Control over large-volume changes of lithium battery anodes via active–inactive metal alloy embedded in porous carbon
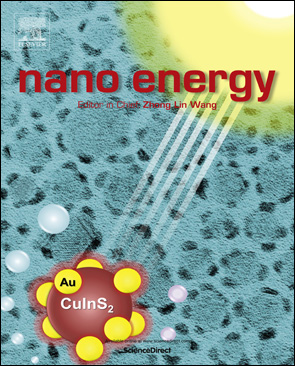
Available online at www.sciencedirect.com
RAPID COMMUNICATION
Control over large-volume changes of lithiumbattery anodes via active–inactive metal alloyembedded in porous carbon
Nasir MahmoodJinghan Sarish Rehman, Quan LiYanglong Hou
aDepartment of Materials Science and Engineering, College of Engineering, Peking University,Beijing 100871, ChinabDepartment of Physics, The Chinese University of Hong Kong, Shatin, New Territory, Hong Kong, China
Received 14 February 2015; received in revised form 26 May 2015; accepted 29 May 2015
Available online 6 June 2015
Lithium ion battery;
Large volume changes and limited access to redox sites of high capacity anode materials are great
challenges. Although, various strategies were adopted but still results are far from required values for
Long cyclic life;
their practical usage. Here, we have designed a unique structure to prevent surface reaction and
structural disintegration meanwhile intrinsic conductivity is improved to involve all redox sites in
conversion reaction. CoSn
x@C–PAn hybrid was synthesized through aqueous chemical route, Co doping
in tin make accessible all redox sites by faster conduction of electrons while its hard nature relaxesinternal stress, carbon shell prevents surface reaction and brings well control on solid electrolyteinterface (SEI) film by maintaining barrier between electrode surface and electrolyte and nitrogendoped porous carbon provides faster diffusion of Li+ deep in electrode make possible high massloadings and conduction highway for electrons. Furthermore, porous carbon also provides room tocompensate volume expansion and keeps electrode structure stable. Because of its unique structurehybrid shows excellent reversible capacity of 2044 mAh/g (retention 100%) with mass loading of3.8 mg/cm2 along with long cyclic life up to 1000 cycles and bears high rate capability (20 A/g).Webelieve that present study makes possible the use of high capacity materials in applications.
& 2015 Elsevier Ltd. All rights reserved.
Presently, lithium ion batteries (LIBs) have got tremendousattention due to their high energy densities and have beenconsidered as promising power source for future electric
E-mail address: . Hou).
N. Mahmood et al.
vehicles (EV) Therefore, in order to achieve the
carbon (N-PGC) matrix named as CoSnx@C–PAn is developed
practical applications of LIBs in EVs, there are numerous
via in-situ polymerization and annealing processes. This
efforts on seeking for high performance anode materials that
nanostructure has multiple advantages: (1) doping of elec-
possess high capacity and excellent stability with long cyclic
trochemically inactive Co prevents structural changes and
life . Metallic tin (Sn) is considered as a potential
enhances internal conductivity of Sn to involve all redox
substitute for conventional graphite anode (372 mAh/g) due
sites in conversion reaction. (2) The nanosized particles can
to its high theoretical capacity (992 mAh/g) and thermal
accommodate large volume strains, hard Co improves CRt
stability . However, structural disintegration, larger
and electrochemical active Sn brings about high capacity.
diffusion barrier, limited access to redox sites and loss of
(3) The mixed phase provides large grain boundary densities
electrical contact have long been identified as primary
for enhanced interfacial Li + storage, channels for faster Li +
reasons for capacity loss and poor cyclic life of Sn-based
diffusion by reducing the diffusion path below 10 nm.
anodes . Although nanochemistry plays a critical role
(4) Carbon shell completely encapsulates nanoparticles
to accommodate volume strains by developing nanosized
(NPs) and thus prevents surface reactions by controlling
structures of Sn, their use is still limited by unstable solid
direct contact with electrolyte and limit SEI on outer side
electrolyte interface (SEI) layer on the surface and poor
with controlled thickness. (5) The carbon framework pro-
internal conductivity As the organic electrolytes
vides faster transport highways for electrons and Li + via
decompose at the working potential of o0.5 V vs. Li+/Li and
pore walls and pores, respectively. (6) High surface area and
forms a thin SEI layer . But the expansion and
well-defined pore size distribution improves the active
contraction of Sn during alloying and de-alloying causes
surface area of the hybrid minimizing percentage of inactive
deformation and breakage of the SEI layer, respectively
material through deep transport of electrolyte and make
. As a result, formation of new SEI on freshly
possible high mass loadings. (7) The existence of large
exposed Sn surface eventually block Li+ transport via accu-
nitrogen contents and graphitic ring (make possible Li6/C6
mulation within SEI and causes poor Coulombic efficiency (CE)
conversion reaction) improve the overall conductivity and
of cell As a consequence, the capacity decays as SEI
electrochemical activity of hybrid to bring high perfor-
thickness increases while most of Sn active material remains
mance. With such a rational design, CoSnx@C–PAn hybrid
electrically connected . However, nanosized designing has
shows excellent discharge capacity of 2044 mAh/g with
improved the cyclic life with enhanced performance but
extraordinary CE and CRt of 100% after 100 cycles at
simple reduction of crystallite size to nanoscale has intro-
0.2 A/g with mass loading of 3.8 mg/cm2. Furthermore,
duced new fundamental challenges . Large surface
hybrid was tested at current density of 10 A/g for 1000
area exposed to electrolyte and higher surface energy that
cycles to explore the stability for long cyclic life and rate
increase side reactions, results in lower CE and causes
capability at higher charge–discharge rates (20 A/g), inter-
thermal runway which leads to internal short circuit due to
estingly, hybrid shows excellent capacity (1256 mAh/g) with
melting of separator –. Furthermore, low tap density
high CE and CRt (100%) after 1000th cycle at current
increases thickness of electrode at high mass loading and
density of 10 A/g. It is believed that the presented design
leads to low volumetric capacity by increasing Li+ transfer
will be very helpful to overcome the large-volume changes
pathway . Generally, poor electrical properties are
in electrode materials for energy storage devices with much
observed due to higher inter-particle resistance which is
improved capacity values.
further dominated by volume changes during charging–dis-charging, affecting cyclic life of electrode drastically A
Experimental section
lot of efforts have been devoted by developing electrolyteblockage layers, creating void space via void engineering and
The synthesis of Sn, Sn@C, PAn, N-PGC and characterization
making their composites with elastically strong graphene and
part are provided in supporting information.
inactive hard metals to sustain the structure and improve thecyclic life of Sn-based electrodes However, to the
best of our knowledge, stability concerns for long cyclic life
2SnO4 nanoparticles
and high performance with good CE and capacity retention(CR
t) at higher mass loadings (43 mg/cm2) has not been
reported yet for Sn-based materials. To achieve this, a careful
(176 mg), CoCl2 6H2O (238 mg) and CTAB (400 mg) are
design of electrode materials, is highly required, that main-
mixed in 20 mL of water and stirred with heating unless
tains faster and deep transfer of Li+, provides better pathway
temperature reaches to 85 1C. Subsequently, 20 ml of 4 M
for electron and ions movement, prevents surface reactions
NaOH solution was added and the mixture was stirred for
with electrolyte and keeps the structural integrity of elec-
60 min at 85 1C. After the completion of reaction, the
trode . Furthermore, Han et al. also investigated theore-
product was collected by centrifugation and washed six
tically that fused graphitic C
times with water and ethanol, repeatedly. Finally, the solid
6 can deliver 42000 mAh/g via
accumulation of Li+ through reversible reaction Li
product was dried at 70 1C for 6 h in a vacuum oven.
Thus, utilizing the above design with conductive networkcontaining fused C
6 backbone can bring higher capacity.
2SnO4–PAn hybrid
Here, we present an architectured design to overcome
the aforementioned problems associated with Sn-based
Co2SnO4–PAn hybrid was synthesized via in-situ polymeriza-
electrodes to bring high performance with longevity at
tion of aniline monomers with Co2SnO4 NPs. Initially 50 mg
low cost. An active–inactive metal alloy sealed in carbon
of aniline monomers was stirred for 5 min in water and pH of
shell and embedded in nitrogen-doped porous graphitic
the solution was turned acidic using HCl. Afterward, the
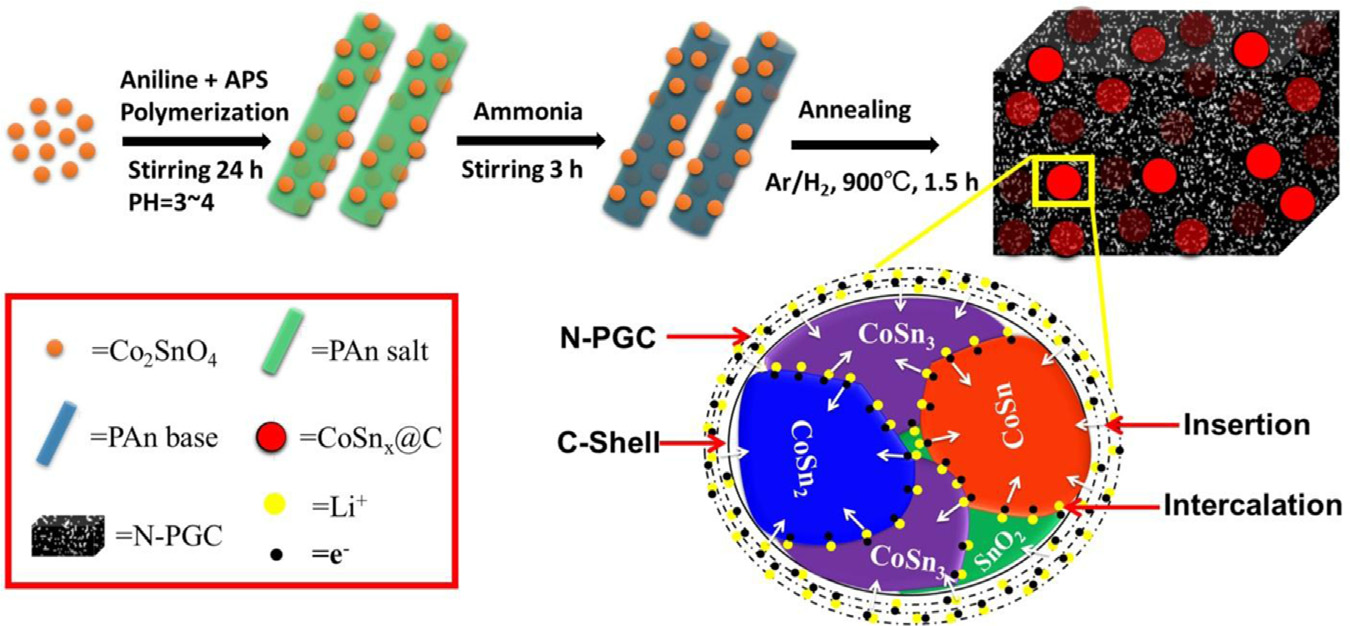
Control over large-volume changes
50 mg of NPs was added and the reaction mixture was
electrochemical properties of Sn. Furthermore, the lithium
stirred further for 10 min. Finally, the addition of APS
storage mechanism is also illustrated in as shown in
(114 mg) as oxidant was done and reaction mixture was
that lithium can be stored through intercalation
stirred for 24 h. After the completion of polymerization
chemistry both in the carbon matrix via Lix/C6 (X=1–6)
addition of ammonia solution was carried out and stirred for
conversion reaction and can contribute capacity up to
further 3 h to turn the PAn salt (green) to PAn base (blue). At
2000 mAh/g depending on the number of lithium intercalated
last, the final product was centrifuge and wash 6 times with
and at Li+ storage at grain boundaries through space charge
water and ethanol repeatedly. The Final product was dried
layer. Further, the diffusion of Li+ becomes easier and faster
at 70 1C for 6 h under vacuum.
through the grain boundaries inside the NPs as shown by thearrows which results faster insertion and conversion reaction.
Synthesis of CoSn
In fact the porous carbon maintain the faster diffusion outside
the NPs and grain boundaries maintain faster diffusion insidethe NPs as results higher rate capability achieved. Further,
CoSnx@C–PAn hybrid was prepared by the thermal annealing
existence of Co inside maintain the continues flow of electron
process. Co2SnO4–PAn hybrid was annealed at 900 1C for
to redox sites and vice versa that utilizes all the redox centers
1.5 h with the heating rate of 2 1C/min under reducing
to bring higher performance, its hard nature prevents the
atmosphere of Ar/H2. Co2SnO4 NPs were also treated under
rapturing of carbon shell, as results direct contact of Sn with
the same condition to obtain CoSnx for comparative study.
electrolyte is inhibited and SEI film was blocked outside thecarbon coating around NPs. Further surface protection blocked
Results and discussion
the undesired side reaction to prevent the formation oflithium dendrites on surface and keep the entire structure
is presenting the synthesis strategy for CoSn
of electrode stable.
hybrid; first Co
To reveal the structure and composition of as-synthesized
2SnO4 NPs were synthesized via wet-chemistry
using co-precipitation of respective salts. Then via in-situ
CoSnx@C–PAn hybrid, the x-ray diffraction (XRD) analysis, x-
polymerization, NPs encapsulated polyaniline (PAn, green as
ray photoelectron spectroscopy (XPS), scanning transmission
emerladine salt) were grown in rod shape morphology which
electron microscope (STEM) and Raman spectroscopy were
were further converted to emerladine base (blue) using NH
carried out. a presents XRD patterns of Co
(the yield of hybrid can be easily increased or decreased by
Co2SnO4–PAn and CoSnx@C–PAn, from where it is observed
controlling the amount of aniline and NPs, without effecting
that Co2SnO4 NPs show their typical peaks well-matched with
electrochemical properties of hybrid). To obtain CoSn
standard card JCPDS no. 29-0514. An additional peak exist at
hybrid, the annealing process of Co
61.41 corresponds to the (220) plane of CoO (JCPDS no.48-
2SnO4–PAn hybrid was
performed at 900 1C for 90 min at a heating rate of 2 1C/min
1719). Interestingly, broad peaks were observed after PAn
under reducing atmosphere, which significantly reduce
grown on NPs due to its amorphous structure, as perceived
from XRD analysis of pure PAn shown in which
2SnO4 NPs to CoSnx alloy and build an uniform carbon shell
on the surface of CoSn
confirms complete encapsulation of NPs without aggrega-
NPs. Furthermore, N-PGC was
obtained from PAn via annealing, has high surface area and
tion. However, after annealing treatment, regular structure
well-defined micropores, additionally annealing effectively
of PAn was changed to porous graphitic carbon and well-
removed existing oxygenated groups that cause thick SEI layer
defined peaks of CoSnx were observed corresponding to
It is perceived that the nanoporous carbons obtained
three phases, CoSn2 (JCPDS no. 25-0256), CoSn (JCPDS no.
from the polymer via pyrolysis will form interconnected
02-0559) and CoSn3 (JCPDS no. 48-1813). Phase segregation
nanochannels and electronically conductive walls for both
of heterogeneous CoSn crystallites with multiple phases
transport, respectively. However, nanocrystals of
observed here is due to higher temperature synthesis
Sn and Sn@C were also synthesized to explore the effect of
It is worth noting that mixed phase has multiple
active–inactive metal alloy and porous carbon substrate on the
advantages over pure phase likewise, high grain boundary
Schematic illustration of synthesis method for CoSnx@C–PAn via in-situ polymerization and annealing processes; further
presenting the mechanism of lithium storage in the hybrid.
N. Mahmood et al.
(a) XRD patterns of Co2SnO4, Co2SnO4–PAn and CoSnx@C–PAn hybrids. (b) HAADF-STEM line profile analysis of CoSnx@C–PAn
hybrid. XPS spectrums of (c) CoSnx@C–PAn hybrid and (d) PAn. (e) BET nitrogen adsorption–desorption isotherm of CoSnx@C–PAn.
(f) Pore size distribution measured using BJH adsorption.
densities enhance the interfacial Li+ storage through space
well-defined reflection peaks exactly matched with
layer charge and thus offer large capacity and provide
JCPDS no. 04-0673, delineating pure phase of Sn NPs
channels for faster transport of Li+ in the NPs ,XRD
b and S5 show the elemental line profile and STEM
pattern of CoSnx@C–PAn also shows two diffraction peaks at
image of a CoSnx@C–PAn, respectively, line scan for bime-
26.61 and 51.71, attributed to (110) and (211) reflections of
tallic alloy was obtained with energy dispersive spectro-
SnO2, respectively (JCPDS no. 41-1445).
scopy (EDS) in high-angle annular dark field STEM (HAADF-
Raman spectrum of CoSnx@C–PAn shows typical D- and G-
STEM). The elemental distribution line for Sn (red) and Co
bands with ID/IG ratio 1.09 which confirms graphitic nature
(black) confirm that the alloy has a consistent chemical
of carbon matrix obtained from PAn ), a little
composition throughout the entire NP with higher concen-
higher intensity of D-band is attributed to the existence of
tration of Sn. Therefore, due to homogenous distribution
NPs . Furthermore, XRD pattern of PAn after annealing
cobalt can easily transfer electron from carbon shell to all
treatment verified the graphitic nature of extracted carbon
internal redox sites to carry on the conversion reaction and
as strong broad peak near 261 ) The
vice versa. Furthermore, faster transfer of electrons to
existence of C =C, C–N, C–H and NH2 was further confirmed
redox sites and accessibility of all redox sites through Co
by Fourier transform infrared (FTIR) spectroscopy, which
doping improved the CRt of the electrode even after long
emphasizes the graphitic nature of carbon with nitrogen
cyclic life. It is also expected that any strain generated by
doping . XRD pattern of Sn is shown in
Sn volume change during lithiation and de-lithiation should
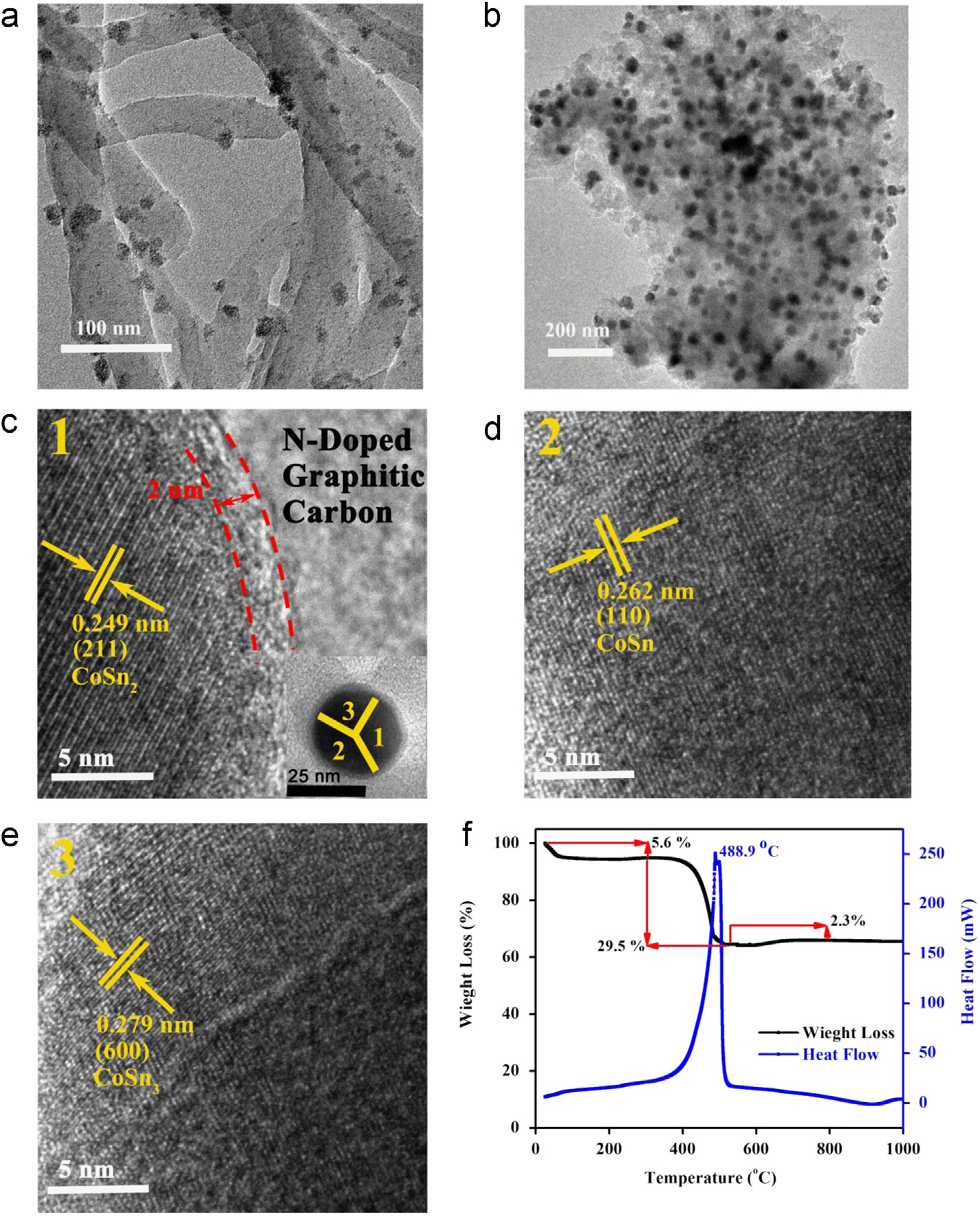
Control over large-volume changes
TEM images of (a) Co2SnO4–PAn hybrid and (b) CoSnx@C–PAn hybrid. (c) HRTEM image of the CoSnx@C–PAn hybrid at position
1 (the inset is enlarge image to show uniform carbon shell on NPs and position of HRTEM analysis), (d) HRTEM image of the CoSnx@C–PAn hybrid at position 2 and (e) HRTEM image of the CoSnx@C–PAn hybrid at position 3. (f) TGA/DSC curves of CoSnx@C–PAn hybrid.
be evenly distributed and compensated by hard counterpart
carbon, which is crucial for limiting the thickness of SEI
(Co). To further confirm the composition of CoSnx@C–PAn
layer on the outer surface of NPs via preventing the direct
hybrid, XPS studies were carried out for both hybrid and PAn
contact of NPs and electrolyte. Surface area and porosity
(and d), the peaks of core levels of Co, Sn, N and C
are important factors that are beneficial in electrochemical
confirms the presence of all chemical species. The concen-
energy storage especially batteries to improve the Li +
tration of each element calculated from XPS is 89.76%,
diffusion . However, fabrication of active nanomaterials
6.08%, 2.50%, 1.56% and 0.10% for C, O, N, Sn and Co,
with porous carbon substrate not only stabilizes structure of
respectively, as XPS is a surface detection technique thus
nanomaterials, but also provides electrical highway to
lower metallic contents were found because of surface
electrons via walls of pores as well as faster pathway to
coverage but still the concentration of Sn is higher than
ions through pores. Furthermore, nanoporosity results in
Co. The presence of little higher concentration of oxygen in
complete wetting of C6 fused aromatic carbon matrix to
CoSnx@C–PAn than PAn suggests that homogeneous precipi-
fulfill the desired conversion reaction of Li6/C6 to bring
tation of single phase CoSn is difficult due to existence of
maximum possible performance as discussed below .
oxidized metallic ions instead of pure metal, in accordance
In present study, PAn was converted to N-PGC by annealing
with XRD results. Further, high resolution Sn3d XPS spectrum
process without any acid or base treatment, and high
shows the existence of oxidized form of tin that is SnO2
surface area of 438.5 m2/g with well-defined pore size of
(Furthermore, the existence of large C peak in
1–1.5 nm was obtained d and e). Homogeneous
XPS results further indicate complete coverage of NPs by
pore size distribution with high surface area provides
N. Mahmood et al.
efficient mass movement and larger space for Li+ storage as
advantages of higher grain boundary density and interfacial
well as relaxes the strains of NPs.
Li + storage at grain boundaries (called buried interfaces)
via space layer charge and brings additional capacity, the NP
Co2SnO4–PAn and CoSnx@C–PAn hybrids are presented in
is characterized by HRTEM at different positions as shown in
. The Co2SnO4 NPs are well-dispersed and embedded
the inset of c. The HRTEM analysis at position 2
in PAn rods without aggregation on surface (a).
(inset of c) shows the inter-planar distance of 0.262
Interestingly, it is found that if polymerizing reagent
nm, which corresponds to (110) plane of CoSn phase that is
(ammonium persulfate, APS) was added before the addition
in accordance with standard card (JCPDS No. 02-0559) as
of NPs, NPs only aggregate on the surface of PAn rods
shown in However, d-spacing of 0.279 nm is
(In fact, addition of APS start polymeriza-
calculated from the HRTEM analysis of position 3 (inset of
tion without NPs, but earlier addition of NPs causes poly-
which corresponds to (600) plane of CoSn3
merization on the surface of NPs in presence of hydrochloric
according to the standard card (JCPDS No. 48-1813) as
acid (HCl) which ionizes the aniline and increase its affinity
shown in Thus, HRTEM studies confirm the
towards NPs, resulting complete encapsulation of NPs and
proposed mechanism () of faster mass transfer
well-define morphology. It is worth noting that NPs are
via grain boundaries and larger Li + storage to bring higher
integrated as dimer or trimer in Co2SnO4–PAn hybrid as
capacity, long stable cyclic life and better rate capability.
compared to individual NPs These dimers and
The content of the metallic constituents (CoSnx) are
trimers fused during heat treatment, transforming into NPs
calculated to be 65% using thermal gravimetric analysis
with average size of 40 nm formed inside N-PGC (
(TGA) as carbon wipe-out at 488 1C through oxidation
the NPs size distribution is shown in The TEM
determined by differential scanning calorimetry (DSC,
image of CoSnx@C–PAn reveals that NPs are evenly distrib-
f). An increase in weight later near 700 1C is due
uted and well embedded in N-PGC matrix. Furthermore, it is
to the oxidation of metallic species. The TEM image of Sn
also perceived that N-PGC matrix has multidimensional
NPs (shows that NPs grew in the size of 20 nm
structure due to random stacking of PAn rods to facilitate
with well-defined structure confirmed through SAED (
the faster mass transportation and enhance the wettability
). A 2.2 nm thick carbon shell is also constructed on
of the C6 matrix. However, the presence of circular rings
pure Sn NPs (Sn@C, ) for the sake of
with bright spots in selected area electron diffraction
comparative electrochemical study with CoSnx@C–PAn.
(SAED) pattern of CoSnx@C–PAn clearly depicts the existence
The benefits of rational design of CoSnx@C–PAn for Li+
of CoSnx alloy in hybrid () and is in accordance
storage were investigated through electrochemical response
with the XRD results mentioned above. It is noted that the
of the obtained hybrid, by developing as anode in coin type
PAn grew in three dimensional networks ,
cell. The redox response of hybrid was delineated by cyclic
mechanism is discussed in supporting information
voltammetry (CV), scanned at 0.2 mV/s and cycled between
), which provides higher surface area to hybrid while PAn
0.005 and 3.0 V vs. Li+/Li a). CV curve shows the
keeps its multidimensional structure even after annealing
similar redox peaks for Sn reaction with Li+ to Li4.4Sn at 1.65
treatment at high temperature (). The aim of
and 0.85 V and extraction of lithium occurred at 0.27 V and
surface protection of NPs was achieved through in-situ
2.1 V during cathodic and anodic sweep, respectively. The
polymerization of aniline monomers on the surface of NPs
peak appear near 0.5 V during cathodic scan corresponds to
rather than construction of simple conduction highway for
the breakdown of electrolyte to form SEI film but no peak
ions and electrons. It is found that during heat treatment
observed in the successive cycles that confirm high reversi-
the aniline attached on NPs surface shaped a uniform shell
bility and control over SEI layer thickness Furthermore, a
of carbon (the inset in c) and additional PAn
small peak appears at 1.35 V confirms the synergism among
developed N-PGC. It is well-known that NPs size distribution
the CoSnx core and N-PGC matrix It is worth noting that
strongly affects the electrochemical performance and tap
no peak was observed during anodic scan for Co which
density of nanomaterials. To obtain narrow size distribution,
demonstrates its completely inactive nature Moreover,
heat treatment at different rates (2, 5 and 10 1C/min) was
to confirm the high rate capability of hybrid, CV scans at
carried out (). It is scrutinized that
different rates were carried out, as shown in . It is
as heating rate was reduced from 10 to 2 1C/min, size
worth noting that similar profile of CV curves at different scan
distribution became narrower and remarkably carbon shell
rates were obtained, which assures high rate capability of the
formed only at lower heating rate. Furthermore, longer
hybrid and easy access of redox sites due to unique internal
time annealing was also adopted with aim to get rid of oxide
structure. In contrast to hybrid electrode, the electrode of Sn
species but it result in poor size distribution which is not
shows huge difference among first and successive cycles that
suitable for better performance (To further
confirm the formation of very thick SEI layer on the surface of
confirm the structure and existence of electrolyte blockage
electrode and further reduction of current intensity in
layer, high resolution TEM (HRTEM) studies were carried out,
successive cycles confirm the reformation of SEI
from c it can be seen that the surface of NP is
because raptured surface exposed fresh surface of tin that
completely covered by 2.2 nm uniform carbon shell.
increases SEI film thickness via irreversible storage of Li+.
Furthermore, inter-planar distance measurements make
The voltage profiles of hybrid b) exhibited typical
sure the existence of both electrochemically active (Sn)
electrochemical features of CoSn (here broader voltage range
and inactive (Co) metals, the measured d-spacing of
of 5 mV to 3 V was used for the complete exploration of
0.249 nm at position 1 (inset of corresponds to
stability of electrode developed with unique composition but
the (211) plane of CoSn2 (JCPDS no. 25-0256) as shown in
the redox reaction is in the limits of anode cut of voltage)
c. To explore the segregation of multiphase,
with almost no change over 100 cycles. Further,
Control over large-volume changes
(a) Cyclic voltammograms of CoSnx@C–PAn at scan rate of 0.2 mV/s in the voltage range of 0.005–3 V vs. Li+/Li.
(b) Galvanostatic charge–discharge curves of CoSnx@C–PAn hybrid cycled 1st, 2nd, 50th and 100th tested at current density of 0.2 A/gin the range of 0.005–3 V vs. Li+/Li. (c) Cyclic performance, capacity retention and Coulombic efficiency of CoSnx@C–PAn for 100cycles at current density of 0.2 A/g in the voltage range of 0.005–3 V vs. Li+/Li. (d) The cyclic performance and Coulombic efficiencyof CoSnx@C–PAn at different current densities in the voltage range of 0.005–3 V vs. Li+/Li.
absence of large plateaus confirms the faster transfer of
of its first lithiation process and result in capacity of
lithium inside the electrode through porous carbon and grain
2037.8 mAh/g. The loss of 341.6 mAh/g capacity is because
boundaries as proved by HRTEM studies c–e)
of the SEI film formation that was overcame in the next cycles
in contrast to the electrode that shows large irreversible
with improved CE. Furthermore, the plateaus in the first
storage of lithium in the first cycle and in successive cycles
discharge curve well concise with CV curve and show two
required large time to diffuse, resulting poor CRt ().
major changes first the lithium storage through the conversion
Furthermore, it is point of ponder that faster diffusion of
reaction with Sn; second through insertion in the carbon
Li+ is necessary for high rate capability and the linear profile
matrix as indicated by the plateau below 0.5 V that continues
is indication of faster diffusion through shorter distance
till the cut off voltage reaches. Further to explore the
(below 10 nm) . In fact, the grain boundaries serve as
practical utilization of as-developed hybrid, its electrochemi-
tunnels inside the NPs for faster transfer of Li+ and Co
cal performances were explored in various voltage widows
provides faster electron flow to redox sites as results an
(0.005 to 1.5 and 0.005 to 0.6 V) as for practical utilizations
efficient redox reaction happened in a short time, thus result
the upper voltage limit of anode should be restricted below
in linear charge-discharge profile with improved performance
1.2–1.3 V . It is worth noting that hybrid outperformed
as discussed by Gogotsi and Okubo et al. The CE is an
even in the restricted voltage windows and shows the
indicator of the reversibility of the electrochemical reaction
discharge capacities of 1524.76 and 880.12 mAh/g after 100
at electrode . The decrease in CE usually happens due to
cycles with CRt of 99% at current density of 0.2 A/g in the
rupture and reformation of SEI layer, especially in later
voltage range of 0.005–1.5 V and 0.005–0.6 V, respectively as
cycles. After initial few cycles, CE remains nearly 100% during
shown in In addition the CE values reach to 100%
charging and discharging process of the hybrid electrode
after initial few cycles that further emphasized the high
(c and d). It should be noted that low areal mass
reversibility of the hybrid in the restricted voltage windows.
loadings were frequently used to achieve the stable cycling
The stable CE and high CRt demonstrated that hybrid is highly
life. However, high mass loadings are needed to realize the
feasible for deep diffusion of Li+ and all material is active in
high performance for electrification of road market The
thick electrode. To observe the effect of mass loading on the
hybrid shows first discharge capacity of 2379.4 mAh/g with
performance of hybrid 4 electrodes were assembled with
mass loading of 3.8 g/cm2 (further the tap density of the
different mass loadings (2–4 mg/cm2) and it was found that
as-synthesized hybrid is 0.46 g/cm3 and this is in the desirable
increasing mass loading has minimal effect on performance
range for electrode material as Sn based materials show large
. Furthermore, similar performance shows high
volume changes) at current density of 0.2 A/g and capacity
repeatability of the results and further exclude the influence
retention was as high as 100% (calculated from 2nd discharge)
of other factors likewise cell assembly, testing conditions etc.
after 100 cycles. While the hybrid shows 85.64% de-lithiation
Stable structure at particle level is highly required to achieve
N. Mahmood et al.
stable long cycling of a high mass loaded electrode, because
(Rct) were measured using appropriate Randles equivalent
electrode level cracking and failure can be possible even with
circuit shown in The calculated Rf and Rct
small changes in particle morphology which accumulate
before testing is 16.39 Ω and 72.14 Ω that slightly increased to
across the thickness of electrode. A successful design of the
18.53 Ω and 75.14 Ω after 1000 cycles of testing, respectively.
hybrid structure is indicated by stable and excellent perfor-
Vertical spikes confirmed the capacitive behavior of hybrid but
mance at high mass loading. To explore the rate capability,
a little increment in ions diffusion resistance (Warburg impe-
hybrid was tested at various current densities and it is found
dance, W) was observed after long cyclic life. Better electron/
that hybrid keeps its reversibility with changing current
ion conductivity over prolonged cycles with strong resistance
densities as shown by stable CE d). The hybrid keeps
control for successive charge–discharge cycles suggest extra-
its reversible capacity as high as 551 mAh/g at higher current
ordinary CRt, CE and high stability of hybrid. To the best of our
density of 20 A/g and restores its excellent performance of
knowledge, long stable life with excellent performance at such
2040.5 mAh/g with decrease in current density to 0.2 A/g
high mass loadings is rarely reported for Sn-based electrodes.
d). Thus, high rate capability along with higher
Synergistic effect of Co doping, C coating and N-PGC with
performance proves the reliability of the developed method
Sn was analyzed by comparative electrochemical studies of
to control over large volume changes of anode materials.
PAn, Sn, Sn@C, Co2SnO4, Co2SnO4–PAn, CoSnx and CoSnx@C–
Furthermore, hybrid was tested for 1000 cycles at 10 A/g to
PAn. It is simply perceived from that after making
evaluate the long cyclic life of electrode. It is found that hybrid
hybrids of two structures the performance increased, which
keeps a capacity of 956.4 mAh/g after 1000th cycle with high
indicated that materials took successfully the advantages of
CRt of 98.5% (only 0.0015% capacity loss per cycle) and stable
both components for better performance due to strong
CE around 100% The stability of structure after
interfacial interactions. The performance of Sn@C is
electrochemical test was examined through TEM and HRTEM
increased after carbon coating but still a large capacity loss
after washing electrode material with acid to remove SEI layer
was observed in the initial cycles. The fact that increasing
). From microscopic analysis, it is evaluated that the
the electronic conductivity of Sn via carbon coating can
structure (measured d-spacing of 0.199 nm corresponds to (310)
improve the performance through faster Li+ diffusion, but
plane of CoSn2 (JCPDS no. 25-0256)) and size of NPs in hybrid
lithium intercalation reaction (Li4.4Sn) may be limited by
are preserved after long cycling test. Moreover, the stability
electron transfer between the carbon coating and redox site
concern of hybrid was examined by electrochemical impedance
in the crystal It is noticeable that when the intrinsic
spectroscopy (EIS) before and after 1000 cycles .
conductivity of Sn was increased through Co doping, an
Similar Nyquist profile was obtained for hybrid before and after
improved performance was achieved but capacity loss at
1000 cycles as both curves show semicircle in high frequency
initial cycles still exist because of surface reactions. Thus, by
region and a straight vertical inclined line in lower frequency.
combining Co doping and constructing shell of carbon in one
The SEI layer impedance (Rf) and charge transfer resistance
(a) Cyclic performance, capacity retention and Coulombic efficiency of CoSnx@C–PAn for 1000 cycles at current density of
10 A/g in the voltage range of 0.005–3 V vs. Li+/Li. (b) Nyquist plots of CoSnx@C–PAn hybrid before and after 1000 cycles of charge–discharge in the range of 100 kHz to 10 mHz at open circuit potential (the inset is Nyquist plot with equal x–y axis ratio at highresolution). (c) Comparison of discharge capacities of CoSnx@C–PAn, CoSnx, Co2SnO4–PAn, Co2SnO4, Sn@C, Sn and PAn at currentdensity of 0.2 A/g in the voltage range of 0.005–3 V vs. Li+/Li.
Control over large-volume changes
performance is attained with high CRt. Further the incor-
of 3.8 mg/cm2. Furthermore, hybrid shows long cyclic stability
poration of hard Co also prevents the rapture of C shell
for 1000 cycles of charge–discharge at current density of 10 A/g
around the NPs by compensating the internal stresses as over
with capacity of 1256 mAh/g after 1000th cycle and impedance
expansion of NPs causes the rapturing of the C shell It is
calculations confirm slight change in resistances after 1000
worth noting that carbon matrix with well-defined pore size
cycles. The rate capability of the hybrid is explored by testing
(1–1.5 nm) provide highway to both electrons and ions
at different current densities and hybrid keeps 551 mAh/g even
through pore walls and pores, respectively. These pores
at 20 A/g. The approach to build active–inactive metal phase
provide tunnels for deep and fast diffusion of Li+ in thick
encapsulated in carbon shell and embedded in N-PGC adaptable
electrode to keep the entire material active, which is further
matrix established here opens up a new avenue to control
facilitated by the internal grain boundaries of NPs to achieve
volume changes and brings high performance of tin-based
faster redox reaction by shortening the internal diffusion
anode, can also be extended to other attractive anode (like
path. The relative sizes of Li+ ions and pores of the electrode
Silicon, Germanium, etc.) and cathode materials systems that
materials are also very important in determining the poten-
suffer large structural changes during conversion reactions.
tial performance. In our designed system, Li+ (0.076 nm) caneasily diffuse through the electrode materials (pore size
Supporting information
1.5 nm) not only providing higher performance but alsohigher rate capability . In addition, higher capacity
Supporting Information is available from the Elsevier or the
is observed than the theoretical capacity of Sn, this addi-
author [part of experimental section, XRD of PAn before and
tional capacity comes up from the interfacial storage of Li+
after annealing, Raman and FTIR results of hybrid, XRD of Sn
due the existence of mixed phase by space layer charge that
NPs, STEM image of hybrid, TEM images of hybrid, Co
is energetically favorable at interface of two phases as shown
NPs, PAn before and after annealing, hybrid at different
in , intercalation of Li+ in N-PGC through conver-
temperature, high resolution spectra of Sn3d and Co2p, Sn
sion reaction of Lix/C6 that contributes large capacity and
and Sn@C NPs, Hybrid after testing, SAED of hybrid and Sn
synergistic effect between NPs and N-PGC The
NPs, HRTEM, of hybrid after testing, NPs distribution graph,
existence of nitrogen in the carbon make it more electro-
PAn polymerization reaction mechanism, CV of Sn NPs,
chemical active that results higher performance (c)
capacity performance of hybrid at different mass loading,
and contribute lager capacity to hybrid performance. As,
behavior of bulk and nanomaterials towards Li + storage,
nitrogen is capable to change the electronic structure and
Nyquist plot and equivalent circuit diagram].
density of state to improve the conductivity and capacity ofgraphitic carbon To further explore the synergism ofdifferent components, EIS studies were done and represen-
tative Nyquist plots are shown in In the light ofabove results, the optimized cycling stability and rate
This work was supported by the NSFC-RGC Joint Research
capability of hybrid is attributed to the enhanced transport
Scheme (51361165201), NSFC (51125001 and 51172005),
kinetics and structural stability. The high transport kinetics
Beijing Natural Science Foundation (2122022), Aerostatic
comes from N-PGC conductive network that provides faster
Science Foundation (2010ZF71003) and Doctoral Program of
electronic highway from current collector to active material
the Ministry of Education of China (20120001110078).
and among the separated NPs as well as Co improves theintrinsic transfer of electron from carbon to redox sites which
Supporting information
make available all the material for higher performance. Thehomogenously porous structure and anisotropic adhesion
Supplementary data associated with this article can be
realizes efficient mass transfer of Li+. Furthermore, the
found in the online version at
structure is protected via accommodating internal strains by
hard Co, preventing surface reactions by carbon shell andpresence of adaptable matrix of N-PGC.
In summary, CoSnx@C–PAn hybrid has been fabricated utilizing
low-cost and large scale aqueous chemical growth under
industrially acceptable conditions to control large volume
changes and make accessible all active sites of Sn-based anode
via active–inactive metal phase embedded in N-PGC matrix.
The hybrid efficiently took the advantages of mixed phase,
enhanced internal conductivity by hard Co doping, surface
protection by carbon shell and high surface area (438.5 m2/g)
with well-defined pore size (1–1.5 nm) to improve the transport
kinetics, lithium intercalation reaction (Li6/C6) in fused C6
aromatic ring of N-PGC and structural stability. The hybrid
possess high capacity of 2044 mAh/g with extraordinary CE and
CRt of 100% after 100 cycles at 0.2 A/g with the mass loading
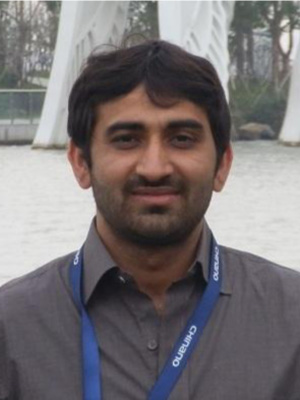
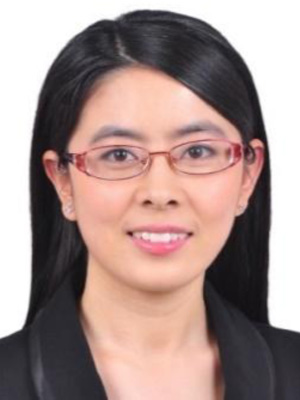
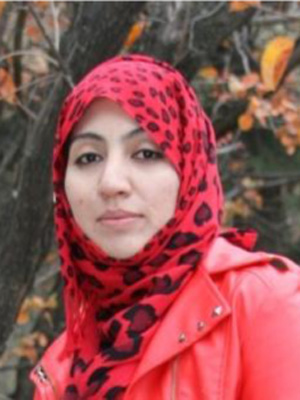
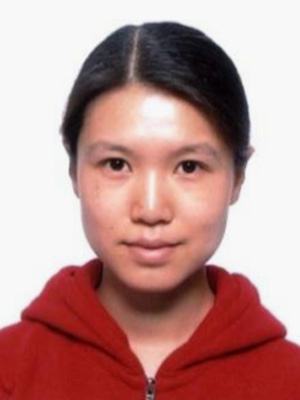
N. Mahmood et al.
Nasir Mahmood obtained his BS degree in
2009 in Chemistry from Punjab University
and MS degree in 2011 in Materials and
Surface Engineering from National Univer-
sity of Science and Technology, Pakistan. He
joined Peking University in 2011, where he
is currently pursuing his Ph.D in Materials
Science and Engineering under the guidance
of Prof. Yanglong Hou. His research involves
the synthesis of graphene/graphene-based
nanomaterials and their application in energy storage and conver-
sion devices.
Jinghan Zhu received her B.S. in Materials
Science and Engineering from the University
of Science and Technology Beijing (USTB,
China) in 2011. She has been pursuing her
Ph.D under the supervision of Prof. Yanglong
Hou in the Department of Materials Science
and Engineering at Peking University since
2011. Her research interests are the chemical
synthesis of graphene based nanomaterials
and their magnetic and catalytic applications.
Sarish Rehman obtained his BS degree in
Chemistry from Peshawar University in 2010
and her MS degree in 2013 in Materials and
Surface Engineering from the National Uni-
versity of Science and Technology, Pakistan.
She joined Peking University in 2013, where
she is currently pursuing her Ph.D in Materi-
als Science and Engineering. Her research
focuses on the synthesis and development
of novel nanomaterials for the application
in energy storage and conversion devices.
Quan Li received her Ph.D in Materials Science
and Engineering from Northwestern University
in 2001. She joined The Chinese University of
Hong Kong as an assistant professor in 2002,
and was promoted to full professor in 2011.
Her research focuses on functional materials,
including the fabrication and assembly of
nanomaterials, characterizations and mea-
surements of individual nanostructures, and
their applications in energy and biomedicine.
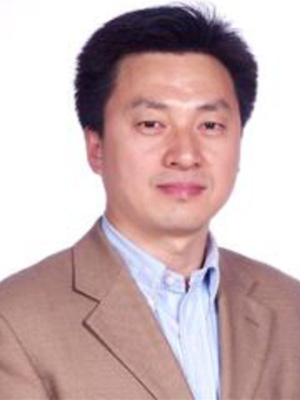
Control over large-volume changes
Yanglong Hou received his Ph.D in Materials
research interests include the design and chemical synthesis of
Science from Harbin Institute of Technology
functional nanoparticles and graphene, and their biomedical and
(China) in 2000. After a short post-doctoral
energy related applications.
training at Peking University, he worked atthe University of Tokyo from 2002 to 2005 asJSPS foreign special researcher and also atBrown University from 2005 to 2007 aspostdoctoral researcher. He joined PekingUniversity in 2007, and now is a Chang JiangChair Professor of Materials Science. His
Source: http://www.phy.cuhk.edu.hk/qli/publication/2015/Control%20over.pdf
The new england journal of medicine Alastair J.J. Wood, M.D., Editor New Drugs for Rheumatoid Arthritis Nancy J. Olsen, M.D., and C. Michael Stein, M.B., Ch.B. heumatoid arthritis affects approximately 1 percent of the From the Divisions of Rheumatology(N.J.O., C.M.S.) and Clinical Pharmacology
Prevalence of diabetes, obesity, and metabolic syndrome in subjects with and without schizophrenia (CURES-104)Subashini R, Deepa M, Padmavati R1, Thara R1, Mohan V Epidemiology and Background: There are some reports that diabetes and metabolic syndrome (MS) are more prevalent among Diabetology, Madras Diabetes Research schizophrenia patients. However, there are very few studies in India which have estimated the prevalence