediterranean waters. Nevertheless, a clear frontal boundary between theModi"ed Atlantic Water (MAW) of the current and the Mediterranean type waters situated to thenorth was identi"ed. Previous work in the region had shown that the frontal zones producednoticeable biological signatures (Raimbault et al., 1993; Lohrenz et al., 1988b; MartmHnez et al., 1990;Peinert and Miquel, 1994; Arnone et al., 1990). In this work, these frontal areas presented higherChl concentrations and at shallower depths than the rest of the stations in their respective transects(Fig. 5), although quite below the '6 mg Chl m reported by Raimbault et al. (1993).
During the ALGERS'96 cruise, the Algerian Current was modi"ed downstream by the presence
of two surface structures: a coastal anticyclonic eddy embedded in a meander of the current and ano!shore cyclonic eddy upstream of it (Font et al., 1998). The presence of this instability had markede!ects on the distribution and abundance of planktonic organisms. The major e!ect of theanticyclonic eddy was a general impoverishment in phytoplankton biomass (Fig. 5, Table 2). Noincreased Chl levels were observed at its coastal end, as no evidence was found of the previouslyreported coastal upwelling cells associated with anticyclonic eddies (Millot, 1985; Arnone and LaViolette, 1986). Conversely, the edges and the center of the cyclonic eddy presented the highest Chlconcentrations in the whole area for the period of study (Fig. 5). The comparatively high Chlconcentrations found at shallow depths around station CE3 were a probable consequence ofnutrient upwelling, associated with the divergence zone caused by relative vorticities of opposedsigns (Fig. 3).
It is well known that, at frontal zones, isopycnal mixing frequently injects nutrients into the
photic layer (e.g. Woods, 1977; Fasham et al., 1985). Diapycnal mixing was also proposed asa mechanism of nutrient injection in the boundary region between the MAW and Mediterraneanwater in the Algerian Current (Raimbault et al., 1993). In any case, in a scenario with well-de"nedmesoscale instabilities inducing localized fertilization events as mentioned above, we expected to
"nd a relationship between phytoplanktonic biomass and proxies of nutrient #ux into the upper
layers of the photic zone, such as the depths of the nutriclines or the averaged DCM (i.e. 20}60 m)nutrient concentrations. Overall, correlations were weak, but became stronger when Chl'2 lmwas considered (Table 3). In the context of an overall low variability of the contribution of big cells('2 lm) to total values of integrated chlorophyll a, the stations with shallower nutriclines (CE3,AE9) showed higher percentages of Chl'2 lm (Table 2), thus probably re#ecting better condi-tions for the in situ growth of phytoplanktonic assemblages. Picoplanktonic ((2 lm) Chl was onaverage approximately half of the total content, slightly lower than the mean percentage given forthe Mediterranean (59%) by Magazzu and Decembrini (1995). A decreasing contribution ofpicoplanktonic Chl with increasing total values (Chisholm, 1992) was identi"ed (Fig. 6), as inprevious reports for the same (Raimbault et al. (1988a, b) or other areas of the Mediterranean (seereview of Magazzu and Decembrini, 1995).
The slightly but consistently higher correlation coe$cients of diverse Chl variables with PO
than with NO concentrations (Table 3) could suggest that P was the limiting nutrient, as
evidenced in other studies carried out in the Mediterranean (e. g. Krom et al., 1991; Berdalet et al.,1996; Zohary and Robarts, 1998). N : P ratios considerably higher than 16 : 1 have been commonly
X.A.G. Mora&n et al. / Deep-Sea Research I 48 (2001) 405}437
viewed as indicators of P limitation in the sea. The signi"cant correlation between pooled data ofChl} and mean N : P ratio for the same depth range in transects AC and AE (r"0.63;
p"0.009; n"16) would suggest that the buildup of high Chl levels would exhaust availableP faster than N. Raimbault and Coste (1990) argued that preferential uptake of phosphate bynutrient-starved phytoplankton was the cause for the very high N : P ratios found in the DCM inthe NW Mediterranean. Furthermore, shallower depths of the nutriclines (related in turn to higherconcentrations of Chl, Table 3) were strongly associated with increasing N : P ratios (r values of
!0.72 and !0.82; p(0.0005; n"26). The lack of a signi"cant relationship between Chl}
and mean N : P ratio in the CE transect could be related to the heterogeneity in the nutrientdistribution imposed by the evolving cyclonic eddy over the general pattern of the AlgerianCurrent. Phytoplankton has been seen as a major contributor to NO concentrations in the photic
layer via nitrate reduction (Blasco, 1971; Dore and Karl, 1996). In this case, DCM nitriteconcentrations (NO }) correlated only with picoplanktonic Chl, not with total Chl content.
The composition of autotrophic and heterotrophic picoplankton revealed di!erences between
Modi"ed Atlantic Water and Mediterranean waters. Except for the prochlorophytes, which weremore abundant at station AE10, all the groups analyzed (heterotrophic bacteria, cyanobacteria andpicoeukaryotes) were more abundant inside the Algerian Current than at this station, representa-tive of Mediterranean water (Table 4, Fig. 7). Heterotrophic bacterial abundance and productiondecreased along the inshore}o!shore gradient (Table 4, Fig. 7). Of the two Synechococcus-typepopulations detected by #ow citometry, the small-sized one (Syn A) also seemed to be associatedwith MAW as its contribution to total cyanobacterial numbers followed the same pattern ofa higher abundance the closer to the coast. Abundances of the prokaryotic autotrophic groups fellin the range of previously reported values for the Mediterranean (Magazzu and Decembrini, 1995;Vaulot et al., 1990), but mean heterotrophic bacterial abundance was higher than values reportedfor the NW or E Mediterranean (Zohary and Robarts, 1992, 1998; Robarts et al., 1996; Gasol et al.,1998; PedroHs-AlioH et al., 1999). The only report on bacterial abundance within the jet of MAW inthe SW Mediterranean (FernaHndez et al., 1994) presented a similar trend as ours, with highernumbers within the core of the jet than in Mediterranean water, but overall lower abundances. Thedepth distribution of prochlorophytes was similar to the typical pattern described for the Mediter-ranean (Vaulot et al., 1990), i.e. maximal densities at surface, and opposed to the pattern describedby Chisholm et al. (1988) for open Atlantic and Paci"c waters. Prochlorophytes and cyanobacterianumbers were strongly correlated, and both groups presented similar abundances with depth in thestations located in the Algerian Current, but prochlorophytes exceeded cyanobacteria in theMediterranean water station (Fig. 7).
4.1. P}E relationships
The higher values of P and E in surface waters, together with the increase in a with depth and
the appearance of photoinhibition in the deep samples can be explained in terms of shadeadaptation of the phytoplankton assemblages from the DCM (Platt et al., 1980; Falkowski, 1981).
In some cases (stations AE8 and AE10), notable di!erences in the photosynthetic parameters werefound even for samples of di!erent depths within the upper mixed layer (Table 5), suggesting thatthe rates of vertical mixing were too slow to induce a similar photoacclimation status for the cells ofthe upper mixed layer (Cullen and Lewis, 1988). It can be speculated that the peculiar P}E response
X.A.G. Mora&n et al. / Deep-Sea Research I 48 (2001) 405}437
Table 8Per meter change in the photosynthetic parameters P , a, b and E for the six stations, calculated as the di!erence
between the maximum and the minimum value (at surface for P and E, and at the DCM for a and b) divided by the
Photosynthetic parameter units in Table 5. *: no photoinhibition at depth.
of the phytoplankton from stations CE4 and CE6, which di!ered from that of the other stations, forinstance in a higher similarity between surface and deep P values (Table 5, Fig. 8), was related to
an in#uence of the cyclonic eddy. Both stations presented the highest P of the data set at the
DCM, despite having the lowest P at the surface. The highest vertical gradients for a and b were
also found at stations CE4 and CE6 (Table 8), suggesting that these charactertistics, like theP values, were associated with the cyclonic circulation. The p pro"le of the transect (Fig. 4)
showed a slight doming of the isopycnals around both stations, associated with a shallowing of thenutriclines for NO and PO (Fig. 5). The highest b value for the DCM sample at station CE6
(Table 5), roughly situated at the center of the cyclonic eddy, would add support to the hypothesisof an upwelling of a strongly shade-acclimated phytoplankton assemblage.
4.2. Integrated phytoplankton and bacterial biomass and production
Previous data of integrated primary production (PP ) for the Algerian Basin or areas under the
in#uence of the MAW jet, for instance the average 880 mg C m d for MAW and470 mg C m d for Mediterranean waters obtained by Lohrenz et al. (1988b), were 1.5}4 timeshigher than those reported here (Lohrenz et al., 1988b; Videau et al., 1994). They were obtainedwith the simulated in situ method, whereas we used photosynthetic parameters, which usually yieldlower PP estimates than in situ methods, in part due to problems with light spectra (e.g. Cullen et
al., 1992; Estrada, in prep.). Our estimates of primary productivity within the Algerian Currentvaried noticeably on a relatively small spatial scale but were on average higher than the valuesusually given for other Mediterranean regions (cf. Sournia, 1973; Estrada et al., 1993; Estrada, 1996;Platt, 1985). Because of constantly sunny conditions during the cruise, variations in incidentirradiance did not induce variability in integrated primary production. The changes in estimatedPP , had we used a constant value of incident irradiance rather than the daily measured ones,
would be as low as $2%. The only measurement of chlorophyll a did not re#ect these pulses ofhigh primary productivity: integrated Chl (Chl ) varied by a factor of 1.6 among the six P}E
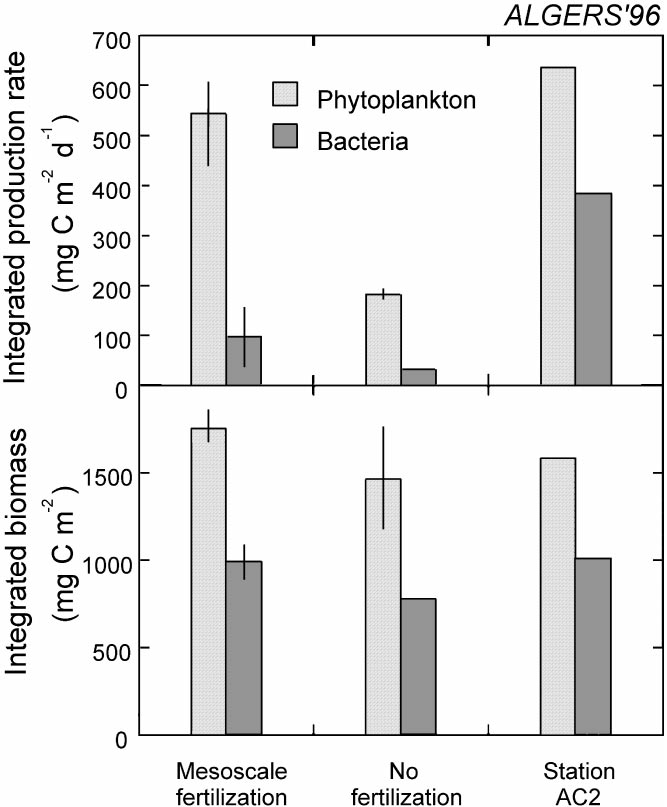
X.A.G. Mora&n et al. / Deep-Sea Research I 48 (2001) 405}437
Fig. 10. Averaged integrated values of phytoplanktonic and heterotrophic bacterial biomass and production underdi!erent hydrodynamical conditions in the Algerian Basin: with mesoscale mechanisms of fertilization (Mesoscalefertilization: stations CE2, CE4, CE6, AE8) and without fertilization (No fertilization: stations AE2 and AE10). StationAC2 was considered separately (see Discussion). Bars represent the stardard deviation of the mean.
stations, while PP varied by a factor of 3.4. Considering all available Chl data (n"28), it still
showed less variability (factor of 2.2) than PP .These results indicate that rates would better
re#ect the responses of planktonic communities to physical forcing than biomass variables.
The primary production results reported here suggest a rapid response of the primary produc-
tion rates to the dynamic conditions in the Algerian Basin. Integrated primary production for theDCM (20}60 m), which made up on average 54% of total PP values, was correlated with two
proxies of upwelling events: temperature at the surface and the depth of the nutricline forphosphate, explaining respectively 75 and 66% of its variance (Fig. 9). Except at the station AE8,located in the frontal zone, PP within the anticyclonic eddy was 2 to 3 times lower than at the
cyclonic eddy stations, re#ecting di!erences in the photosynthetic response of algal assemblages.
These di!erences could in turn be related to fertilization events. Fig. 10 shows the average PP at
the stations a!ected by the di!erent mesoscale mechanisms of nutrient injections into the photiclayer compared with stations that were out of their in#uence. Di!erences were clearer forproduction rates than for the biomass of primary producers. Although the cyclonic eddies formed
X.A.G. Mora&n et al. / Deep-Sea Research I 48 (2001) 405}437
by the Algerian Current do not usually persist longer than a few weeks and present a shallowersignal than anticyclonic ones (Millot et al., 1990), they might be relevant at biological scales,representing episodic events of enhanced primary production (Lohrenz et al., 1988b) not accountedfor in low resolution surveys of regional productivity.
The above discussion does not apply though to the high value of PP found at station AC2 (Fig.
10). Nutrient upwelling was not likely to be the cause, as PO } and NO } concentrations
were only slightly higher than those of the most oligotrophic station (AE10) and the depths of thenutriclines were almost the same as for station AE2, stations that yielded a value more than 3 timeslower than AC2. A possible explanation could be regenerated production based on recyclednutrients, as AC2 also showed the highest rate of bacterial heterotrophic production (BHP ).
Regenerated production could also help explain the high surface value of P found at station
AE10, although PO was depleted down to &100 m depth (Fig. 8, Table 1).
Considering the east}west gradient of increasing bacterial production that emerges from the yet
scarce studies in the Mediterranean Sea (e.g. PedroHs-AlioH et al., 1999; Conan et al., 1999; Gasol etal., 1998; FernaHndez et al., 1994; Zohary and Robarts, 1992; Robarts et al., 1996), the resultspresented here would fall at the end of maximum values in the published ranges, again stressing theimportance of the Algerian Current as harbouring a distinct plankton assemblage. As found forphytoplankton, production rates of heterotrophic bacteria showed higher variability than thecorresponding biomass (Fig. 10), in accordance with PedroHs-AlioH et al. (1999). These authorsdescribed higher BHP /PP and BHP /BB ratios in coastal than in o!shore waters of the
NW Mediterranean, the same trend identi"ed by us (Table 7). This situation perhaps would re#ecta greater contribution of allochthonous DOC to bacterial carbon requirements. The gross bacterialuptake of organic carbon (g-BHP ), estimated using a bacterial growth e$ciency (BGE) of 0.20,
resulted in more than 50% of the primary production being processed through the bacterioplan-kton compartment (Table 7, st AC2 not included). If a value of 0.05 BGE, which could be morerealistic according to Gasol et al. (1998) and del Giorgio and Cole (1998), were used, the systemwould be clearly net heterotrophic, with g-BHP exceeding PP at all stations (from &1.6-fold
up to &12-fold). Taking into account that particulate or total primary production is only a roughapproximation of the organic carbon made available to bacterioplankton (Pace and Cole, 1996),sources of dissolved organic matter other than phytoplanktonic primary production have to befound to account for the total #ow of organic carbon through heterotrophic bacteria. Althoughmuch controversy still exists on BGE in marine systems, except for the most productive areas,a consensus around low values (i.e., &0.10) appears to be arising (see references in del Giorgio andCole, 1998). The relationships between phytoplankton and bacterioplankton would change de-pending on the adopted BGE, but heterotrophic bacteria would still amount to a signi"cant sourceof carbon for higher trophic levels also within the Algerian Current.
In summary, the sampling of two eddies of opposed circulation types allowed us to con"rm
previous statements on the strong physical-biological coupling in the Algerian eddies based onbiomass or ocean colour measurements (Arnone et al., 1990; Millot et al., 1990). This conclusionwas mainly evidenced by the relationships between hydrodynamical variables, as indicators ofmesoscale mechanisms of nutrient fertilization and the values of photosynthetic parameters andintegrated primary production. Production rates presented more variability in response to changesin the physical environment than standing stock variables such as phytoplankton or heterotrophicbacteria biomass. Clear di!erences were also observed in a number of biomass and production
X.A.G. Mora&n et al. / Deep-Sea Research I 48 (2001) 405}437
variables between the Atlantic waters and the Mediterranean waters further north, in accordancewith previous observations (Lohrenz et al., 1988b, Raimbault et al., 1993; FernaHndez et al., 1994).
Finally, biological responses to transient injections of nutrients associated with short-lived cycloniceddies were found, suggesting that the generality and frequence of these eddies should be carefullyconsidered in future determinations of regional production.
We thank the people on board the R/V Hespe&rides for their help during the cruise. We are also
grateful to Dolors VaqueH, Jordi Font, BeleHn MartmHn-MmHguez and two anonymous reviewers forhelpful criticism and comments on a previous version of the manuscript. X.A.G.M. acknowledgesthe receipt of a FPI pre-doctoral fellowship from the Spanish Ministry of Education and Culture.
This work was supported by grants AMB95-0901-C02 (Spanish CYCIT) and MAS3-CT96-0051(European Union MAST Programme, MATER Project).
Arnone, R.A., La Violette, P.E., 1986. Satellite de"nition of the bio-optical and thermal variation of coastal eddies
associated with the African Current. Journal of Geophysical Research 91, 2351}2364.
Arnone, R.A., Wiesenburg, D.A., Saunders, K.D., 1990. The origin and characteristics of the Algerian Current. Journal of
Geophysical Research 95, 1587}1598.
Berdalet, E., MarraseH, C., Estrada, M., Arin, L., MacLean, M.L., 1996. Microbial community responses to nitrogen- and
phosphorus-de"cient nutrient inputs: microplankton dynamics and biochemical characterization. Journal of Plank-ton Research 18, 1627}1641.
Blasco, D., 1971. AcumulacioHn de nitritos a determinados niveles marinos por la accioHn del "toplancton (Accumulation
of NO at certain depths in the ocean by the phytoplankton). Ph.D. Science, University of Barcelona, 231 pp.
Chepurnova, E.A., Gutveyb, C.G., 1988. Distribution and size structure of phototrophic bacterioplankton (Cyanobac-
teria) in the Mediterranean Sea and Atlantic Ocean. Okeanologiya 28, 828}834.
Chisholm, S.W., 1992. Phytoplankton size. In: Falkowski, P.G., Woodhead, A. (Eds.), Primary Productivity and
Biogeochemical Cycles in the Sea. Plenum Press, New York, pp. 213}238.
Chisholm, S.W., Olson, R.J., Zettler, E.R., Goericke, R., Waterbury, J.B., Welschmeyer, N.A., 1988. A novel free-living
prochlorophyte abundant in the oceanic euphotic zone. Nature 334, 340}343.
Conan, P., Turley, C., Stutt, E., Pujo-Pay, M., Van Wambeke, F., 1999. Relationship between phytoplankton e$ciency
and the proportion of bacterial production to primary production in the Mediterranean Sea. Aquatic MicrobialEcology 17, 131}144.
Co(teH, B., Platt, T., 1984. Utility of the light-saturation curve as an operational model for quantifying the e!ects of
environmental conditions on phytoplankton photosynthesis. Marine Ecology * Progress Series 18, 57}66.
Cullen, J.J., Lewis, M.R., Davies, C.O., Barber, R.T., 1992. Photosynthetic characteristics and estimated growth rates
indicate grazing is the proximate control of primary production in the equatorial Paci"c. Journal of GeophysicalResearch 97, 639}654.
Cullen, J.J., Lewis, M.R., 1988. The kinetics of photoadaptation in the context of vertical mixing. Journal of Plankton
Research 10, 1039}1063.
del Giorgio, P.A., Cole, J.J., 1998. Bacterial growth e$ciency in natural aquatic systems. Annual Review of Ecology and
Systematics 29, 503}541.
Dore, J.E., Karl, D.M., 1996. Nitrite distributions and dynamics at station ALOHA. Deep-Sea Research Part II 43,
X.A.G. Mora&n et al. / Deep-Sea Research I 48 (2001) 405}437
Estrada, M., 1996. Primary production in the northwestern Mediterranean. Scientia Marina 60, 55}64.
Estrada, M., MarraseH, C., Latasa, M., Berdalet, E., Delgado, M., Riera, T., 1993. Variability of deep chlorophyll maximum
characteristics in the Northwestern Mediterranean. Marine Ecology * Progress Series 92, 289}300.
Falkowski, P.G., 1981. Light-shade adaptation and assimilation numbers. Journal of Plankton Research 3, 203}216.
Fasham, M.J.R., Platt, T., Irwin, B., Jones, K., 1985. Factors a!ecting the spatial pattern of the deep chlorophyll
maximum in the region of the Azores Front. Progress in Oceanography 14, 129}165.
FernaHndez, M., Bianchi, M., Van Wambeke, F., 1994. Bacterial biomass, heterotrophic production and utilization of
dissolved organic matter photosynthetically produced in the Almeria-Oran front. Journal of Marine Systems 5,313}325.
Font, J., Millot, C., Salas, J., Julia , A., Chic, O., 1998. The drift of the Modi"ed Atlantic Water from the Alboran Sea to the
eastern Mediterranean. Scientia Marina 62, 211}216.
Gasol, J.M., Doval, M.D., Pinhassi, J., CalderoHn-Paz, J.I., Guixa-Boixareu, N., VaqueH, D., PedroHs-AlioH, C., 1998. Diel
variations in bacterial heterotrophic activity and growth in the northwestern Mediterranean Sea. Marine Ecology
* Progress Series 164, 107}124.
Heburn, G.W., La Violette, P.E., 1990. Variations in the structure of the anticyclonic gyres found in the Alboran Sea.
Journal of Geophysical Research 95, 1599}1613.
Herbland, A., Lebouteiller, A., Raimbault, P., 1985. Size structure of phytoplankton in the Equatorial Atlantic Ocean.
Deep-Sea Research 32, 819}836.
Kirchman, D., Sigda, J., Kapuscinski, R., Mitchell, R., 1992. Statistical analysis of the direct count method for
enumerating bacteria. Applied and Environmental Microbiology 44, 376}383.
Kirkwood, D.S., 1992. Stability of solutions of nutrient salts during storage. Marine Chemistry 38, 151}164.
Krom, M.D., Kress, N., Brenner, S., Gordon, L.I., 1991. Phosphorus limitation of primary productivity in the eastern
Mediterranean Sea. Limnology and Oceanography 36, 424}432.
Lacombe, H., Richez, C., 1982. The regime of the Strait of Gibraltar. In: Nihoul, J.C.J. (Ed.), Hydrodynamics of
Semi-Enclosed Seas. Elsevier, Amsterdam, pp. 13}74.
Li, W.K.W., Dickie, P.M., Harrison, W.G., Irvin, B.D., 1993. Biomass and production of bacteria and phytoplankton
during the spring bloom in the western North Atlantic Ocean. Deep-Sea Research Part II 40, 307}327.
Lohrenz, S.E., Arnone, R.A., Wiesenburg, D.A., DePalma, I., 1988a. Satellite detection of transient enhanced primary
production in the western Mediterranean Sea. Nature 335, 245}247.
Lohrenz, S.E., Wiesenburg, D.A., DePalma, I.P., Johnson, K.S., Gustafson, D.E., 1988b. Interrelationships among
primary production, chlorophyll, and environmental conditions in frontal regions of the western Mediterranean Sea.
Deep-Sea Research 35, 793}810.
Magazzu , G., Decembrini, F., 1995. Primary production, biomass and abundance of phototrophic picoplankton in the
Mediterranean Sea: a review. Aquatic Microbial Ecology 9, 97}104.
MartmHnez, R., Arnone, R.A., Velasquez, Z., 1990. Chlorophyll a and respiratory transport system activity in microplan-
kton from the surface waters of the Western Mediterranean. Journal of Geophysical Research 95, 1615}1622.
Massana, R., Gasol, J.M., Bj+rnsen, P.K., Blackburn, N., HagstroKm, As., Hietanen, S., Hygum, B.H., Kuparinen, J.,
PedroHs-AlioH, C., 1997. Measurement of bacterial size via image analysis of epi#uorescence preparations: description ofan inexpensive system and solutions to some of the most common problems. Scientia Marina 61, 397}407.
Millot, C., 1985. Some features of the Algerian Current. Journal of Geophysical Research 90, 7169}7176.
Millot, C., 1994. Models and data: a synergetic approach in the western Mediterranean Sea. In: Malanotte-Rizzoli, P.,
Robinson, A.R. (Eds.), Erice School Proceedings, Ocean Processes in Climate Dynamics: Global and MediterraneanExamples. Kluwer Academic Publishers, Dordrecht, pp. 407}425.
Millot, C., 1999. Circulation in the Western Mediterranean sea. Journal of Marine Systems 20, 423}442.
Millot, C., Taupier-Letage, I., Benzohra, M., 1990. The Algerian eddies. Earth-Science Reviews 27, 203}219.
Mitchell, B.G., Holm-Hansen, O., 1991. Observations and modelling of the Antarctic phytoplankton crop in relation to
mixing depth. Deep-Sea Research 38, 981}1007.
MoraHn, X.A.G., Gasol, J.M., Arin, L., Estrada, M., 1999. A comparison between glass "ber and membrane "lters for the
estimation of phytoplankton POC and DOC production. Marine Ecology * Progress Series 187, 31}41.
Norland, S., 1993. The relationship between biomass and volume of bacteria. In: Kemp, P.F., Sherr, B.F., Sherr, E.B.,
Cole, J.J. (Eds.), Handbook of Methods of Aquatic Microbial Ecology. Lewis Publishers, Boca Raton, pp. 303}307.
X.A.G. Mora&n et al. / Deep-Sea Research I 48 (2001) 405}437
Obaton, D., Millot, C., Chabert D'Hie res, G., Taupier-Letage, I., (in press). The Algerian Current: comparisons between
in situ and laboratory measurements. Deep-Sea Research I, in press.
Pace, M.L., Cole, J.J., 1996. Regulation of bacteria by sources and predation tested in whole-lake experiments. Limnology
and Oceanography 41, 1448}1460.
Pedder, M.A., 1993. Interpolation and "ltering of spatial observations using successive corrections and Gaussian "lters.
Monthly Weather Review 121, 2889}2902.
PedroHs-AlioH, C., CalderoHn-Paz, J.-I., Guixa-Boixereu, N., Estrada, M., Gasol, J.M., 1999. Bacterioplankton and phytop-
lankton biomass and production during summer strati"cation in the northwestern Mediterranean Sea. Deep-SeaResearch I 46, 985}1019.
Peinert, R., Miquel, J.-C., 1994. The signi"cance of frontal processes for vertical particle #uxes: a case study in the
Alboran Sea (SW Mediterranean Sea). Journal of Marine Systems 5, 377}389.
Platt, T., 1985. Phytoplankton production in oligotrophic marine ecosystems: the Mediterranean Sea. In: Moraitou-
Apostolopoulou, M., Kiortsis, V. (Eds.), Mediterranean Marine Ecosystems. Plenum Press, New York, pp. 231}246.
Platt, T., Gallegos, C.L., Harrison, W.G., 1980. Photoinhibition of photosynthesis in natural assemblages of marine
phytoplankton. Journal of Marine Research 38, 687}701.
Porter, K.G., Feig, Y.S., 1980. The use of DAPI for identifying and counting the aquatic micro#ora. Limnology and
Oceanography 25, 943}948.
Raimbault, P., Coste, B., 1990. Very high values of nitrate : phosphate ratio ('30) in the subsurface layers of the
western Mediterranean Sea. Rapports et Proces-Verbaux Reunions Commission International Mer MeHditerraneHe32, C-18.
Raimbault, P., Coste, B., Boulhadid, M., Boudjellal, B., 1993. Origin of high phytoplankton concentration in deep
chlorophyll maximum (DCM) in a frontal region of the Southwestern Mediterranean Sea (Algerian Current).
Deep-Sea Research I 40, 791}804.
Raimbault, P., Rodier, M., Taupier-Letage, I., 1988a. Size-fraction of phytoplankton in the Ligurian Sea and the Algerian
Basin (Mediterranean Sea): size distribution versus total concentration. Marine Microbial Food Webs 3, 1}17.
Raimbault, P., Taupier-Letage, I., Rodier, M., 1988b. Vertical size distribution of phytoplankton in the western
Mediterranean Sea during early summer. Marine Ecology * Progress Series 45, 153}158.
Robarts, R.D., Zohary, T., Waiser, M.J., Yacobi, Y.Z., 1996. Bacterial abundance, biomass, and production in relation to
phytoplankton biomass in the Levantine Basin of the southeastern Mediterranean Sea. Marine Ecology * ProgressSeries 137, 273}281.
Sakshaug, E., Bricaud, A., Dandonneau, Y., Falkowski, F.G., Kiefer, D.A., Legendre, L., Movel, A., Parslow,
J., Takahashi, M., 1997. Parameters of photosynthesis: de"nitions, theory and interpretation of results. Journal ofPlankton Research 19, 1637}1670.
Salas, J., Millot, C., Font, J., GarcmHa-Ladona, E., submitted. Analysis of mesoscale phenomena in the Algerian basin from
drifting buoys and infrared images. Deep-Sea Research I, submitted for publication.
Sieracki, M.E., Johnson, P.W., Sieburth, J. McN., 1985. Detection, enumeration, and sizing of planktonic bacteria by
image-analyzed epi#uorescence microscopy. Applied and Environmental Microbiology 49, 799}810.
Simon, M., Azam, F., 1989. Protein content and protein synthesis rates of planktonic marine bacteria. Marine Ecology
* Progress Series 51, 201}213.
Smith, D.C., Azam, F., 1992. A simple, economical method for measuring bacterial protein synthesis rates in seawater
using H-leucine. Marine Microbial Food Webs 6, 107}114.
Sournia, A., 1973. La production primaire planctonique en MeHditerraneHe: Essai de mise a jour. Bulletin de l'EDtude en
Commun de la MeHditerraneHe (Special Issue) 5, 1}128.
Taupier-Letage, I., Millot, C., 1988. Surface circulation in the Algerian Basin during 1984. Oceanologica Acta (Special
Issue) 9, 79}85.
Taylor, A.H., Geider, R.J., Gilbert, F.J.H., 1997. Seasonal and latitudinal dependencies of phytoplankton carbon-to-
chlorophyll ratios: results of a modelling study. Marine Ecology Progress Series 152, 51}66.
TreHguer, P., LeCorre, P., 1975. Manuel d'analyse des sels nutritifs dans l'eau de mer. Utilisation de l'AutoAnalyzer
2 Technicon, 2nd Edition. Laboratoire d'OceHanographie Chimique, UniversiteH de la Bretagne Occidentale, Brest, 110 pp.
TintoreH, J., Gomis, D., Alonso, S., Parrilla, G., 1991. Mesoscale dynamics and vertical motion in the AlboraHn Sea. Journal
of Physical Oceanography 21, 811}823.
X.A.G. Mora&n et al. / Deep-Sea Research I 48 (2001) 405}437
Vaulot, D., Partensky, F., Neveux, J., Mantoura, R.F.C., Llewellyn, C.A., 1990. Winter presence of prochlorophytes
in surface waters of the northwestern Mediterranean Sea. Limnology and Oceanography 35, 1156}1164.
Videau, C., Sournia, A., Prieur, L., Fiala, M., 1994. Phytoplankton and primary production characteristics at selected
sites in the geostrophic Almeria-Oran front system (SW Mediterranean Sea). Journal of Marine Systems 5, 235}250.
Webb, W.L., Newton, M., Starr, D., 1974. Carbon dioxide exchange of Alnus rubra: a mathematical model. Oecologia 17,
Woods, J.D., 1977. Parameterization of unresolved motions. In: Kraus, E.B. (Ed.), Modelling and Prediction of the Upper
Layers of the Ocean. Pergamon Press, New York, pp. 118}140.
Zohary, T., Robarts, R.D., 1998. Experimental study of microbial P limitation in the eastern Mediterranean. Limnology
and Oceanography 43, 387}395.
Zohary, T., Robarts, R.D., 1992. Bacterial numbers, bacterial production, and heterotrophic nanoplankton abundance in
a warm core eddy in the Eastern Mediterranean. Marine Ecology * Progress Series 84, 132}137.
Source: http://www.ieo-gijon.es/personal/Xelu/Mor%E1n%20et%20al%2001%20(DSRI).pdf
Directors of Pharmacy NHS Scotland Formulary of Pre-Labelled Medicines for Unscheduled Care Second Edition September 2012 FOREWORD This Second Edition of the NHS Scotland Formulary of Pre-Labelled Medicines for Unscheduled Care has built upon the principles of rationalisation outlined in the 1st Edition and has widened the scope of the document to produce a Formulary containing those pre-labelled medicines that are required in unscheduled care settings. In addition, extensive information on anti-infective prescribing and preparations has been included based on best practice outlined with current HPA Guidance. This document is a valuable resource for NHS Scotland and should help to standardise practice across Health Boards. The Working Group is to be congratulated on the work undertaken to review and up-date this guidance and is asked to continue this valuable work in the future. The Directors of Pharmacy will support the local implementation of this revised Formulary and commend this document to you. Evelyn McPhail Chair Directors of Pharmacy NHS Scotland
Les 7 centres de vaccination (27-31.10 ) Halte à la grippe a (H1n1) Die 7 impfzentren os 7 centros de vacinação Centre scolaire et sportif 9a, av. nicolas Kreins Première phase de vaccination réservée aux personnes prioritaires du 27 au 31 octobre 2009 Centre culturel Aal Seeërei rue de l'industrie Pourquoi se faire vacciner ?