Unifr.ch
Restorative Neurology and Neuroscience 25 (2007) 435–443
Pharmacological modulation oflearning-induced plasticity in human auditorycortex
Christiane M. Thiel
∗Institute of Biology and Environmental Sciences, University of Oldenburg, 26111 Oldenburg, Germany
Abstract.
Purpose: Converging evidence from animals and humans indicate that the primary auditory cortex is continuously
reshaped in an experience-dependent way. Reorganisation in primary auditory cortex can be observed at the level of receptive
fields, topographic maps and brain activations measured with neuroimaging methods. Several neuromodulatory systems were
shown to contribute to such an experience-dependent reorganization.
Methods: This paper reviews evidence addressing the cholinergic, noradrenergic, dopaminergic and serotonergic modulation of
learning-, experience-, and injury-induced plasticity in the auditory cortex.
Results: Regarding learning-induced plasticity in the auditory cortex most studies have investigated the role of the cholinergic
system and shown that ACh is essential for this form of rapid plasticity. Nevertheless there is also evidence that the catecholamines
dopamine and noradrenaline might contribute to learning- and experience-induced changes in the auditory cortex.
Conclusions: I suggest, that the available experimental data on cholinergic and noradrenergic modulation of plasticity offers a
promising basis for potential pharmacological interventions to aid recovery of aural functions.
Keywords: Plasticity, psychopharmacology, auditory cortex, drug, experience
rochemical modulation of plasticity outside the audito-ry cortex, assuming that similar neurochemical mech-
Several studies have highlighted the importance of
anisms may be responsible for promoting plasticity in
neuromodulators, especially the cholinergic system, in
other primary sensory and motor cortices.
regulating learning-induced plasticity in the auditory
An important issue when addressing the pharmaco-
cortex. This review will summarize research on phar-
logical modulation of plasticity is its clinical appli-
macological modulation of plasticity in humans and
cation. The use of pharmacological interventions for
animals. The main focus is on neurochemical mecha-
promoting cognitive or motor functions after brain in-
nisms of rapidly induced plasticity in associative learn-
jury has recently received some attention (Parton et
ing situations, but evidence from studies on training-
al., 2005). One of the most promising approaches has
induced plasticity as well as findings on recovery offunction after injury are also considered. Since the
been the administration of amphetamines for recovery
number of studies is small that specifically address
of motor and language functions after stroke (Martins-
pharmacological modulation of plasticity in the audi-
son and Eksborg, 2004). Even though in the auditory
tory cortex, I will also consider evidence showing neu-
system, evidence from basic research in animals andhumans offers a promising basis for potential pharma-cological interventions, there are currently no clinical
∗Address for correspondence: Institute of Biology and Environ-
applications of this knowledge. One preliminary study
mental Sciences, Faculty V, Carl von Ossietzky University of Olden-burg, 26111 Oldenburg, Germany. Tel.: +441 798 3641; Fax: +441
however suggests that amphetamine might be useful for
798 3848; E-mail:
[email protected].
improving aural rehabilitation and increasing neural ac-
0922-6028/07/$17.00 2007 – IOS Press and the authors. All rights reserved
C.M. Thiel / Pharmacological modulation of learning-induced plasticity in human auditory cortex
tivity in the auditory cortex after cochlear implantation
viously best frequency (Bakin and Weinberger, 1990;
(Tobey et al., 2005).
see however Ohl and Scheich, 2005). The learning-induced changes are acquired rapidly after only a fewpairings of the tone and footshock and have an endur-
2. Plasticity in the auditory cortex
ing effect on the receptive fields of auditory corticalneurons (Weinberger et al., 1993; Edeline et al., 1993).
The processing of auditory information depends not
Frequency receptive fields in primary auditory cortex
only on the physical properties of stimuli but also on
were also shown to be rapidly modified in other learning
prior experience and learning. Response properties of
paradigms such as habituation learning, instrumental
single neurons and neuron populations are continuous-
avoidance learning and frequency discrimination learn-
ly reshaped in an experience-dependent way through-
ing (Condon and Weinberger, 1991; Edeline and Wein-
out life to meet changing behavioural needs.
berger, 1993; Bakin et al., 1996). A complementary
ticity refers to such an experience-related structural
approach is to study learning-induced plasticity by in-
and/or functional neuronal reorganization. Different
vestigating changes in cerebral metabolism (Gonzalez-
techniques have been used to gauge plastic changes
Lima and Scheich, 1986; Poremba et al., 1998) or tono-
in animals and humans. These include the analysis
topic maps (Rutkowski and Weinberger, 2005). These
of receptive fields in single cells, topographic cortical
studies provide evidence for an increased spatial repre-
maps, brain activations measured in metabolic studies
sentation of conditioned stimuli in the auditory cortex.
and lately brain activity measured with neuroimaging
An elegant study by Rutkowski et al. (2005) further
methods in humans (Bakin and Weinberger, 1990; Re-
suggests that the extent of the area tuned to the CS fre-
canzone et al., 1993; Thiel et al., 2002b).
quency is related to the behavioural importance of the
Plasticity encompasses a variety of different con-
cepts, ranging from plastic changes that are inducedrapidly up to modifications observed only at longer time
2.2. Learning-induced plasticity in humans
scales. I will refer to rapidly induced plasticity in as-sociative learning situations as learning-induced plas-
Several neuroimaging studies, using eye-blink and
ticity. Plasticity observed in perceptual learning situ-
aversive conditioning paradigms, have shown learning-
ations, which involves repeated training over several
induced changes in regional cerebral blood flow (rCBF)
days and weeks will be referred to as training-induced
or blood-oxygen-level dependent (BOLD) signal in the
plasticity. Finally injury-induced plasticity relates to
human auditory cortex (Molchan et al., 1994; Schreurs
reorganization evident after damage to the periphery or
et al., 1997; Morris et al., 1998; Thiel et al., 2002a;
central nervous system.
Thiel et al., 2002b). Molchan et al. (1994) and Schreurset al. (1997) provided evidence for increased rCBF
2.1. Learning-induced plasticity in animals
in primary auditory cortex when comparing the pairedpresentation of a tone and an air puff in an eye blink
Learning-induced plasticity within the auditory cor-
conditioning paradigm with explicitly unpaired presen-
tex is often studied in aversive conditioning paradigms
tations of the tone and air puff. This suggests increased
(Edeline, 1999; Weinberger, 2004). In aversive con-
neural activity in the auditory cortex during associa-
ditioning a previously neutral stimulus, such as a
tive learning. Using functional magnetic resonance
tone (conditioned stimulus; CS), acquires significance
imaging (fMRI) and a differential aversive conditioning
through its prediction of a future aversive event, such
paradigm we found evidence for an increase in BOLD
as an electric shock (unconditioned stimulus; US).
signal in the auditory cortex for a tone which was paired
Learning-induced plasticity in such associative learn-
with an aversive event as compared to a tone, which
ing paradigms has been documented with a variety of
was not paired with the aversive event. That is, auditory
different methods and species. Several studies have
stimuli with an acquired relevance induce greater neu-
demonstrated that frequency responsive receptive fields
ral activity in the auditory cortex than stimuli without
in the primary auditory cortex are retuned during aver-
sive conditioning (Bakin and Weinberger, 1990; Wein-
Overall, the findings demonstrate that blood-flow
berger et al., 1993; Edeline et al., 1993). Most stud-
based techniques such as positron emission tomogra-
ies reported a shift of the receptive fields towards the
phy (PET) or fMRI are able to gauge learning-induced
frequency of the CS with reduced responses to the pre-
changes in associative learning paradigms and provide
C.M. Thiel / Pharmacological modulation of learning-induced plasticity in human auditory cortex
valuable evidence regarding mechanisms of plastici-
the cochlea. In humans, one neuroimaging study inves-
ty in the human brain. It should be however empha-
tigated the effects of high frequency hearing loss and
sised that despite some striking similarities between
was able to demonstrate cortical map reorganization in
learning-induced receptive field or map plasticity in
the auditory cortex (Dietrich et al., 2001). In contrast
animals and plasticity seen with neuroimaging meth-
to training-induced reorganization there is however on-
ods in humans, there are differences in the underly-
ly limited evidence for perceptual consequences of a
ing techniques to measure plasticity (e.g. neuronal re-
haemodynamic responses), and experi-
mental protocols (i.e. animal studies mostly comparereceptive fields before and after conditioning has taken
3. Neurochemical modulation of plasticity in
place while neuroimaging studies often assess learning-
induced changes during conditioning). Nevertheless,the fact that both measures of plasticity are sensitive to
3.1. The cholinergic system
cholinergic manipulations (see below) suggests at leastsome commonality.
Basal forebrain cholinergic neurons send projections
to the entire cortical mantle, including the primary au-
2.3. Training- and injury-induced plasticity
ditory cortex (Kamke et al., 2005). A considerableamount of data indicate the importance of the cholin-
Changes in the cortical representation of auditory
ergic basal forebrain in modulating responses of corti-
stimuli have also been found with behavioural train-
cal neurons. Evidence for long lasting changes in neu-
ing or after injury (Irvine et al., 2001). In the audi-
ronal reactivity due to iontophoretical application of
tory cortex of owl monkeys Recanzone et al. (1993)
acetylcholine (ACh) was shown by Krnjevic and Phillis
have shown a reorganization of frequency representa-
(1963a) in anaesthetised animals. Several other animal
tion following several weeks of frequency discrimina-
studies confirmed this modulatory role of basal fore-
tion training which correlated with performance. How-
brain ACh upon responses to visual (Sato et al., 1987),
ever, in cats improvements in frequency discrimination
auditory (Metherate and Ashe, 1991) or somatosenso-
were not accompanied by changes in tonotopic maps
ry (Tremblay et al., 1990) stimulation. The choliner-
(Brown et al., 2004). Several studies in human volun-
gic modulation of neuronal responsiveness in auditory
teers have reported plastic changes in auditory cortex
and visual cortex is blocked by the administration of
activity after successful frequency discrimination train-
atropine, suggesting an effect mediated through mus-
ing or a pitch memory task (Cansino and Williamson,
carinic cholinergic receptors (Sato et al., 1987; Mether-
1997; Menning et al., 2000; Jancke et al., 2001; Gaab et
ate and Ashe, 1991). While the majority of respons-
al., 2006). Note, that both, decreases and increases in
es are facilitated by ACh, a suppression of firing rate
the measured signal were found and it has been shown
has also been found (Sato et al., 1987; Tremblay et al.,
that depending on the training task used, an increased
1990; Metherate and Ashe, 1991). The direction of
or reduced representation is found in the auditory cor-
cortical response modulation can vary depending, for
tex (Guenther et al., 2004). Several other studies, not
example, on the strength of basal forebrain stimulation,
mentioned in further detail here, have investigated con-
the cortical layer of ACh application or the type of neu-
sequences of auditory experience in relation to learning
ron investigated (Metherate and Ashe, 1991; Xiang et
speech sounds (e.g. Callan et al., 2003) or plasticity
al., 1998; Kimura et al., 1999).
in musicians (Pantev et al., 2003). However, in con-
It is widely reported that increases in cortical ACh
trast to learning-induced plasticity observed in associa-
release occur when animals are presented with be-
tive learning situations, auditory experiences associated
haviourally relevant, aversive stimuli (Acquas et al.,
with behavioural training require at least several hours
1996; Thiel et al., 2000). With regard to learning-
of practice to develop and tonotopic reorganizations are
induced plasticity, a striking observation is that audito-
not always observed.
ry cortex receptive field and map plasticity can not on-
Similar changes in tonotopic representations in pri-
ly be induced by pairing a tone with an aversive event
mary auditory cortex are also seen after restricted
but also by pairing the tone with electrical stimulation
cochlear lesions in animals (see Irvine et al., 2000).
of the nucleus basalis (Hars et al., 1993; Bakin and
The changes are evident as expanded representations of
Weinberger, 1996; Kilgard and Merzenich, 1998; Mi-
frequencies represented at loci in the unaffected part of
asnikov et al., 2001) or an iontophoretic administration
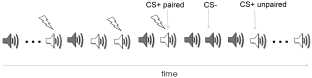
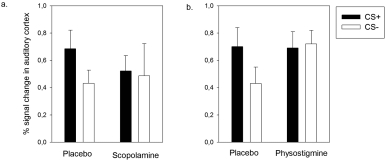
C.M. Thiel / Pharmacological modulation of learning-induced plasticity in human auditory cortex
Fig. 1. Schematic illustration of the aversive conditioning approach used in the fMRI studies.
Fig. 2. Plots of percent signal change (mean and S.E.M.) of two voxels in the auditory cortex illustrating cholinergic modulation of learning-inducedBOLD activity (for full data see [68; 69]) a. Effects of cholinergic blockade with scopolamine in a right auditory cortex voxel showing asignificant group by conditioning interaction (x = 57, y = −15, z = 6) b. Effects of cholinergic enhancement with physostigmine. Activityin a left auditory cortex voxel showing a group by conditioning interaction in the follow up study (x = −63, y = −18, z = 9). Note thatin comparison with placebo, scopolamine reduced activations to the CS+ whereas physostigmine increased activations to the CS−. Reprintedfrom Neurobiology of Learning and Memory, 80, Thiel, Cholinergic modulation of learning and memory in the human brain as detected withfunctional neuroimaging, 234–244, Copyright (2003), with permission from Elsevier".
of ACh (Metherate and Weinberger, 1989). A study
a shock (CS−). A partial reinforcement schedule was
by McLin et al. (2002) further underlines that stimula-
used in which only one half of the CS+ stimuli were
tion of the cholinergic basal forebrain not only induces
paired with the aversive event (CS+ paired) and the
frequency specific neuronal plasticity but also elicits
other half were not (CS+ unpaired). This enabled the
cardiac and respiratory responses to the frequency of
comparison of BOLD activity to the CS+ in the absence
the tone, indicating the induction of behavioural asso-
of the shock with activations to the CS− (see Fig. 1).
ciative memory. The plasticity induced by pairing nu-
In other words, two auditory stimuli are compared, one
cleus basalis stimulation with a tone is blocked by sys-
with acquired significance and one without. Learning-
temic or cortical atropine which suggests that activa-
induced plasticity in this context is defined as a higher
tion of muscarinic ACh receptors is crucial to this form
BOLD signal to the unpaired CS+ as compared to the
of learning-induced plasticity (Bakin and Weinberger,
1996; Miasnikov et al., 2001).
In our first study subjects were given in a between
In order to investigate the role of the cholinergic sys-
group design either placebo or 0.4 mg of the muscarinic
tem in learning-induced plasticity in humans we per-
antagonist scopolamine intravenously to block cholin-
formed two fMRI studies involving a cholinergic drug
ergic function (Thiel et al., 2002b). A reaction time
challenge (Thiel et al., 2002a; Thiel et al., 2002b).
measure indicated behavioural learning in the place-
Learning-induced auditory plasticity was studied in a
bo but not the scopolamine group.
differential conditioning paradigm. We used two tones
group, learning-induced enhancement of the BOLD re-
of different frequency as CS (400 Hz or 1600 Hz). One
sponse in the auditory cortex was evident to the CS+
of these tones was paired with an electric shock to the
but not to the respective CS−. Under scopolamine,
left leg (CS+) whereas the other was never paired with
the enhancement of BOLD activity to the CS+ was
C.M. Thiel / Pharmacological modulation of learning-induced plasticity in human auditory cortex
blocked, suggesting that cholinergic muscarinic recep-
ry cortex (Juliano et al., 1991). These findings are in
tors are involved in these learning-induced responses
contrast with recent evidence by Kamke et al. (2005) in
(see Fig. 2a). The findings provide in vivo evidence that
the auditory cortex that suggests that cholinergic input
learning-induced plasticity in human auditory cortex is
is not required for lesion-induced plasticity. It is cur-
attenuated by blockade of cholinergic neurotransmis-
rently open whether the observed discrepancies are due
to difference between cortical systems or rather differ-
Since a cholinergic blockade was found to impair
ences in experimental design between the studies (see
learning-induced plasticity we aimed to further investi-
Kilgard, 2005 for further discussion).
gate the clinically more relevant question whether an in-crease in cholinergic function would increase plasticity.
3.2. The noradrenergic system
We used the same differential conditioning paradigmand between group design as above but the choli-
Noradrenergic neurons are located in the pontine and
neesterase inhibitor physostigmine to boost choliner-
medullary reticular formation. The main source of the
gic function (Thiel et al., 2002a). Data in the placebo
central noradrenergic innervation is the locus ceruleus,
group showed again an enhanced BOLD response to
which provides widespread projections to the entire
the CS+ as compared to the CS− in the auditory cor-
brain (Drouin and Tassin, 2002). With regard to plas-
tex, indicating learning-induced changes. In contrast
ticity in the auditory system, the work of Edeline and
to our hypothesis however, the physostigmine group
colleagues suggests that the iontophoretic application
did not show any differential activation to the CS+
of noradrenaline primarily decreases evoked responses
vs. CS−. This absence of learning-induced plastic
in the auditory cortex of anaesthetised rats (Manunta
changes was however different from that seen previ-
and Edeline, 1997; Manunta and Edeline, 1998). Fur-
ously with scopolamine. While scopolamine prevent-
ther, pairing a particular tone frequency with a nora-
ed the increased neural activation to the CS+ but did
drenaline application changed frequency tuning curves
not interfere with activity to the CS−, physostigmine
in the auditory cortex, suggesting that noradrenaline
increased activations to the CS− without interfering
modulates learning-induced plasticity. The effect was
with the CS+ (see Fig. 2b). We therefore suggest that
dependent on alpha noradrenergic receptors and was
in healthy human volunteers, cholinergic blockade re-
in most cases evident as a response decrease (Manunta
duces neuronal processing of relevant stimuli, where-
and Edeline, 2004).
as cholinergic stimulation increases processing of ir-
There is ample evidence for a noradrenergic role in
relevant stimuli. Both mechanisms reduce learning-
training-induced and injury-dependent plasticity in the
induced plasticity in the auditory cortex. The effects
motor system. In healthy humans it has been shown
of physostigmine could reflect that cholinergic stimu-
that amphetamine can facilitate plasticity induced by
lation in healthy volunteers may overstimulate an oth-
motor training (Butefisch et al., 2002; Tegenthoff et al.,
erwise perfectly balanced cholinergic system.
2004) and language learning (Breitenstein et al., 2004).
The influence of the cholinergic system on training-
Further, a single dose of the noradrenaline reuptake
induced auditory plasticity has not been investigat-
inhibitor reboxetine improves motor skill acquisition
Training-induced plasticity has however often
and excitability in the motor cortex of healthy volun-
been studied in the motor system, where it has been
teers (Plewnia et al., 2004). These findings underline
shown that changes in functional topography are ob-
the importance of noradrenergic neurotransmission for
served after training voluntary movements (Nudo et
training-induced plasticity, at least in the motor cortex.
al., 1996). Two studies support a cholinergic role in
Regarding potential clinical applications, the com-
training-induced motor plasticity. First, the muscarinic
bination of amphetamines with behavioural training is
antagonist scopolamine is able to attenuate training-
currently one of the most promising pharmacological
induced motor plasticity in humans (Sawaki et al.,
approaches in stroke recovery (Boyeson and Feeney,
Second, animal evidence suggests that le-
1990).1 Amphetamine acts on a variety of neurotrans-
sions of the cholinergic basal forebrain abolish training-
mitter systems including the dopaminergic, noradren-
induced map expansions in the primary motor cortex
ergic and serotonergic system but it has been suggested
(Conner et al., 2005).
With regard to injury-induced plasticity, nucleus
1Note, however, that due to the small number of studies and open
basalis lesions were found to block topographic reor-
safety-issues the routine use of amphetamines is currently not indi-
ganizations after peripheral injury in the somatosenso-
cated for the treatment of stroke.
C.M. Thiel / Pharmacological modulation of learning-induced plasticity in human auditory cortex
that the noradrenergic action is crucial for the recovery-
al., 2001). Even though l-dopa is the precursor of both,
promoting effects after injury (Boyeson and Feeney,
dopamine and noradrenaline, only a small fraction of
1990; see however Breitenstein et al., 2006). In ani-
l-dopa is converted into noradrenaline, suggesting that
mals it was shown that a single dose of amphetamine
recovery-promoting effects of l-dopa may primarily be
can promote motor recovery after motor cortex ablation
due to the dopaminergic action of the drug (Breitenstein
(Feeney et al., 1982). Recovery-promoting effects of
et al., 2006).
amphetamine were also observed after lesions of thevisual and sensorimotor cortices (Feeney and Hovda,
3.4. The serotonergic system
1985; Schmanke and Barth, 1997). These findingsindicate that pharmacological interventions may show
The serotonergic innervation of the cortex originates
similar effects in different sensory and motor cortices
from the brain stems raphe nuclei (Azmitia and Segal,
and that beneficial effects of amphetamine might also
1978). Iontophoretic application of serotonin (5-HT)
be seen after damage to the auditory cortex. Indeed, a
was shown to induce both, inhibition and excitation of
preliminary study by Tobey et al. (2005) in eight pa-
cortical neurons (Krnjevic and Phillis, 1963b). With re-
tients with cochlear implants provides first evidence
gard to learning-induced plasticity in the auditory cor-
that the additional use of d-amphetamine in aural re-
tex in vivo microdialysis data suggest however that 5-
habilitation facilitates speech tracking scores and neu-
HT is rather related to the stress induced by the aversive
ral activity in the auditory cortex in adult cochlear im-
stimulus in conditioning paradigms than to associative
plant users. The study therefore suggests that a nora-
learning (Stark and Scheich, 1997). Even though this
drenergic/dopaminergic intervention may be useful for
would speak against a critical role of 5-HT in learning-
promoting recovery of function in the auditory system.
induced plasticity, there is at least evidence for a sero-tonergic modulation of training-dependent plasticity in
3.3. The dopaminergic system
the motor cortex (Pleger et al., 2004). This findingin healthy volunteers is in line with clinical evidence
The dopaminergic input to the cortex originates in
showing that stimulation of serotonergic function in-
the ventral tegmental area (Moore and Bloom, 1978).
creases functional recovery from motor deficits after
Iontophoretic application of dopamine to cells in the
stroke (Pariente et al., 2001). Further research is need-
cerebral cortex results mostly in a depression of neu-
ed to investigate in more detail the potential role of
ronal activity (Krnjevic and Phillis, 1963b). With re-
serotonin in plasticity in the auditory cortex.
gard to learning-induced plasticity, in vivo microdial-ysis provides evidence for increased dopaminergic ac-tivity in the primary auditory cortex during learning
of tone-shock associations (Stark and Scheich, 1997).
Further, it has been shown that pairing a particular
I have summarised evidence that drugs targeting dif-
tone frequency with stimulation of the ventral tegmen-
ferent neurotransmitter systems are able to modulate
tal area shifts frequency response curves and increases
learning- and experience-induced plasticity and recov-
the spatial representation of the respective frequency
ery of function. Regarding learning-induced plasticity
area in the primary auditory cortex. Since the effects
in the auditory cortex most studies have investigated the
were blocked by D1 and D2 receptor antagonists, the
role of the cholinergic system and shown that ACh is
findings suggest that the neurotransmitter dopamine en-
essential for this form of rapid plasticity. Nevertheless
ables learning-induced plasticity in the auditory cortex
there is also evidence that the catecholamines dopamine
(Kisley and Gerstein, 2001; Bao et al., 2001).
and noradrenaline might contribute to learning- and
Even though most of the evidence on recovery-
experience-induced changes in the auditory cortex and
promoting actions of amphetamine suggests that the
to aural rehabilitation after cochlear implantation.
main action is dependent on noradrenergic neurotrans-
Several questions however deserve further attention:
mission, some data also speaks in favour of a dopamin-
It is currently unknown, whether different neuromodu-
ergic role. First, the recovery-promoting actions of am-
latory systems subserve similar, overlapping functions
phetamine can be blocked by the dopaminergic antag-
in promoting plasticity or whether their action can be
onist haloperidol (Feeney et al., 1982). Second, there
differentiated. For example, even though pairing a tone
is evidence in humans for increased recovery of motor
with electrical stimulation of brain areas containing ei-
functions after l-dopa administration (Scheidtmann et
ther cholinergic or dopaminergic neurons both increase
C.M. Thiel / Pharmacological modulation of learning-induced plasticity in human auditory cortex
the spatial representation of the respective tone in the
Azmitia, E. C., & Segal, M. (1978). An autoradiographic analysis of
primary auditory cortex, other measures, such as the
the differential ascending projections of the dorsal and median
representation of adjacent frequencies or the effects in
raphe nuclei in the rat. J Comp Neurol, 179, 641-667.
the secondary auditory cortex were shown to differ-
Bakin, J. S., South, D. A., & Weinberger, N. M. (1996). Induction of
receptive field plasticity in the auditory cortex of the guinea pig
entiate between a "dopaminergic" VTA and "cholin-
during instrumental avoidance conditioning. Behav Neurosci,
ergic" nucleus basalis stimulation (Bao et al., 2001).
A second question that will need to be investigated
Bakin, J. S., & Weinberger, N. M. (1996). Induction of a physiologi-
in further detail is whether different forms of plastic-
cal memory in the cerebral cortex by stimulation of the nucleus
ity are amenable to the same neurochemical modula-
basalis. Proc Natl Acad Sci USA, 93, 11219-11224.
tion. If different forms of plasticity are not manifesta-
Bakin, J. S., & Weinberger, N. M. (1990). Classical conditioning
tions of the same cellular processes it will be unlikely
induces CS−specific receptive field plasticity in the auditory
that one neuromodulator equally influences all forms
cortex of the guinea pig. Brain Research, 536, 271-286.
of plasticity. Indeed for the cholinergic system it has
Bao, S., Chan, V. T., & Merzenich, M. M. (2001). Cortical re-
recently been shown that despite its role in learning-
modelling induced by activity of ventral tegmental dopamineneurons. Nature, 412, 79-83.
induced plasticity, cholinergic function is not necessaryfor lesion-induced plasticity (Kamke et al. 2005). Fur-
Boyeson, M. G., & Feeney, D. M. (1990). Intraventricular nore-
pinephrine facilitates motor recovery following sensorimotor
thermore it would be valuable to know, whether similar
cortex injury. Pharmacol Biochem Behav, 35, 497-501.
neurochemical mechanisms operate in different prima-
Breitenstein, C., Floel, A., Korsukewitz, C., Wailke, S., Bushuven, S.,
ry sensory and motor cortices. If this was the case,
& Knecht, S. (2006). A shift of paradigm: From noradrenergic
many of the findings on training-induced plasticity and
to dopaminergic modulation of learning? J Neurol Sci, 248,
recovery of function obtained for example in the motor
system might also be applied to the auditory system.
Breitenstein, C., Wailke, S., Bushuven, S., Kamping, S., Zwitserlood,
Finally, it will be necessary to show that the neuro-
P., Ringelstein, E. B., & Knecht, S. (2004). D-amphetamineboosts language learning independent of its cardiovascular and
chemical modulation of plasticity found in animals can
motor arousing effects. Neuropsychopharmacology, 29, 1704-
also be observed in the human brain and that results
obtained with rather simple auditory stimuli also apply
Brown, M., Irvine, D. R., & Park, V. N. (2004). Perceptual learning
to more complex stimuli. Recently the combination
on an auditory frequency discrimination task by cats: associa-
of psychopharmacology and functional neuroimaging
tion with changes in primary auditory cortex. Cerebral Cortex,
(also known as pharmacological MRI) has been used
14, 952-965.
increasingly to investigate the pharmacological modu-
Butefisch, C. M., Davis, B. C., Sawaki, L., Waldvogel, D., Classen,
lation of brain activity (Honey and Bullmore, 2004). A
J., Kopylev, L., & Cohen, L. G. (2002). Modulation of use-dependent plasticity by d-amphetamine. Ann Neurol, 51, 59-
similar approach using MEG will add valuable infor-
mation on drug action with high temporal resolution
Callan, D. E., Tajima, K., Callan, A. M., Kubo, R., Masaki, S.,
(Kahkonen, 2006). Such approaches in combination
& kahane-Yamada, R. (2003). Learning-induced neural plas-
with suitable paradigms for investigating auditory plas-
ticity associated with improved identification performance af-
ticity will enable researchers to study the neurochem-
ter training of a difficult second-language phonetic contrast.
Neuroimage, 19, 113-124.
ical modulation of neural activity in the human audi-tory cortex and provide an experimental approach that
Cansino, S., & Williamson, S. J. (1997). Neuromagnetic fields reveal
cortical plasticity when learning an auditory discrimination
has relevance to studying mechanisms of recovery and
task. Brain Research, 764, 53-66.
treatment effects in patients with injury.
Condon, C. D., & Weinberger, N. M. (1991). Habituation produces
Acknowledgements: The work on cholinergic mod-
frequency-specific plasticity of receptive fields in the auditory
ulation in the auditory cortex was funded by a research
cortex. Behavioral Neuroscience, 105, 416-430.
grant from the German Research Foundation (DFG TH
Conner, J. M., Chiba, A. A., & Tuszynski, M. H. (2005). The basal
forebrain cholinergic system is essential for cortical plasticityand functional recovery following brain injury. Neuron, 46,173-179.
Dietrich, V., Nieschalk, M., Stoll, W., Rajan, R., & Pantev, C.
(2001). Cortical reorganization in patients with high frequencycochlear hearing loss. Hear Res, 158, 95-101.
Acquas, E., Wilson, C., & Fibiger, H. C. (1996). Conditioned and
unconditioned stimuli increase frontal cortical and hippocam-
Drouin, C., & J.-P. Tassin, 2002, Norepinephrine, in VS Ramachan-
pal acetylcholine release: effects of novelty, habituation and
dran ed., Encyclopedia of the Human Brain: Elsevier, pp.
fear. The Journal of Neuroscience, 16, 3089-3096.
C.M. Thiel / Pharmacological modulation of learning-induced plasticity in human auditory cortex
Edeline, J. M. (1999). Learning-induced physiological plasticity
Kimura, F., Fukuda, M., & Tsumoto, T. (1999). Acetylcholine sup-
in the thalamo-cortical sensory systems: a critical evaluation
presses the spread of excitation in the visual cortex revealed
of receptive field plasticity, map changes and their potential
by optical recording: possible differential effect depending on
mechanisms. Prog Neurobiol, 57, 165-224.
the source of input [In Process Citation]. Eur J Neurosci, 11,3597-3609.
Edeline, J. M., Pham, P., & Weinberger, N. M. (1993). Rapid de-
velopment of learning-induced receptive field plasticity in the
Kisley, M. A., & Gerstein, G. L. (2001). Daily variation and appet-
auditory cortex. Behav Neurosci, 107, 539-551.
itive conditioning-induced plasticity of auditory cortex recep-tive fields. Eur J Neurosci, 13, 1993-2003.
Edeline, J. M., & Weinberger, N. M. (1993). Receptive field plasticity
in the auditory cortex during frequency discrimination training:
Krnjevic, K., & Phillis, J. W. (1963b).
Iontophoretic studies of
selective retuning independent of task difficulty. Behavioral
neurones in the mammalian cerebral cortex. J Physiol, 165,
Neuroscience, 107, 82-103.
Feeney, D. M., Gonzalez, A., & Law, W. A. (1982). Amphetamine,
Krnjevic, K., & Phillis, J. W. (1963a). Acetylcholine-sensitive cells
haloperidol, and experience interact to affect rate of recovery
in the cerebral cortex. J Physiol, 166, 296-327.
after motor cortex injury. Science, 217, 855-857.
Manunta, Y., & Edeline, J. M. (1998). Effects of noradrenaline on
rate-level function of auditory cortex neurons: is there a "gat-
Feeney, D. M., & Hovda, D. A. (1985). Reinstatement of binocular
ing" effect of noradrenaline? Experimental Brain Research,
depth perception by amphetamine and visual experience after
visual cortex ablation. Brain Research, 342, 352-356.
Manunta, Y., & Edeline, J. M. (1997).
Effects of noradrenaline
Gaab, N., Gaser, C., & Schlaug, G. (2006). Improvement-related
on frequency tuning of rat auditory cortex neurons. Eur J
functional plasticity following pitch memory training. Neu-
Neurosci, 9, 833-847.
roimage, 31, 255-263.
Manunta, Y., & Edeline, J. M. (2004). Noradrenergic induction of
Gonzalez-Lima, F., & Scheich, H. (1986). Neural substrates for tone-
selective plasticity in the frequency tuning of auditory cortex
conditioned bradycardia demonstrated with 2-deoxyglucose.
neurons. Journal of Neurophysiology, 92, 1445-1463.
II. Auditory cortex plasticity. Behav Brain Res, 20, 281-293.
Martinsson, L., & Eksborg, S. (2004). Drugs for stroke recovery:
Guenther, F. H., Nieto-Castanon, A., Ghosh, S. S., & Tourville, J. A.
the example of amphetamines. Drugs Aging, 21, 67-79.
(2004). Representation of sound categories in auditory cortical
McLin, D. E., III, Miasnikov, A. A., & Weinberger, N. M. (2002).
maps. J Speech Lang Hear Res, 47, 46-57.
Induction of behavioral associative memory by stimulation of
Hars, B., Maho, C., Edeline, J. M., & Hennevin, E. (1993). Basal
the nucleus basalis. Proc Natl Acad Sci USA, 99, 4002-4007.
forebrain stimulation facilitates tone-evoked responses in the
Menning, H., Roberts, L. E., & Pantev, C. (2000). Plastic changes
auditory cortex of awake rat. Neuroscience, 56, 61-74.
in the auditory cortex induced by intensive frequency discrim-
Honey, G., & Bullmore, E. (2004). Human pharmacological MRI.
ination training. Neuroreport, 11, 817-822.
Trends Pharmacol Sci, 25, 366-374.
Metherate, R., & Ashe, J. H. (1991). Basal forebrain stimulation
Irvine, D. R., Rajan, R., & Brown, M. (2001). Injury- and use-
modifies auditory cortex responsiveness by an action at mus-
related plasticity in adult auditory cortex. Audiol Neurootol,
carinic receptors. Brain Research, 559, 163-167.
6, 192-195.
Metherate, R., & Weinberger, N. M. (1989). Acetylcholine produces
Irvine, D. R., Rajan, R., & McDermott, H. J. (2000). Injury-induced
stimulus-specific receptive field alterations in cat auditory cor-
reorganization in adult auditory cortex and its perceptual con-
tex. Brain Research, 480, 372-377.
sequences. Hear Res, 147, 188-199.
Miasnikov, A. A., McLin, D., & Weinberger, N. M. (2001). Mus-
carinic dependence of nucleus basalis induced conditioned re-
Jancke, L., Gaab, N., Wustenberg, T., Scheich, H., & Heinze, H. J.
ceptive field plasticity. Neuroreport, 12, 1537-1542.
(2001). Short-term functional plasticity in the human auditorycortex: an fMRI study. Brain Res Cogn Brain Res, 12, 479-
Molchan, S. E., Sunderland, T., McIntosh, A. R., Herscovitch, P.,
& Schreurs, B. G. (1994). A functional anatomical study ofassociative learning in humans. Proc Natl Acad Sci USA, 91,
Juliano, S. L., Ma, W., & Eslin, D. (1991). Cholinergic depletion pre-
vents expansion of topographic maps in somatosensory cortex.
Proc Natl Acad Sci USA, 88, 780-784.
Moore, R. Y., & Bloom, F. E. (1978). Central catecholamine neuron
systems: anatomy and physiology of the dopamine systems.
Kahkonen, S. (2006).
Magnetoencephalography (MEG): a non-
Annu Rev Neurosci, 1, 129-169.
invasive tool for studying cortical effects in psychopharmacol-ogy. Int J Neuropsychopharmacol, 9, 367-372.
Morris, J. S., Friston, K. J., & Dolan, R. J. (1998). Experience-
dependent modulation of tonotopic neural responses in human
Kamke, M. R., Brown, M., & Irvine, D. R. (2005). Basal forebrain
auditory cortex. Proc R Soc Lond B Biol Sci, 265, 649-657.
cholinergic input is not essential for lesion-induced plasticityin mature auditory cortex. Neuron, 48, 675-686.
Nudo, R. J., Milliken, G. W., Jenkins, W. M., & Merzenich, M. M.
(1996). Use-dependent alterations of movement representa-
Kilgard, M. P. (2005). Cortical map reorganization without cholin-
tions in primary motor cortex of adult squirrel monkeys. J
ergic modulation. Neuron, 48, 529-530.
Neurosci, 16, 785-807.
Kilgard, M. P., & Merzenich, M. M. (1998). Cortical map reorga-
Ohl, F. W., & Scheich, H. (2005). Learning-induced plasticity in
nization enabled by nucleus basalis activity [see comments].
animal and human auditory cortex. Curr Opin Neurobiol, 15,
Science, 279, 1714-1718.
C.M. Thiel / Pharmacological modulation of learning-induced plasticity in human auditory cortex
Pantev, C., Ross, B., Fujioka, T., Trainor, L. J., Schulte, M., & Schulz,
in the rat. J Neurotrauma, 14, 459-468.
M. (2003). Music and learning-induced cortical plasticity. Ann
Schreurs, B. G., McIntosh, A. R., Bahro, M., Herscovitch, P., Sun-
N Y Acad Sci, 999, 438-450.
derland, T., & Molchan, S. E. (1997). Lateralization and be-
Pariente, J., Loubinoux, I., Carel, C., Albucher, J. F., Leger, A.,
havioral correlation of changes in regional cerebral blood flow
Manelfe, C., Rascol, O., & Chollet, F. (2001). Fluoxetine mod-
with classical conditioning of the human eyeblink response. J
ulates motor performance and cerebral activation of patients
Neurophysiol, 77, 2153-2163.
recovering from stroke. Ann Neurol, 50, 718-729.
Stark, H., & Scheich, H. (1997). Dopaminergic and serotonergic neu-
Parton, A., Coulthard, E., & Husain, M. (2005). Neuropharmacolog-
rotransmission systems are differentially involved in auditory
ical modulation of cognitive deficits after brain damage. Curr
cortex learning: a long-term microdialysis study of metabo-
Opin Neurol, 18, 675-680.
lites. J Neurochem, 68, 691-697.
Pleger, B., Schwenkreis, P., Grunberg, C., Malin, J. P., & Tegenthoff,
Tegenthoff, M., Cornelius, B., Pleger, B., Malin, J. P., &
M. (2004). Fluoxetine facilitates use-dependent excitability of
Schwenkreis, P. (2004).
Amphetamine enhances training-
human primary motor cortex. Clin Neurophysiol, 115, 2157-
induced motor cortex plasticity. Acta Neurol Scand, 109, 330-
Plewnia, C., Hoppe, J., Cohen, L. G., & Gerloff, C. (2004). Improved
Thiel, C. M., Bentley, P., & Dolan, R. J. (2002a). Effects of choliner-
motor skill acquisition after selective stimulation of central
gic enhancement on conditioning-related responses in human
norepinephrine. Neurology, 62, 2124-2126.
auditory cortex. Eur J Neurosci, 16, 2199-2206.
Poremba, A., Jones, D., & Gonzalez-Lima, F. (1998). Classical con-
Thiel, C. M., Friston, K. J., & Dolan, R. J. (2002b). Cholinergic mod-
ditioning modifies cytochrome oxidase activity in the auditory
ulation of experience-dependent plasticity in human auditory
system. Eur J Neurosci, 10, 3035-3043.
cortex. Neuron, 35, 567-574.
Recanzone, G. H., Schreiner, C. E., & Merzenich, M. M. (1993).
Thiel, C. M., Muller, C. P., Huston, J. P., & Schwarting, R. K.
Plasticity in the frequency representation of primary auditory
(2000). Auditory noise can prevent increased extracellular
cortex following discrimination training in adult owl monkeys.
acetylcholine levels in the hippocampus in response to aversive
J Neurosci, 13, 87-103.
stimulation. Brain Res, 882, 112-119.
Rutkowski, R. G., & Weinberger, N. M. (2005). Encoding of learned
Tobey, E. A., Devous, M. D., Sr., Buckley, K., Overson, G., Harris,
importance of sound by magnitude of representational area
T., Ringe, W., & Martinez-Verhoff, J. (2005). Pharmacological
in primary auditory cortex. Proc Natl Acad Sci USA, 102,
enhancement of aural habilitation in adult cochlear implant
users. Ear Hear, 26, 45S-56S.
Sato, H., Hata, Y., Masui, H., & Tsumoto, T. (1987). A functional
Tremblay, N., Warren, R. A., & Dykes, R. W. (1990). Electrophysi-
role of cholinergic innervation to neurons in the cat visual
ological studies of acetylcholine and the role of the basal fore-
cortex. Journal of Neurophysiology, 58, 765-780.
brain in the somatosensory cortex of the cat. II. Cortical neu-rons excited by somatic stimuli. Journal of Neurophysiology,
Sawaki, L., Boroojerdi, B., Kaelin-Lang, A., Burstein, A. H., Bute-
fisch, C. M., Kopylev, L., Davis, B., & Cohen, L. G. (2002).
Cholinergic influences on use-dependent plasticity. J Neuro-
Weinberger, N. M. (2004). Specific long-term memory traces in
physiol, 87, 166-171.
primary auditory cortex. Nat Rev Neurosci, 5, 279-290.
Scheidtmann, K., Fries, W., Muller, F., & Koenig, E. (2001). Ef-
Weinberger, N. M., Javid, R., & Lepan, B. (1993). Long-term reten-
fect of levodopa in combination with physiotherapy on func-
tion of learning-induced receptive-field plasticity in the audi-
tional motor recovery after stroke: a prospective, randomised,
tory cortex. Proc Natl Acad Sci USA, 90, 2394-2398.
double-blind study. Lancet, 358, 787-790.
Xiang, Z., Huguenard, J. R., & Prince, D. A. (1998). Cholinergic
Schmanke, T., & Barth, T. M. (1997).
Amphetamine and task-
switching within neocortical inhibitory networks.
specific practice augment recovery of vibrissae-evoked fore-
limb placing after unilateral sensorimotor cortical injury
Source: https://www.unifr.ch/inph/vclab/assets/files/PDFs/thiel.ach.auditory.review.2007.pdf
RMM NÚMERO 7 - PÁG. 15 (VOL 1 - PÁG. 207) NOVIEMBRE 2013 puede ser muy útil a la hora de planificar los recursos humanos Y volviendo al tema por el que comenzamos, esto tendrá sanitarios: según refiere el documento citado, experiencias en también repercusiones en la política de jubilación. La presión varios países muestran que la prolongación de la vida activa de
Lara et al, Cancerología 1 (2006): 283-295 Manejo del Paciente Terminal Argelia Lara Solares, Antonio C. Tamayo Valenzuela, Sandra P. Gaspar Carrillo Unidad de Medicina del Dolor y Paliativa. Instituto Nacional de Ciencias Médicas y Nutrición "Salvador Zubirán" A Terminal disease is by definition, an advanced progressive and not curable il ness that does not respond to a specific