Contrasting reproductive strategies of triploid hybrid males in vertebrate mating systems
doi: 10.1111/jeb.12556
Contrasting reproductive strategies of triploid hybrid malesin vertebrate mating systems
*Institute of Evolutionary Biology and Environmental Studies, University of Zurich, Zurich, Switzerland†Department of Zoology, Faculty of Natural Sciences, Comenius University in Bratislava, Bratislava, Slovak Republic
‡Laboratory of Fish Genetics, Institute of Animal Physiology and Genetics, Libechov, Czech Republic
§Department of Zoology, Faculty of Science, Charles University in Prague, Prague, Czech Republic
all-hybrid population;
The scarcity of parthenogenetic vertebrates is often attributed to their
asexual reproduction;
‘inferior' mode of clonal reproduction, which restricts them to self-repro-
gamete production;
duce their own genotype lineage and leaves little evolutionary potential
with regard to speciation and evolution of sexual reproduction. Here, we
show that for some taxa, such uniformity does not hold. Using hybridoge-
netic water frogs (Pelophylax esculentus) as a model system, we demonstrate
that triploid hybrid males from two geographic regions exhibit very different
reproductive modes. With an integrative data set combining field studies,
crossing experiments, flow cytometry and microsatellite analyses, we found
that triploid hybrids from Central Europe are rare, occur in male sex onlyand form diploid gametes of a single clonal lineage. In contrast, triploidhybrids from north-western Europe are widespread, occur in both sexes andproduce recombined haploid gametes. These differences translate into con-trasting reproductive roles between regions. In Central Europe, triploidhybrid males sexually parasitize diploid hybrids and just perpetuate theirown genotype – which is the usual pattern in parthenogens. In north-wes-tern Europe, on the other hand, the triploid males are gamete donors fordiploid hybrids, thereby stabilizing the mixed 2n-3n hybrid populations. Bydemonstrating these contrasting roles in male reproduction, we draw atten-tion to a new significant evolutionary potential for animals with nonsexualreproduction, namely reproductive plasticity.
(Bengtsson, 2009; rev. in Sch€
on et al., 2009). Partheno-
genetic (also called unisexual) vertebrates mostly arose
In vertebrates, a little more than 0.1% of extant species
by hybridization between two phylogenetically related
reproduce by parthenogenesis sensu lato, that is by apo-
sexual species (Vrijenhoek et al., 1989; Avise, 2008;
mictic and automictic parthenogenesis, gynogenesis or
Choleva et al., 2012; but see Sinclair et al., 2010). Com-
hybridogenesis (for details see Suomalainen et al., 1987;
bining two different, independently evolving genomes
Parker & Niklasson, 1999; Vrijenhoek, 1999; Neaves &
of sexual progenitors leads to difficulties in pairing of
Baumann, 2011). Comparative studies of these repro-
divergent homologs during gametogenesis. This has
ductive modes are not only important for understand-
modified the normal meiotic cycle in hybrids so that
ing the evolution of parthenogenesis and explaining
chromosome segregation and recombination is absent
the paradox of sex (Otto & Lenormand, 2002), they
or limited during gametogenesis. Occasionally the mei-
also yield a deeper understanding of the origin of
otic problems also result in the production of diploid
gametes which, after fusion with haploid or diploidones, produce triploid or tetraploid individuals (Sten-berg & Saura, 2009). The preconditions and the evolu-
Correspondence: Heinz-Ulrich Reyer, Institute of Evolutionary Biology
tionary role of polyploidy plays in animal systems are,
and Environmental Studies, University of Zurich, Winterthurerstrasse
however, still widely debated (Cunha et al., 2008;
190, Zurich 8057, Switzerland. Tel.: +41 44 635 49 80; fax: +41 44 63557 11; e-mail:
[email protected]
Christiansen & Reyer, 2009; Choleva et al., 2012).
(e.g. Uzzell et al., 1975; St€
ock et al., 2010b, 2012).
whereas fish and amphibians are sperm-dependent par-
Together, these two factors may result in dynamic
thenogens. Therefore, the latter can be considered as
reproductive relationships (Alves et al., 2001). This can
sexual parasites that must live and mate with an ances-
lead to the formation of a new bisexual species via
tor (the sexual host) to obtain the sperms that are nec-
polyploid speciation (Cunha et al., 2008), or play a key
essary for the parthenogen's reproduction (Vrijenhoek,
role in maintaining bisexual hybrid populations by
1989). In one form, hybridogenesis, the parasitic taxa
releasing the hybrid from its reproductive dependence
have a hemiclonal heredity mode, because only one of
on a sexual progenitor (G€
unther, 1975; G€
their parental genomes is transmitted to the next gener-
1979; Christiansen & Reyer, 2009).
ation, whereas the second parental genome is elimi-nated prior to meiosis. True syngamy between a
The Pelophylax esculentus study system
haploid clonal gamete (called a hemiclone sensu Vrijen-hoek, 1979) from the hybridogenetic hybrid and a
In this study, we address the origin and test ambiguous
recombined gamete provided by the parental species
reproductive roles of male polyploidy in P. esculentus
whose genome has been eliminated in the hybridogens
hybrid water frogs by comparing new results from a
reconstitutes a hybrid state in the progeny. Therefore,
detailed investigation on a local scale with previously
maternal and paternal genomes do not recombine,
published results on a wide geographic scale (Pruvost
except on rare occasions (Vorburger, 2001b; Guex et al.,
et al., 2013a). The P. esculentus complex includes two
2002; Schmeller et al., 2005; Lamatsch & St€
sexual species, Pelophylax lessonae (Camerano, 1882),
The general rareness of unisexual vertebrates is
the pool frog (genotype LL), and Pelophylax ridibundus
attributed to the necessity to overcome several prob-
(Pallas, 1771), the marsh frog (RR). From their primary
lems before they can establish themselves within a nar-
hybridization originated, and still originates, the bisex-
ual hybridogenetic P. esculentus (Linnaeus, 1758), the
hypothesis; Moritz et al., 1989). These problems include
edible frog (genomic composition LR) (Fig. 1a). In most
genetic incompatibilities between nonrelated parental
of the species' European range, diploid P. esculentus live
genomes in hybrids, segregation of parental genomes
in sympatry with P. lessonae. In these so-called L-E sys-
during meiosis and finding an ecological niche in com-
tems, the hybrid excludes its haploid L genome, trans-
petition with their progenitor species. Another conse-
mits in its gametes the haploid R genome and restores
quence is that most unisexuals maintain a single clonal
hybridity in the new generation by obtaining the L
reproductive mode within a mating complex, irrespec-
genome from mating with P. lessonae (Fig. 1b). In some
tive of whether they are of a monophyletic origin (e.g.
populations, the mirror images, so-called R-E systems,
North American hybrid fish Poecilia formosa; St€
are found. Here, most diploid P. esculentus hybrids
2010a), or of an ongoing polyphyletic origin (e.g. Euro-
exclude the R, transmit their L genomes and mate with
pean hybrid fish of the genus Cobitis; Choleva et al.,
P. ridibundus to perpetuate the hybrid populations
2008; Janko et al., 2012). Hence, unisexual vertebrates
(reviewed by Graf & Polls Pelaz, 1989; Pl€
otner, 2005).
are generally considered as taxa with a low evolution-
In several areas of the species' range also triploid
ary potential in terms of speciation and evolution of
hybrids have been found. This is especially true for
sex. Their uniform reproductive mode, so the argu-
northern European regions belonging to the drainage
ment, allows for a single role only: to self-reproduce
basins of the North Sea and the Baltic Sea (Berger,
their own genotypes or individual lineages (e.g. Vrijen-
1988b; Rybacki & Berger, 2001; Pl€
otner, 2005). In this
hoek, 1989; Maynard-Smith, 1992).
area, the most frequent population structure is the one
This, however, is not always true. Some of these
with no parental species and two or three types of
mating systems display reproductive plasticity with
hybrids: diploid LR in sympatry with triploid hybrids,
signs of an evolutionary potential. This plasticity is
mostly with LLR, but also with LRR or both (Christiansen
achieved through at least two co-occurring factors.
& Reyer, 2009; Arioli et al., 2010; Jakob et al., 2010; Pru-
First, although hybrid males are usually rare and sterile
vost et al., 2013a). In those all-hybrid populations, trip-
(e.g. Choleva et al., 2012), functional hybrid males
loids of both genomic compositions (LLR and LRR) are
occur regularly in some taxa. These include the
usually formed by fusion of diploid clonal LR eggs pro-
hybridogenetic water frog Pelophylax esculentus (Graf
duced by LR females with haploid recombined L or R
& Polls Pelaz, 1989; Polls Pelaz, 1994; Christiansen
sperm of LLR or LRR males, respectively (see Fig. 1d for
& Reyer, 2009), the fishes Squalius alburnoides (Alves
the LR/LLR populations). Diploid hybrids (LR) can arise
et al., 2001) and Hypseleotris (Schmidt et al., 2011), and
from the fusion of haploid-recombined L and R gametes
the Palearctic green toad of the Bufotes viridis complex
of male and female LLR and LRR, respectively, and from
ock et al., 2010b). Second, a single hybrid genotype
the fusion of recombined L eggs of LLR females and hap-
of the above mentioned taxa can often produce more
loid clonal R sperm of LR males (for details see Christian-
than one type of gametes with some level of recombi-
sen, 2009 and Christiansen & Reyer, 2009). Therefore,
nation between the conspecific genomes in polyploids
by providing recombined haploid gametes in E-E
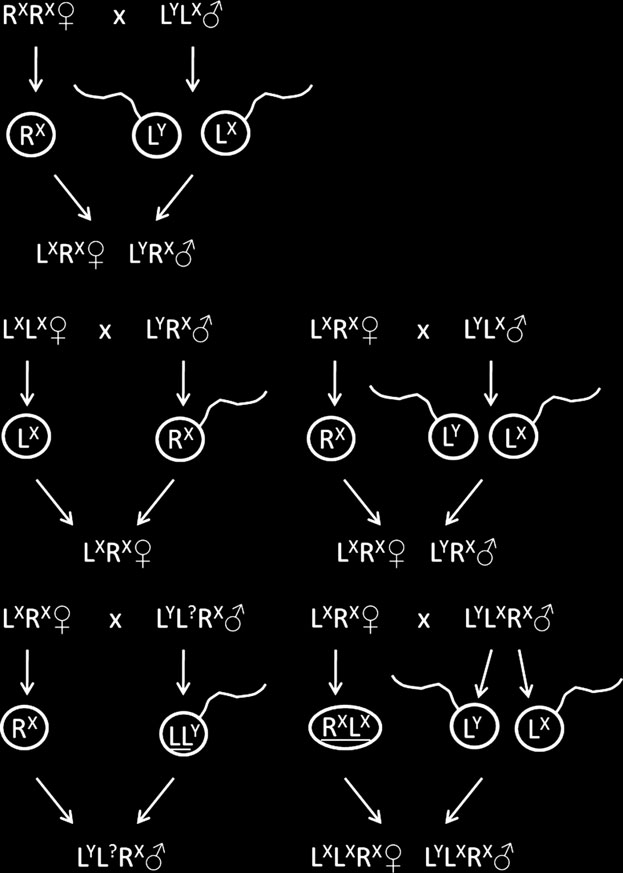
Reproductive strategies in triploid frogs
We, therefore, sampled the area with Central Euro-
pean triploid P. esculentus populations to address the ori-gin
understand how polyploid vertebrates can evolve fromtheir sexual ancestors and to investigate whether theyuse different reproductive modes in different geographicareas. We particularly studied the following four topics:(I) The structure of populations in terms of genotypes,ploidy levels and sex ratios; (II) Gamete types of dip-loids and triploids, and formation of triploids; (III) Therole of triploids within the breeding system; and (IV)
Single or multiple origin and nature of hemiclonallytransmitted genomes. Here, we integrate multiple typesof data from European water frogs to demonstrate con-trasting reproductive pathways (self-reproducing modeor contributing to perpetuate the hybrid population)found within a single parthenogenetic mating system(P. esculentus complex), genotype and sex.
Materials and methods
To address the origin and role of polyploidy in waterfrog systems, we combined multiple types of data. Wedid a comparative field study (for topic I), performedartificial crossing, conducted microsatellite analysesexperiments and flow cytometry on sperms (for topicsII and III) and compared gamete production patterns,triploid formation and hemiclonal lineages among theeight populations from our study area in Central Eur-ope (for topic IV).
Fig. 1 Origin of (a) diploid Pelophylax esculentus (LR) from primaryhybridization between Pelophylax lessonae males (LL) and Pelophylax
ridibundus females (RR) and (b) perpetuation of diploid and (c)
During springs 2008–2010, we collected both published
triploid hybrid lineages in Central Europe and (d) north-western
and unpublished data on the assumed presence of trip-
Europe. Gamete types are shown in circles and underlined in caseof diploid gametes. X and Y indicate female and male sex-
loid P. esculentus in Central Europe and sampled a total
determining factors, respectively.
of 524 specimens from eight populations in Slovakiaand one in the Czech Republic (see Fig. 2 for locations
systems, triploid males substitute the role of parental spe-
and Table 1 for names, coordinates, frog sample size
cies in L-E and R-E systems (G€
unther et al., 1979), turn-
and type of each population). We also sampled twice
ing sperm-dependent hybridogens into independent
(May 2009 and June 2014) in an area studied by Tun-
‘sexually' reproducing units with an evolutionary poten-
ner & Heppich-Tunner (1992) (see ellipse in Fig. 2),
tial (Christiansen & Reyer, 2009).
but in contrast to these authors, there we did not find
In contrast to this pattern, hybrids from Central Eur-
a single polyploid frog in a total of more than 200 indi-
ope are mostly of diploid genomic constitution (Berger
viduals. Frogs were hand-collected at night and kept
et al., 1988; Vorburger, 2001a; Pl€
otner, 2005; Mikulıcek
separated by sexes in spacious plastic containers. They
et al., 2014a). So far, triploids have been reported only
were assigned to taxa (P. lessonae, P. ridibundus and
from two Central European regions and only in the
P. esculentus) according to species-specific morphological
form of LLR males (Tunner & Heppich-Tunner, 1992;
characters (Pl€
otner, 2005). All specimens were mea-
Tunner, 2000; Mikulıcek & Kotlık, 2001; Mikulıcek
sured, photographed and toe clipped. Ploidy levels of
et al., 2014a). In contrast to the well-studied species'
the P. esculentus hybrids were determined by erythro-
north-western range, where triploid hybrids flourish,
cytes' size in field conditions (erythrocytes of triploids
the gamete production pattern and the reproductive
are significantly larger than diploid ones; Berger,
role of LLR males in Central Europe were poorly
1988a; Vinogradov et al., 1990) and later confirmed by
known. It was also not known whether triploids in the
DNA microsatellite analyses in the laboratory. Frogs
two geographical areas originated from the same or dif-
selected for crossing were individually transpondered
ferent hyridization events.
(RFID PIT tag AEG ID-162, Ulm, Germany), separated
Table 1 Population types and number of frogs sampled in each of them (N LL = number of Pelophylax lessonae, N LR = number of diploidPelophylax esculentus, N LLR = number of triploid LLR P. esculentus, N RR = number of Pelophylax ridibundus).
Sajdıkove Humence Sajd
Numbers in brackets give the number of males, females and individuals of unknown sex, respectively; ?? indicates that in Bahno and KozıChrbat, no LR males were caught, although their presence is likely.
by sex and population of origin and transported to the
were achieved following the Berger et al. (1994) proto-
University of Z€
urich. During transport, they were stored
col with slight modifications: To induce ovulation,
in cloth bags containing small pieces of rubber sponge
females were injected with 100 lL per 10 g body mass
and showered daily with fresh water. All frogs survived
of a 20 mg L1 LHRH hormone in Holtfreter solution
the journey. Once in Z€
urich, they were kept separated
(59 mM NaCl, 0.7 mM KCl, 0.9 mM CaCl2, 2.4 mM NaH-
by sexes, released in outdoor cages and fed ad libitum
CO3 and 1.6 mM MgSO4, pH 7.4). Males were anesthe-
with live crickets.
tized in a buffered solution of MS-222 (0.15 g L1)before having one of their testes removed and laceratedinto a Petri dish to obtain the sperm solution. This pro-
Artificial crossing experiments
tocol permits the use of the same sperm solution to fer-
We studied the gamete production pattern of hybrid
tilize eggs from different females and to cross the same
frogs coming from the populations where triploids were
female with different males. After about 15 days, the
found (Table 1), with the exception of Bahno, because
obtained embryos reached free swimming stage (stage
this population was discovered later in the course of
25, Gosner, 1960) and were euthanized using an over-
this study. Instead, we included one population without
dosed MS-222 buffered solution (2 g L1). The off-
Sastın-Straze) where we caught a large num-
spring of a few crosses were used for other experiments
ber of diploid hybrids of both sexes. The original experi-
(Pruvost et al., 2013b), but their genotypic data could
mental design was to cross each hybrid both with other
also be used for our purpose.
hybrids and with at least one specimen of each parentalspecies to determine whether they produce clonal or
recombined gametes. Because some females had a lim-ited number of eggs, the full design could not be
Forty-three hybrids were analysed by flow cytometry to
applied in the populations of
Sajdıkove Humence and
confirm their ploidy level and, if males, to determine
Borovec (see results in Table 2). Based on results of
ploidy level of their sperms. Blood and sperm samples
previous studies using four to eight allozyme markers
were stabilized in buffer (40 mM citric acid trisodium
and crossing experiments with frogs from four Central
salt, 0.25 M saccharose and 5% DMSO) and immedi-
European populations (Tunner, 1980; Tunner & Hepp-
ately frozen at 80 °C (Cunha et al., 2008). Samples of
ich-Tunner, 1992; Mikulıcek & Kotlık, 2001), we tested
both parental species were used as a diploid standard.
with a set of microsatellites a prediction whether dip-
Relative nuclear DNA content was measured using
loid and LLR triploid hybrids produce haploid R and
DAPI fluorochrome applying a commercial kit Cystain
diploid LL gametes, respectively. Artificial fertilizations
two Step High Resolution DNA Staining (Partec GmbH,
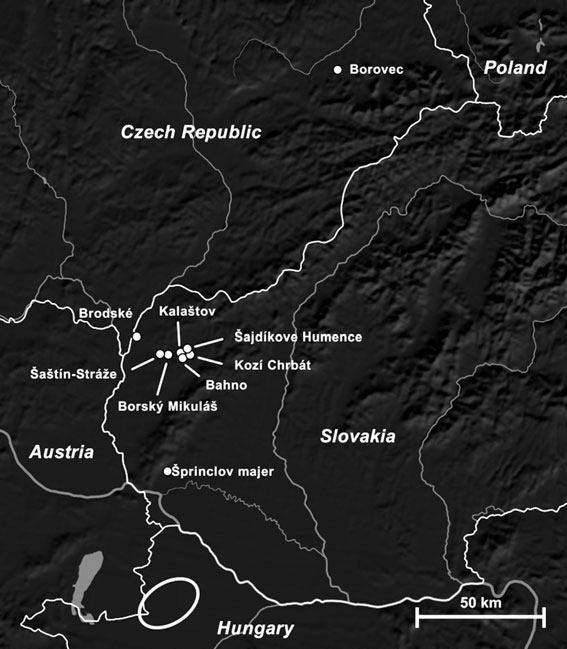
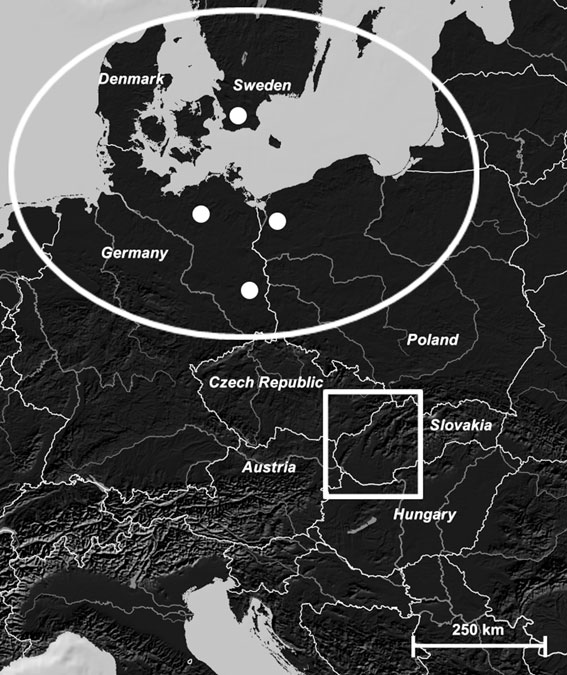
Reproductive strategies in triploid frogs
unster, Germany). Fluorescence intensity of 5000
stained nuclei was measured in Partec PA II flow cy-tometer with a speed 0.5 lL s1. Flow cytometric histo-grams were evaluated using FloMax 2.52 software(Partec GmbH, M€
unster, Germany).
The combination of 18 microsatellite loci was used todetermine and/or confirm the genomic composition ofthe crossed specimens and their offspring, in terms oftaxon and ploidy level to understand the heredity modeof polyploids. To address the evolution of water frogpolyploidy, we used a population genetic approach.
Observation of a low genetic diversity and little geneticdifferentiation within clonally transmitted genomeswould suggest a single rather than a multiple origin ofhemiclones. DNA was extracted from toe or tail tips ofthe adult frogs or tadpoles, respectively, stored in 96%ethanol. The Qiagen BiosprintTM 96 DNA Blood Kit(Qiagen, Venlo, the Netherlands) was used for extrac-tion following supplier's protocol. We used a set of 18microsatellite primer pairs which were run in four pri-mer mixes:
1 Primer Mix 1A – CA1b6, Ga1a19 redesigned (Arioli
et al., 2010), RlCA1b5, RlCA5 (Garner et al., 2000),Rrid064A (Christiansen & Reyer, 2009)
2 Primer Mix 1B – Re2CAGA3 (Arioli et al., 2010),
Res16, Res20 (Zeisset et al., 2000) RlCA2a34 (Chris-tiansen & Reyer, 2009)
3 Primer Mix 2A – ReGA1a23, Rrid169A, Rrid059A
redesigned (Christiansen & Reyer, 2009), Res22(Zeisset et al., 2000), Rrid013A (Hotz et al., 2001)
4 Primer Mix 2B – Re1CAGA10 (Arioli et al., 2010),
RlCA18 (Garner et al., 2000), RlCA1a27, Rrid135A(Christiansen & Reyer, 2009).
Details on PCR protocols are given by Christiansen
(2009) and Christiansen & Reyer (2009). Fragmentlength analysis of the PCR products was run on an ABI3730 Avant capillary sequencer with internal size stan-dard (GeneScan-500 LIZ; Life Technologies EuropeB.V., Zug, Switzerland), and the alleles were scoredwith the GeneMapper software 3.7 (Applied Biosys-tems, Foster City, CA, USA).
We knew from previous studies that three microsatel-
lite loci are species specific for the P. lessonae genome
Fig. 2 (a) Areas of water frog populations with triploid hybrids
(Res20, RlCA1a27 and RlCA18), four are specific for
relevant to this study. The ellipse shows the approximate
P. ridibundus genome (Re2CAGA3, Res22, Rrid169A
major distribution around the Baltic Sea, with four localities
and Rrid135A), and 11 loci amplify in both the L and R
(black dots) for which the north-western European pattern of
genomes (Christiansen, 2005, 2009; Arioli et al., 2010).
gamete production has been documented. The rectangleindicates the Central European area investigated in this study.
(b) Enlarged map of the study area shows locations of the nine
Estimation of null alleles and selection of
investigated populations (black dots) and a previously studied
microsatellite loci
area (ellipse; Tunner & Heppich-Tunner, 1992) with the sameCentral European gamete production pattern as found in our
Because L and R genomes do not recombine in hybrids,
the two genomes were considered separately in the
Table 2 Origin, genotype, sex and individual numbers of the
absence of an allele as evidence for a null allele. We
frogs used in artificial crossing experiments.
then excluded all loci showing an estimated null allelefrequency > 0.2 in any of the populations. This led us
to exclude locus Re1CAGA10 for the L genome,
Genotype Sex Ind. Num.
crosses offspring Gametes
RlCA2a34 for the R genome, and RlCA5 and Res16 for
both genomes from subsequent analyses. We also
Rrid059A redesigned for the L genome and locus
ReGA1a23 for the R genome, because in all samples
they showed only one allele per locus and, thus, pro-
vided no variation for the genetic analysis. This left us
with 8 loci for the L genome and 11 for the R genome:
Res20, RlCA2a34, ReGA1a23, RlCA1a27 and RlCA18 (L
genome); Ga1a19 redesigned, Rrid064, Re2CAGA3,
Res22, Rrid169A, Rrid059A redesigned, Re1CAGA10
and Rrid135A (R genome); and CA1b6, RlCA1b5 and
Rrid013A (for both L and R genomes).
Analysis of genetic diversity and differentiation at
individual and population levels
We calculated the gene diversity, corrected for sample
size, expressed by the expected heterozygosity (H
1978), using the program SPAGeDi 1.3 (Hardy & Veke-
mans, 2002) and the allelic richness (AR) using the
program FStat 2.9.3.2 (Goudet, 2001). Genetic differen-
tiation between populations and genotypes was mea-
sured using FST statistics following the method of Weir
& Cockerham(1984), which is implemented in the pro-
gram SPAGeDi 1.3. The program allows the combina-
tion of multiple ploidy levels in the same analysis.
Concerning genetic diversity, we used two tailed pair-
wise t-tests on the values of He for each locus, to test
N crosses = number of different partners the individual was
the significance of differences between different frog
crossed with; N offspring = number of resulting tadpoles that were
types, independent of their origin, and we used ANOVAs
analysed; and Gametes = gamete type produced by each individual
to look for differences in He between population types.
as deducted from the parents' and the offspring's genotypes. All
Statistical tests were run using the program R 2.15.1
individuals exclusively produced the indicated gamete type.
(http://www.r-project.org/). Differences in AR amonggenomes present in different genotypes were carriedout using two-sided permutation tests implemented in
subsequent genetic analyses. Prior to these steps, we
tested raw data for the presence of null alleles. Nonam-
To test whether R and L genomes present in hybrido-
plifying loci were rerun for PCR two to three times.
genetic hybrids are related to those present in the local
When even then no allele was amplified, we attributed
parental species, Bayesian assignment programs STRUC-
the result to the presence of a null allele, rather than to
TURE 2.3.3 (Pritchard et al., 2000; Falush et al., 2007)
low DNA quality, because this individual DNA ampli-
and BAPS 5.3 (Corander et al., 2003) were applied.
fied for other loci. Potential genotyping errors like stut-
These programs use an iterative approach to assign
tering, allelic dropout or presence of null alleles were
genotypes into K populations without a priori knowl-
tested separately for parental RR and LL taxa using the
edge of the population membership of individuals, min-
program Micro-Checker 2.2.3 (Van Oosterhout et al.,
2004). We estimated frequencies of null alleles with the
disequilibria within populations. Both parental genomes
Brookfield 2 null allele estimator, which treats nonam-
were analysed separately. Models implemented in both
plifications as data and regards them as null homozyg-
programs assume that loci are unlinked and in H-W
equilibrium. These assumptions are unlikely to be met
(Brookfield, 1996). Because this method cannot be
in clonal and hemiclonal hybrid populations because of
applied to the diploid hybrids, we inspected the L and
fixed heterozygosity and linkage of multilocus haplo-
R genomes in hybrids visually and considered the
types. Therefore, we did not infer the most likely
Reproductive strategies in triploid frogs
number of K, that is clusters with H-W and linkage
equilibria. Instead, only fixed K = 2 and K = 3 were
microsatellite alleles, producing a chain of allele sizes
used, assuming hybrids and a parental species (K = 2),
which represent our MLGs. We then compared these
and diploid hybrids, triploid hybrids and a parental spe-
MLGs to find whether they were present in other pop-
cies (K = 3) as the clusters, respectively. Using STUC-
ulations under study. The detected MGLs were named
TURE, admixture and uncorrelated allele models were
after the hemiclone type (L, R, LL), followed by a capi-
applied. The analyses were based on runs of 106 itera-
tal letter attributed in accordance to the descending
tions, following a burn-in period of 100 000 iterations.
overall frequency (e.g. L-A = P. lessonae hemiclone-
A series of ten independent runs for each K was made
A = most frequent L hemiclone). For more details, see
with the same parameters to test the accuracy of
results. In BAPS, a clustering of groups of individualswas run first, followed by an admixture clustering (Cor-
ander & Marttinen, 2006; Corander et al., 2008). Thenumber of iterations that were used to estimate the
Structure of water frog populations (topic I)
admixture coefficients for the individuals, and thenumber of reference individuals from each population,
The genomic composition of the 524 sampled speci-
was 200. The number of iterations that were used to
mens analysed with 18 microsatellite loci showed that
estimate the admixture for the reference individuals
all but one population contained two or three water
was set to 20.
frog genotypes (DNA microsatellite data are given inData S1). The exception was the
Sprinclov majer local-
ity, where we found only P. ridibundus. The exact num-
Analysis of hemiclonal diversity
bers, including sex ratio for each genotype, are listed in
As coined by Vrijenhoek (1979), the term ‘hemiclone'
Table 1. Based on their genotype composition, the eight
refers to the clonally transmitted haploid genome,
populations were classified into four types (1–4), each
which in our case can be of the L or R type. We deter-
represented by two localities. The four populations of
mined them by a multilocus genotype (MLG), defined
types 1 and 2 contained only diploid hybrids, whereas
by the identical combination of alleles found in our mi-
the populations of types 3 and 4 were inhabited also by
crosatellite analysis. The same MLG can be, however,
triploids. In all but two populations, diploid genotypes
found also in two or more unrelated sexual individuals
were always found in both sexes with a male bias in
when discrimination power of used molecular markers
the parental species LL and RR and a female bias in LR
is low. Therefore, we first calculated two statistics,
hybrids (see totals in Table 1). The two exceptions were
probability of identity (PI) and probability of identity
Bahno and Kozı Chrbat where no LR males were
siblings (PIsibs), that estimate the probability that two
caught. In contrast, triploid LLR hybrids in the four
individuals randomly chosen from a population have
populations of types 3 and 4 occurred as males only;
the same MLG on a set of markers (Waits et al., 2001).
LLR females were neither caught during this study
Both statistics were calculated for both parental species
(Table 1) nor found during previous samplings per-
using GenAlEx 6.4 (Peakall & Smouse, 2006). PI and
formed by Mikulıcek et al. (2014a).
2.4 9 104, respectively. PI and PIsibs for P. lessonae
Gamete production (topics II and III)
were 1.6 9 107 and 2.3 9 103, respectively. Thesevalues are reasonably low (cf. Waits et al., 2001), indi-
To identify the heredity mode among hybrids, we per-
cating there is low probability that two P. ridibundus or
formed flow cytometry on sperms of 28 males and
P. lessonae individuals share the same MLG on a set of
genotyped 2216 offspring from 96 crosses through mi-
used microsatellites. Following this calculation, we
crosatellite analyses. Flow cytometric analysis allowed
applied a conservative approach and recognized a hemi-
us to distinguish different ploidy levels among frogs
clone when the same MLG was present in our sample
(2n, 3n and one 4n individual) and between parental
more than three times.
genotypes (RR and LL) (Data S2a). It also allowed dis-
As different hemiclonal gametes may fuse (syngamy)
tinguishing between haploid sperms (produced by
and develop into diploid zygotes on the basis of
parental males and 2n and 4n hybrids) and 2n sperms
hybrid 9 hybrid matings (Hotz et al., 1992), we also
(produced by 3n males) (Data S2b). In all these cases,
searched for possible hemiclonal MLG combinations in
the flow cytometric histograms were clearly nonover-
the individual genomes of the parental species (LL and
lapping. In contrast, overlapping histograms of blood
RR) and in diploid and triploid hybrids (LR and LLR).
samples did not allow distinguishing between genotypes
Because some triploid LLR hybrids may produce also
of diploid hybrids (LR) and parental species, nor was it
diploid (LL) hemiclonal gametes (Tunner & Heppich-
possible to tell whether LLR males produced LL or LR
Tunner, 1992; Mikulıcek & Kotlık, 2001), we tested the
sperms. However, in combination with results from the
data for the presence of LL hemiclones as well. To do
artificial crossing experiment, we unambiguously iden-
tified the gamete production pattern, including for
(AR = 1.625) and LL (AR = 8.125, two-sided
female eggs which cannot be analysed through flow
permutation test, P = 0.0001), and between LLR and
cytometry. All specimens of the two sexual parental
LR (AR = 7.272, P = 0.003), but not between diploid
species used for the crosses acted as normal haploid
hybrids and P. lessonae (P = 0.308). For the P. ridibundus
gamete donors (L in P. lessonae, R in P. ridibundus) with
genome, highly significant differences have been found
chromosome segregation in accordance with the second
Mendel's law. Both sexes of LR hybrids produced hap-
P = 0.009), and between RR and LLR (AR = 3.000,
loid R gametes only. The triploid LLR hybrid males
P = 0.002), but not between diploid and triploid
exclusively produced diploid clonal LL gametes, a pat-
hybrids (P = 0.825).
tern supported by two independent analyses: flow
Global FST values showed significant and substantial
cytometry on sperm (Data S2) and microsatellite analy-
differentiation among populations for both genomes.
ses on parents and offspring from the crossing experi-
The mean FST values were 0.271 for the L genome and
0.114 for the R genome, respectively. For the L
(WFB015-54 from Kozı Chrbat) produced haploid R
sperms and a few diploid cells of unknown genotypic
between LL and LR individuals (FST = 0.021), but very
large differentiation between LLR and both LL and LRindividuals
Table 4). For R genomes, the genetic differentiation
Population genetics (topic IV)
was small between LR and LLR hybrids (FST = 0.019),
Genetic diversity and differentiation
whereas it was large between RR and both LR and LLR
The genetic diversity estimates for the L genomes (HeL)
hybrids (FST = 0.133 and FST = 0.129, respectively).
and for the R genomes (HeR), are presented in Table 3
Pairwise FST values clearly separated the L genomes of
and Data S3. Pooled over all eight populations, gene
triploid LLR hybrids from those of LR and LL individu-
diversity in the P. lessonae genome was significantly
als (Table 5). Hence, the triploids were in their L ge-
lower in LLR triploids (HeL = 0.256) compared to P. les-
nomes genetically not only strongly differentiated from
the parental LL individuals, but also from the diploid
LR hybrids in syntopic populations. In contrast, there
t(7) = 2.364, P = 0.011). No significant differences in
was little to only moderate genetic differentiation in L
HeL were found between the P. lessonae genome of dip-
genomes of parental LL individuals and diploid LR
loids and the parental species (t
2.364, P = 0.111).
hybrids in all population types. The only exception was
For the P. ridibundus genome, significant differences in
represented by diploid LR hybrids from the Czech pop-
ulation of Borovec, whose L genome was distinct from
0.631) and both LR (HeR
all other populations (Table 5).
P = 0.001) and LLR hybrids (HeR = 0.413, t(10) = 0.228,
Concerning the R genomes, parental RR individuals
P = 0.006), but not between diploid and triploid
from different localities revealed mostly little to large
0.228, P = 0.996). Significant differences
genetic differentiation between themselves and mostly
in gene diversity between different population types
moderate to large differentiation between them and
were not observed.
both diploid and triploid hybrids from all population
In terms of AR, highly significant differences in
types (Table 5). In contrast, there was only little to
the P. lessonae genome have been found between
moderate differentiation among R genomes of both
Table 3 Gene diversity (He) corrected for sample size (Nei, 1978) for Pelophylax lessonae genomes (HL) and Pelophylax ridibundus genomes(HR) in the different frog genotypes (LL, LLR, LR, RR). Sample size is given in brackets.
PT2 (LL + LR + RR)
PT2 (LL + LR + RR)
PT4 (LLR + LR + RR)
Sajdıkove Humence
PT4 (LLR + LR + RR)
Reproductive strategies in triploid frogs
hybrid types (LR and LLR) from all populations. Again,
a separate cluster with high probability. Structuring in
the population in Borovec stood out, because both the
the P. ridibundus genome between the genotypes RR,
diploid and the triploid hybrids genetically differed in
LR and LLR was not so straightforward (Fig. 3b). More
their R genome from parental RR individuals and
than 90% of P. ridibundus individuals were assigned to
hybrids found elsewhere.
the cluster 1 regardless of the number of expected K,
The results of the two Bayesian programs were
whereas 64% of both diploid and triploid hybrids were
concordant and revealed substantial structuring in the
assigned to cluster 2 (including almost all diploid LR
P. lessonae genome (Fig. 3a). Triploid hybrids on the
from Borovec); remaining hybrids were assigned to the
one hand and diploid hybrids and P. lessonae on
cluster 1 (assuming K = 2) and clusters 1 or 3 (assum-
the other were unequivocally assigned to two separate
ing K = 3). Only few individuals were assigned to more
clusters assuming K = 2. Assuming K = 3, Bayesian
than one cluster revealing admixture across analyses.
clustering was very similar, with the exception of LRhybrids from Borovec – most of them were assigned to
Analysis of hemiclonal diversityWith respect to the R genomes present in hybrid indi-viduals, we detected a total of 14 hemiclones with dif-
Table 4 Pairwise FST values for L (below the diagonal) and R
ferent relative frequencies among populations (Table 6
(above the diagonal) genomes between the hybrid types listed in
and Data S4). In the Czech population of Borovec, we
the left column (LLR, LR) and the hybrids and parental species
found only a single hemiclone (R-B), whereas the
shown in the top horizontal row (LL, LLR, LR, RR) and parental
Slovakian populations contained multiple R hemi-
species (LL, RR), pooled over all populations.
clones, ranging from four in Brodske to eight in
Straze (Table 6). Hemiclone R-A occurred in all fourpopulation types (PT1-4); five (R-F, R-H, R-K, R-L and
R-N) occurred only in populations with parental LL
frogs (PT1 and/or PT2); and three hemiclones (R-B, R-
Table 5 Pairwise FST value comparisons between all genotype-population combinations for L (below the diagonal) and R (above thediagonal) genomes. Darker colours correspond to lower FST values. For abbreviations of population names, see Table 1.
Bors Kala Brod Sast Bahn Kozi
Sajd Boro Bors Kala Brod Sast Bahn Kozi
Sajd Boro Brod Sast Sajd Boro Spri
Bahn LLR .280
very great
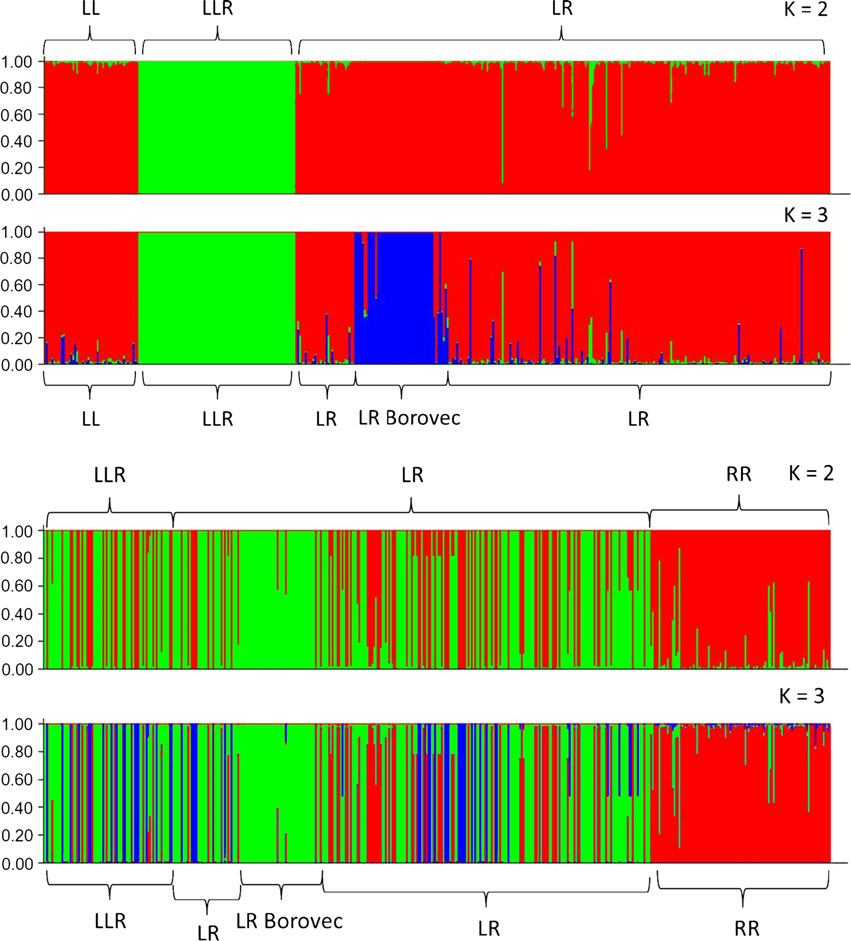
Fig. 3 Structuring in the L genome (a)and the R genome (b) according to aBayesian analysis assuming two (K = 2)and three (K = 3) clusters, respectively.
G, and R-M) were found only in populations where
genomes combined from two hemiclones, namely as a
triploid LLR hybrids are present (PT3 and PT4). The
combination of LL-A hemiclone and one of six R hemi-
remaining four hemiclones (R-C, R-E, R-I and R-J)
clones (Comb-B to G, Table 6). Three LLR males from
were not specific to any population type.
Borovec were composed of LL-B and R-B hemiclones
Concerning the L genome, the number of hemiclones
(Comb-H, Table 6).
was much smaller than within the R genome. Wedetected only a single L hemiclone (L-A) and two LL
(diploid) hemiclones (LL-A and LL-B). L-A occurredonly in diploid hybrids from Borovec but there in a
Population composition and gamete production
very high proportion (38 of 50 sampled LR frogs). Both
(topics I and II)
LL hemiclones were present in all male LLR triploidhybrids (N = 83). One hemiclone (LL-B) was restricted
In the nine sampling sites that we studied in Central
to Borovec, and the other one (LL-A) was present in
Europe, we have identified four population types,
the three Slovak populations (Bahno, Kozı Chrbat and
three where hybrids live in sympatry with one or
Sajdıkove Humence). The two LL hemiclones differed
both parental species and one with hybrids only. Our
by only one allele in their MLG comparison, showing
combined data from flow cytometry, crossing experi-
one dinucleotide repetition difference at the locus
ments, analysis of genetic diversity and gene flow
RlCA18. In Borovec, the locus RlCA18 amplified for
between genotypes show that even in the two appar-
alleles 177 and 181, whereas LLR frogs from Slovakia
ent all-hybrid populations of type 3, the parental spe-
carried alleles 179 and 181. We further found that 35
cies P. lessonae rather than triploid hybrids provide L
of 50 LR hybrids from Borovec likely originated from a
combination of two hemiclones, namely L-A and R-B
hybrids. This is further supported by the FST statistics
(called as Comb-A, Table 6). Triploid LLR individuals in
and clustering of L genomes from LR hybrids with
Kozı Chrbat,
Sajdıkove Humence and Bahno had also
P. lessonae and not with LLR hybrids (Tables 4 and 5),
Reproductive strategies in triploid frogs
Table 6 Multilocus genotypes (MLGs) for L, R and LL hemiclones and their combinations found in the study and their distribution overthe populations.
Hemiclonal MLG name
Distribution of hemiclonal MLGs in populations
17 Sast (17 LR), 13 Kozi (9 LLR, 4 LR),
LR and LLR hybrids
10 Sajd (4 LLR, 6 LR), 6 Bahn (2 LLR, 4 LR),
5 Brod (LR), 3 Bors (LR), 2 Kala (LR)
50 Boro (3 LLR, 47 LR), 2 Bahn (LR),
21 Kozi (15 LLR, 6 LR), 14 Kala (LR),
8 Bahn (1 LLR, 7 LR), 4 Sajd (3LLR, 1 LR)
10 Bors (LR), 8 Sast (LR), 7 Kozi (4 LLR, 3 LR),
6 Bahn (LR), 6 Brod (LR), 5 Sajd (2 LLR, 3 LR),
14 Kozi (10 LLR, 4 LR), 7 Bahn (2 LLR, 5 LR),
6 Sajd (5 LLR, 1 LR), 2 Kala (LR)
18 Sats (LR), 1 Kala (LR)
11 Kozi (8 LLR, 3 LR), 1 Sajd (LLR)
6 Bors (LR), 5 Sast (LR), 1 Kala (LR)
8 Kala (LR), 1 Bahn (LR), 1 Bors (LR)
5 Sast (LR), 3 Brod (LR), 1 Bahn (LR)
6 Sast (LR), 1 Bors (LR)
5 Kozi (LLR), 1 Sajd (LLR)
2 Sast (LR), 1 Bors (LR) 1 Brod (LR)
L hemiclone in LR hybrids,
LL hemiclone in LLR hybrids
52 Kozi (LLR), 20 Sajd (LLR), 5 Bahn (LLR)
Comb. LL+R hemiclones
15 Kozi, 3 Sajd, 1 Bahn (composed of LL-A + R-C)
10 Kozi, 5 Sajd, 2 Bahn (composed of LL-A + R-E)
9 Kozi, 4 Sajd, 2 Bahn (composed of LL-A + R-A)
8 Kozi. 1 Sajd (composed of LL-A + R-G)
4 Kozi, 2 Sajd (composed of LL-A + R-D)
5 Kozi, 1 Sajd (composed of LL-A + R-M)
3 Boro (composed of LL-B + R-B)
Comb L+R hemiclones
35 Boro (composed of L-A + R-B)
Letters (A-N) behind the genomes indicate different hemiclones: ‘single MLG' refers to allele combinations that were found in only one ortwo copies and, hence, were not considered to form a hemiclone. For abbreviations of population names, see Table 1.
as well as by contrasting levels of genetic diversity (He
between RR individuals sampled in different sites
and AR), which is comparable between LR hybrids and
(Table 5; cf. Mikulıcek et al., 2014b). Moreover, the R
P. lessonae but substantially lower in the LL genome of
genome of hybrids reveals lower genetic diversity in
LLR triploids. L genome provisioning through P. lesso-
comparison to P. ridibundus, thus showing that only
nae is characteristic for L-E system (here represented
part of the P. ridibundus individuals contributed to the
by the population type 1), where diploid hybrids clon-
formation of hybridogenetic lineages. Our combined
ally transmit R genomes (see Table 2) and receive L
data show that LLR hybrids exclusively produce diploid
gametes from P. lessonae (Tunner, 1974; Uzzell & Ber-
LL rather than haploid L sperms. Thus, matings
ger, 1975; Graf & Polls Pelaz, 1989). Even where
between LLR males and LR females will result in LLR
parental P. ridibundus individuals exist, as in population
offspring. This raises the question how then diploid LR
types 2 and 4, they do not seem to be the major con-
hybrids are produced and maintained in the four pop-
tributors of R gametes to hybrid progeny. This is indi-
ulations of types 3 and 4. At present, the answer
cated by the fact that genetic differentiation among R
remains open, but we develop three not mutually
genomes (FST, Bayesian analysis) is larger between LR
exclusive hypotheses in Data S5.
hybrids and RR sexuals in the same population than
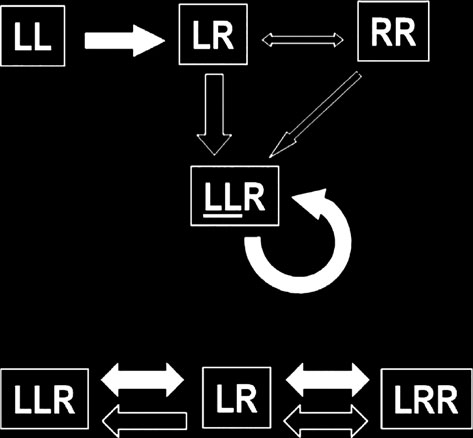
The population data and gamete production modes
(a) Central Europe
are in agreement with an XX–XY sex determinationsystem in which the hemiclonal genome may be cou-pled with either an X or Y haploid set of chromosomes(Graf & Polls Pelaz, 1989). In hybrids, the R genomelikely carries a female determining factor (X), whereasL genomes carry female (X) or male (Y) determiningfactors with equal probability (Berger et al., 1988;Christiansen, 2009). Therefore, in principle, when thediploid LL sperms of LLR males fertilize haploid R eggsof LR females, only LLR males (LYL?RX) will be pro-duced (Fig. 1c). In contrast, diploid LR hybrids come inboth sexes, but with an excess of females because LR
(b) North-western Europe
males sire daughters only (cf. Fig. 1b). This female biasseems to be particularly extreme in the PannonianBasin to which all but one (Borovec) study populationbelongs. In this basin, male proportions as low as 3%
Fig. 4 Heredity pathways of L genomes (black arrows) and R
have been found (Tunner & Dobrowsky, 1976; Berger
genomes (white arrows) between different genotypes in
et al., 1988; Gubanyi & Creemers, 1994; Mikulıcek &
populations with triploid hybrids from (a) Central Europe (this
Kotlık, 2001). Therefore, the virtual absence of LR
study) and (b) north-western Europe (simplified from Som &
males in Bahno and Kozı Chrbat may be the result of a
Reyer, 2006; Christiansen, 2009).
sampling bias that is due to low abundance. In contrast,the lack of LLR females in Central Europe is to beexpected from the gamete production pattern (Fig. 1c).
produce LLR offspring of both sexes (LYL?RX, LXLXRX)(Fig. 1d).
Second, LLR males in Central Europe sexually para-
The reproductive role of triploid males in Central
sitize LR females for self-reproduction, because the
and north-western Europe (topic III)
resulting progeny are 100% LLR males which will
In none of the nine Central European populations
exclude the R genomes at gametogenesis (Fig. 4a).
that we studied in this paper did we find evidence
These diploid LR females, in turn, are also sexual para-
for the type of all-hybrid populations that are typical
sites because for successful reproduction of hybrid off-
for north-western Europe. Even the two populations
spring they require a donor of L gametes, which likely
containing only diploid LR and triploid LLR hybrids
(Fig. 4a). In contrast, in all-hybrid E-E populations
Although both regions share a presence of LLR males,
from the north-western Europe, L and R alleles are
they differ in several aspects which are summarized
passed on between diploid and triploid males and
females (Berger, 1988b; G€
First, sex ratios differ markedly among LLR triploids.
& Reyer, 2006) (Fig. 4b). In these latter populations,
Because of the XX/XY sex determining mechanism
LLR males are sexual hosts for the diploid LR females
described above, fusion of LL sperms from LLR males
and, hence, fulfil the key role that P. lessonae has in L-E
and R eggs from LR females in Central Europe will
systems. Thus, in north-western Europe, triploids help
result in LLR males only (LYL?RX) (Fig. 1c). In contrast,
in stabilizing (all-hybrid) populations by substituting
fusion of haploid L sperms from LLR males with diploid
the role of sexual species, whereas in Central Europe,
LR eggs from LR females in north-western Europe will
they do not.
Table 7 Differences between water frog populations with triploid LLR individuals in north-western and Central Europe; occasionaldeviations from this pattern do occur, based on this study and data from Christiansen (2009) Christiansen & Reyer (2009) and Jakob et al.
(2010).
Features of LLR frogs
North Western Europe
Gamete production
Clonal LL gametes
Recombined L gametes
Origin of triploids
LL sperms from LLR males 9 R eggs from LR females
L sperms from LLR males 9 LR eggs from LR females
Reproductive role
Self-reproduction sexual parasites
L gamete donor substitute sexual hosts
Reproductive strategies in triploid frogs
present, we do not know whether the two geographic
The origin of male polyploidy in Central Europe
regions represent single or multiple hybrid origin.
However, our results strongly suggest that partheno-
Our results from microsatellite genotyping, crossing
genetic animals (sensu lato) originating from the same
experiments and population genetic statistics consis-
parental species and carrying even the same genotype
tently indicate that LLR from all populations were very
(here LLR) can independently develop various repro-
similar with respect to the multilocus genotype (MLG)
ductive roles. These findings place hybrid water frogs
of their two lessonae genomes: in the three Slovakian
in contrast to most other vertebrate parthenogenetic
populations, the MLG was identical, and in the Czech
systems. For example, all taxa of parthenogenetic rep-
population of Borovec (130 km apart), it differed by
tiles are virtually constrained into a single reproduc-
only a single allele mutation at the locus RlCA18. We
therefore believe that LL hemiclones represent a single
represents a genetic copy of the mother (see a list of
clonal lineage which diversified by mutation after
taxa in Kearney et al., 2009). Similarly, most parthe-
nogenetic fish (either diploid or polyploid) show a
The geographic origin of this LL hemiclonal lineage,
uniform reproductive system, for example in the
however, remains puzzling. Given the high genetic dif-
genus Cobitis, Poecilia, Poeciliopsis and others (Lamatsch
ferentiation in L genomes between the LLR triploids
and the group of Slovakian sexual LL and hybrid LR
S. alburnoides complex produce eggs of various ploidies
frogs, the origin is unlikely to have been in situ, at least
within a single genotype and individual, their role in
not in a recent time. On the other hand, the LL hemi-
a mating system is rather complex than contrasting
clone of triploid males is genetically more similar to the
(Alves et al., 2001). Fertile diploid and triploid hybrid
L genome of diploid hybrids in Borovec than to the L
males in Squalius maintain only clonal spermatogene-
genome of diploid hybrids in Slovakia. Therefore, we
sis, whereas tetraploids produce one type of meiotic
suggest that the LL hemiclonal lineage might have orig-
sperms (Collares-Pereira et al., 2013). Therefore, a
inated somewhere in the area of Borovec, a sample site
demonstration of contrasting roles in reproduction of
situated in the proximity of the European watershed of
a single genotype in vertebrate parthenogens in gen-
the Baltic Sea, the North Sea and the Black Sea. Subse-
eral, and in male sex in particular (i.e. to be a donor
quently, it may have spread southerly through the
of gametes vs. to be a sexual parasite as we evi-
Danubian Basin. The origin of the haploid L hemiclone
denced in LLR triploids), gives an example of a new
found in LR hybrids in Borovec remains unclear,
significant evolutionary potential (reproductive plastic-
because we were not able to recognize its donor in the
ity) in animals with nonsexual reproduction. The
present data also open research questions for future
The presence of several R hemiclones in the Slovak
studies, namely how these triploid male lineages with
populations suggests their multiple origins. This pattern
different inheritance modes evolutionarily affect the
has also been documented for other populations of
dynamics of hybrid populations and what happens in
water frogs (e.g. Tunner, 1974; Uzzell & Berger, 1975;
a contact zone between the two geographic regions'
Hotz et al., 2008). Instead of a scenario of ongoing pri-
populations where the two lineages may meet in the
mary hybridizations between P. lessonae and P. ridibun-
same population.
dus, we suppose the existence of several R hemiclonesto be explained rather by past than current primary
hybridization events. If primary hybridization wasongoing and common, then we would expect low
We thank A. Hoffman, D. Hollinger, M. Kautman and
genetic differentiation in R genome between sexual
M. Konvicka for help in collecting frogs, S. R€
(RR) and hybrid (LR) genomes, i.e. primary hybridiza-
ger for assistance in the laboratory, S.
tion should tend to decrease genetic differentiation
with flow cytometry analysis and S. Rauch and Y. Willi
between sexual and hemiclonal genomes. Contrary to
for helpful input on microsatellite analyses. Specimens
this expectation, we have found substantial genetic dif-
involved in the crosses are lodged in the European
ferentiation (i.e. low gene flow rate) between both
Water Frogs Collection of the Zoological Museum of
genomes, corroborating results based on AFLP markers
the University of Z€urich. Sampling was performed
(Mikulıcek et al., 2014b).
under the permissions of the Ministry of Environmentof the Slovak Republic No. 6846/06-3.1 and 9303/2009-2.1 and of the Nature Conservation Agency of the
General evolutionary implications
Hybrid water frog triploids in north-western Europe
gratefully acknowledge financial support through Grant
and in the Central European area represent indepen-
No. 383911 provided by the Charles University Grant
Agency (GA UK) and support No. RVO 67985904 by
characterized by contrasting inheritance modes. At
the Academy of Sciences of the Czech Republic to L.
Choleva, and Grant No. 3100A0-120225/1 by the Swiss
Corander, J., Waldmann, P. & Sillanp€a€a, M.J. 2003. Bayesian
National Science Foundation to H.-U. Reyer.
analysis of genetic differentiation between populations.
Genetics 163: 367–374.
Corander, J., Marttinen, P., Siren, J. & Tang, J. 2008.
Enhanced Bayesian modelling in BAPS software for learn-ing genetic structures of populations. BMC Bioinformatics 9:
Alves, M.J., Coelho, M.M. & Collares-Pereira, M.J. 2001. Evo-
lution in action through hybridisation and polyploidy in an
Cunha, C., Doadrio, I. & Coelho, M.M. 2008. Speciation
Iberian freshwater fish: a genetic review. Genetica 111:
towards tetraploidization after intermediate processes of
non-sexual reproduction. Philos. Trans. R. Soc. Lond. B Biol.
Arioli, M., Jakob, C. & Reyer, H.-U. 2010. Genetic diversity in
Sci. 363: 2921–2929.
water frog hybrids (Pelophylax esculentus) varies with popula-
Falush, D., Stephens, M. & Pritchard, J.K. 2007. Inference of
tion structure and geographic location. Mol. Ecol. 19:
population structure using multilocus genotype data: domi-
nant markers and null alleles. Mol. Ecol. Notes 7: 574–578.
Avise, J.C. 2008. Clonality – The Genetics, Ecology, and Evolution
Garner, T.W.J., Gautschi, B., R€
othlisberger, S. & Reyer, H.-U.
of Sexual Abstinence in Vertebrate Animals. Oxford University
2000. A set of CA repeat microsatellite markers derived from
Press, New York, NY.
the pool frog, Rana lessonae. Mol. Ecol. 9: 2173–2175.
Bengtsson, B.O. 2009. Asex and evolution: a very large-scale
Gosner, K.L. 1960. A simplified table for staging anuran
overview. In: Lost Sex. The Evolutionary Biology of Parthenogen-
embryos and larvae with note on identification. Herpetologica
on, K. Martens & van Dijk P., eds), pp. 1–19.
16: 183–190.
Springer, Dordrecht, the Netherlands.
Goudet, J. 2001. FSTAT, a program to estimate and test gene
Berger, L. 1988a. Principles of studies of European water frogs.
diversities and fixation indices (2.9.3.2.). http://www2.unil.
Acta Zool. Cracov. 31: 563–580.
Graf, J.D. & Polls Pelaz, M. 1989. Evolutionary genetics of the
persisting in agrocenoses of central Poland (Amphibia, Sali-
Rana esculenta complex. In: Evolution and Ecology of Unisexual
entia, Ranidae). Proc. Acad. Nat. Sci. Philadelphia 140:
Vertebrates, Vol. 466 (R.M. Dawley & J.P. Bogart, eds), pp.
289–301. New York State University Museum Bulletin,
Berger, L., Uzzell, T. & Hotz, H. 1988. Sex determination and
sex-ratios in Western Palearctic water frogs – XX and XY
Gubanyi, A. & Creemers, R. 1994. Population structure of
female hybrids in the Pannonian Basin. Proc. Acad. Nat. Sci.
water frogs in a flood plain of the river Danube (Szigetk€
Philadelphia 140: 220–239.
in Hungary. Zool. Pol. 39: 441–445.
Berger, L., Rybacki, M. & Hotz, H. 1994. Artificial fertilization
Guex, G.-D., Hotz, H. & Demlitsch, R.D. 2002. Deleterious
of water frogs. Amphib.-Reptil. 15: 408–413.
alleles and differential viability in progeny of natural hemicl-
Brookfield, J.F.Y. 1996. A simple new method for estimating
onal frogs. Evolution 56: 1036–1044.
null allele frequency from heterozygote deficiency. Mol. Ecol.
unther, R. 1975. Zum nat€
urlichen Vorkommen und zur Mor-
5: 453–455.
phologie triploider Teichfr€
osche "Rana esculenta", L., in der
Choleva, L., Apostolou, A., Rab, P. & Janko, K. 2008. Making
DDR (Anura, Ranidae). Mitt. Zool. Mus. Berlin 51: 145–158.
it on their own: sperm-dependent hybrid fishes (Cobitis)
unther, R. & Pl€
otner, J. 1990. Mating pattern in pure hybrid
switch the sexual hosts and expand beyond the ranges of
populations of water frogs, Rana kl. esculenta (Anura, Rani-
their original sperm donors. Philos. Trans. R. Soc. Lond. B Biol.
dae). Alytes 8: 90–98.
Sci. 363: 2911–2919.
unther, R., Uzzell, T. & Berger, L. 1979. Inheritance patterns
Choleva, L., Janko, K., De Gelas, K., Bohlen, J., Slechtova, V.,
in triploid Rana "esculenta" (Amphibia, Salientia). Mitt. Zool.
Rabova, M. et al. 2012. Synthesis of clonality and polyploidy
Mus. Berlin 55: 35–57.
in vertebrate animals by hybridization between two sexual
Hardy, O.J. & Vekemans, X. 2002. SPAGeDi: a versatile com-
species. Evolution 66: 2191–2203.
puter program to analyse spatial genetic structure at the
Christiansen, D.G. 2005. A microsatellite-based method for
individual or population levels. Mol. Ecol. Notes 2: 618–620.
genotyping diploid and triploid water frogs of the Rana escul-
Hotz, H., Beerli, P. & Spolsky, C. 1992. Mitochondrial-DNA
enta hybrid complex. Mol. Ecol. Notes 5: 190–193.
reveals formation of nonhybrid frogs by natural matings
Christiansen, D.G. 2009. Gamete types, sex determination and
between hemiclonal hybrids. Mol. Biol. Evol. 9: 610–620.
stable equilibria of all-hybrid populations of diploid and trip-
Hotz, H., Uzzell, T., Guex, G.-D., Alpers, D., Semlitsch, R.D. &
loid edible frogs (Pelophylax esculentus). BMC Evol. Biol. 9: 135.
Beerli, P. 2001. Microsatellites: a tool for evolutionary
Christiansen, D.G. & Reyer, H.-U. 2009. From clonal to sexual
genetic studies of western Palearctic water frogs. Zoosyst. Evol.
hybrids: genetic recombination via triploids in all-hybrid
77: 43–50.
populations of water frogs. Evolution 63: 1754–1768.
Hotz, H., Guex, G.-D., Beerli, P., Semlitsch, R.D. & Pruvost,
Collares-Pereira, M.J., Matos, I., Morgado-Santos, M. & Coel-
N.B.M. 2008. Hemiclone diversity in the hybridogenetic frog
ho, M.M. 2013. Natural pathways towards polyploidy in ani-
Rana esculenta outside the area of clone formation: the view
mals: the Squalius alburnoides fish complex as a model
from protein electrophoresis. J. Zool. Syst. Evol. Res. 46:
system to study genome size and genome reorganization in
polyploids. Cytogent. Genome Res. 140: 97–116.
Jakob, C., Arioli, M. & Reyer, H.-U. 2010. Ploidy composition
Corander, J. & Marttinen, P. 2006. Bayesian identification of
in all-hybrid frog populations in relation to ecological condi-
admixture events using multi-locus molecular markers. Mol.
tions. Evol. Ecol. Res. 12: 633–652.
Ecol. 15: 2833–2843.
Reproductive strategies in triploid frogs
Janko, K., Kotusz, J., De Gelas, K., Slechtova, V., Opoldusova,
Pruvost, N.B.M., Hollinger, D. & Reyer, H.-U. 2013b. Geno-
Z., Drozd, P. et al. 2012. Dynamic formation of asexual dip-
type-temperature interactions on larval performance shape
loid and polyploid lineages: multilocus analysis of Cobitis
population structure in hybridogenetic water frogs (Pelophy-
reveals the mechanisms maintaining the diversity of clones.
lax esculentus complex). Funct. Ecol. 27: 459–471.
PLoS One 7: e45384.
Rybacki, M. & Berger, L. 2001. Types of water frog populations
Kearney, M., Fujita, M.K. & Ridenour, J. 2009. Lost sex in the
(Rana esculenta complex) in Poland. Mitt. Mus. Nat. Berlin,
reptiles: constraints and correlations. In: Lost Sex. The Evolu-
Zool. Reihe 77: 51–77.
tionary Biology of Parthenogenesis (I. Sch€
on, K. Martens & van
Schmeller, D.S., Seitz, A., Crivelli, A. & Veith, M. 2005. Cross-
Dijk P., eds), pp. 447–474. Springer, Heidelberg.
ing species' range borders: interspecies gene exchange medi-
Lamatsch, D.K. & St€
ock, M. 2009. Sperm-dependent partheno-
ated by hybridogenesis. Proc. Biol. Sci. 272: 1625–1631.
genesis and hybridogenesis in teleost fishes. In: Lost Sex. The
Schmidt, D.J., Bond, N.R., Adams, M. & Hughes, J.M. 2011.
Evolutionary Biology of Parthenogenesis (I. Sch€
Cytonuclear evidence for hybridogenetic reproduction in
van Dijk P., eds), pp. 399–432. Springer, Heidelberg.
natural populations of the Australian carp gudgeon (Hypsel-
Maynard-Smith, J. 1992. Age and the unisexual lineage. Nat-
eotris: Eleotridae). Mol. Ecol. 20: 3367–3380.
ure 356: 661–662.
on, I., Martens, K. & van Dijk, P., eds. 2009. Lost Sex. The
Mikulıcek, P. & Kotlık, P. 2001. Two water frog populations
Evolutionary Biology of Parthenogenesis. Springer, Heidelberg.
from western Slovakia consisting of diploid females and dip-
Sinclair, E.A., Pramuk, J.B., Bezy, R.L., Crandall, K.A. & Sites,
loid and triploid males of the hybridogenetic Rana esculenta
J.W. Jr 2010. DNA evidence for nonhybrid origins of parthe-
(Anura, Ranidae). Mitt. Zool. Mus. Berlin 77: 59–64.
nogenesis in natural populations of vertebrates. Evolution 64:
Mikulıcek, P., Kautman, M., Kautman, J. & Pruvost, N.B.M.
2014b. Mode of hybridogenesis and habitat preferences
Som, C. & Reyer, H.-U. 2006. Demography and evolution of
influence population composition of water frogs (Pelophylax
pure hybridogenetic frog (Rana esculenta) populations. Evol.
esculentus complex, Anura: Ranidae) in a region of sympatric
Ecol. Res. 8: 1235–1248.
occurrence (western Slovakia). J. Zool. Syst. Evol. Res. doi: 10.
Stenberg, P. & Saura, A. 2009. Cytology of asexual animals.
In: Lost Sex. The Evolutionary Biology of Parthenogenesis (I.
Mikulıcek, P., Kautman, M., Demovic, B. & Janko, K. 2014a.
on, K. Martens, P. van Dijk, eds), pp. 63–74. Springer,
When a clonal genome finds its way back to a sexual species:
evidence from ongoing but rare introgression in the hybrido-
ock, M., Lampert, K.P., Moeller, D., Schlupp, I. & Schartl,
genetic water frog complex. J. Evol. Biol. 27: 628–642.
M. 2010a. Monophyletic origin of multiple clonal lineages in
Moritz, C., Brown, W.M., Densmore, L.D., Wright, J.W.,
an asexual fish (Poecilia formosa). Mol. Ecol. 19: 5204–5215.
Vyas, D., Donnellan, S. et al. 1989. Genetic diversity and
ock, M., Ustinova, J., Lamatsch, D.K., Schartl, M., Perrin, N.
the dynamics of hybrid parthenogenesis in Cnemidophorus
& Moritz, C. 2010b. A vertebrate reproductive system
(Teiidae) and Heteronotia (Gekkonidae). In: Ecology and Evo-
involving three ploidy levels: hybrid origin of triploids in a
lution of Unisexual Vertebrates, Vol. 466 (R.M. Dawley & J.P.
contact zone of diploid and tetraploid Palearctic green toads
Bogart, eds), pp. 87–112. New York State Museum, Albany,
(Bufo viridis subgroup). Evolution 64: 944–959.
ock, M., Ustinova, J., Betto-Colliard, C., Schartl, M., Moritz,
Neaves, W.B. & Baumann, P. 2011. Unisexual reproduction
C. & Perrin, N. 2012. Simultaneous Mendelian and clonal
among vertebrates. Trends Genet. 27: 81–88.
genome transmission in a sexually reproducing, all-triploid
Nei, M. 1978. Estimation of average heterozygosity and genetic
vertebrate. Proc. Biol. Sci. 279: 1293–1299.
distance for small number of individuals. Genetics 89:
Suomalainen, E., Saura, A. & Lokki, J. 1987. Cytology and Evo-
lution in Parthenogenesis. CRC Press, Boca Raton, FL.
Otto, S.P. & Lenormand, T. 2002. Resolving the paradox of sex
Tunner, H.G. 1974. Die klonale Struktur einer Wasserfrosch-
and recombination. Nat. Rev. Genet. 3: 252–261.
population. Z. Zool. Syst. Evol. 12: 309–314.
Parker, E.D. Jr & Niklasson, M. 1999. Genetic structure and
Tunner, H.G. 1980. Kreuzungsexperimente mit Wasserfr€
evolution in parthenogenetic animals. In: Evolutionary Genet-
osterreichischen und polnischen Mischpopulationen
ics: from Molecules to Morphology (R.S. Singh, C.B. Krimbas,
(Rana lessonae + Rana esculenta). Z. Zool. Syst. Evol. 18: 257–297.
eds), pp. 456–474. Cambridge University Press, Cambridge.
Tunner, H.G. 2000. Evidence for genomic imprinting in uni-
Peakall, R. & Smouse, P.E. 2006. GENALEX 6: genetic analysis
sexual triploid hybrid frogs. Amphib.-Reptil 21: 135–141.
in Excel. Population genetic software for teaching and
Tunner, H.G. & Dobrowsky, M.T. 1976. Morphological, sero-
research. Mol. Ecol. Notes 6: 288–295.
logical and enzymological differentiation of Rana lessonae and
otner, J. 2005. Die westpal€aarktischen Wasserfr€osche. Laurenti-
hybridogenetic Rana esculenta from Seewinkel and Neusie-
Verlag, Bielefeld.
dlersee (Austria, Burgenland). Zool. Anz. 197: 6–22.
Polls Pelaz, M. 1994. Modes of gametogenesis among kleptons
Tunner, H.G. & Heppich-Tunner, S. 1992. A new population
of the hybridogenetic water frog complex: an evolutionary
system of water frogs discovered in Hungary. In: Proc. 6th
synthesis. Zool. Pol. 39: 123–128.
Ord. Gen. Meet. Soc. Europ. Her. pp. 453–460. 19–23 August
Pritchard, J.K., Stephens, M. & Donnelly, P. 2000. Inference of
1991. Budapest, Hungary.
population structure using multilocus genotype data. Genetics
Uzzell, T. & Berger, L. 1975. Electrophoretic phenotypes of Rana
155: 945–959.
ridibunda, Rana lessonae, and their hybridogenetic associate,
Pruvost, N.B.M., Hoffmann, A. & Reyer, H.-U. 2013a. Gamete
Rana esculenta. Proc. Acad. Nat. Sci. Philadelphia 127: 13–24.
production patterns, ploidy, and population genetics reveal
Uzzell, T., Berger, L. & G€
unther, R. 1975. Diploid and triploid
evolutionary significant units in hybrid water frogs (Pelophy-
progeny from a diploid female of Rana esculenta (Amphibia
lax esculentus). Ecol. Evol. 3: 2933–2946.
Salientia). Proc. Acad. Nat. Sci. Philadelphia 127: 81–91.
Van Oosterhout, C., Hutchinson, W.F., Wills, D.P.M. & Ship-
Zeisset, I., Rowe, G. & Beebee, T.J.C. 2000. Polymerase chain
ley, P. 2004. Microchecker: software for identifying and cor-
reaction primers for microsatellite loci in the north European
recting genotyping errors in microsatellite data. Mol. Ecol.
water frogs Rana ridibunda and R. lessonae. Mol. Ecol. 9:
Notes 4: 535–538.
Vinogradov, A.E., Borkin, L.J., G€unther, R. & Rosanov, J.M.
1990. Genome elimination in diploid and triploid Rana escul-enta males – Cytological evidence from DNA flow cytometry.
Supporting information
Genome 33: 619–627.
Additional Supporting Information may be found in the
Vorburger, C. 2001a. Fixation of deleterious mutations in clo-
online version of this article:
nal lineages: evidence from hybridogenetic frogs. Evolution55
Data S1 DNA microsatelitte data file for the 16 loci
: 2319–2332.
Vorburger, C. 2001b. Non-hybrid offspring from matings
used in the study (RlCA5 and Res16 were not used for
between hemiclonal hybrid water frogs suggest occasional
any of the analyses).
recombination between clonal genomes. Ecol. Lett. 4: 628–636.
Data S2 Genome sizes measured by flow cytometry
Vrijenhoek, R. 1979. Factors affecting clonal diversity and
based on blood (b) and sperm (s) samples.
coexistence. Am. Zool. 19: 787–797.
Data S3 Gene diversity (He) corrected for sample size
Vrijenhoek, R.C. 1989. Genetic and ecological constraints on
(Nei, 1978) for P. lessonae and P. ridibundus genomes in
the origins and establishment of unisexual vertebrates. In:
sexual and hybrid genotypes sampled in populations.
Evolution and Ecology of Unisexual Vertebrates, Vol. 466 (R.M.
Data S4 Hemiclonal and single multilocus genotypes
Dawley & J.P. Bogart, eds), pp. 24–31. New York State Uni-
(MLGs) in four genotypes (LL, LR, LLR and RR) from
versity Museum Bulletin, Albany, NY.
nine study populations (Pop.).
Vrijenhoek, R.C. 1999. Parthenogenesis and natural clones. In:
Encyclopedia of Reproduction, Vol. 3 (E. Knobil & J.D. Neill,
Three not mutually exclusive hypotheses to
eds), pp. 695–702. Academic Press, San Diego, CA.
explain how diploid LR hybrids are maintained in pop-
Vrijenhoek, R.C., Dawley, R.M., Cole, C.J. & Bogart, J.P. 1989.
ulations types PT3 (LR females and LLR males) and PT4
A list of the known unisexual vertebrates. In: Evolution and
(LR males and females, LLR males and RR males and
Ecology of Unisexual Vertebrates, Vol. 466 (R.M. Dawley & J.P.
Bogart, eds), pp. 19–23. New York State University MuseumBulletin, Albany, NY.
Data deposited at Dryad: doi:10.5061/dryad.hn4tn
Waits, L.P., Luikart, G. & Taberlet, P. 2001. Estimating the
Received 4 December 2013; accepted 17 November 2014
populations: cautions and guidelines. Mol. Ecol. 10: 249–256.
Weir, B.S. & Cockerham, C.C. 1984. Estimating F-statistics for
the analysis of population structure. Evolution 38: 1358–1370.
Source: http://www.uli-reyer.ch/index_htm_files/Pruvost%20et%20al%20(2014).pdf
© Schattauer 2011 Diagnostik und Behandlung des Gestationsdiabetes entsprechend den aktuellsten Leitlinien U. Schäfer-Graf Berliner Diabeteszentrum für Schwangere, Klinik für Gynäkologie & Geburtshilfe, St. Joseph Krankenhaus Keywords Definition Gestationsdiabetes, Insulinresistenz in der Gestational diabetes, insulin resistance in
DER PROTESTE GEGEN DIE IN SALZBURG WIR SOGNS GLEI, WIR WOANS! .4 WOGEGEN WIR KÄMPFEN .6 WOFÜR WIR KÄMPFEN .17 GEGNER_INNEN – WHO IS WHO DER FUNDIS .29 CHRONOLOGIE –PROTESTE IN SALZBURG SEIT 2008 .43 QUELLEN, LINKS, LESETIPPS .46 GLOSSAR .48 ANARCHAFEMINISTISCHES GEBETSBUCH .53