Microsoft word - v-fuel company and technology sheet 2008.doc
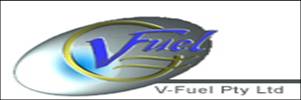
COMPANY AND TECHNOLOGY INFORMATION SHEET
V-Fuel Pty Ltd
Energy storage has become the critical issue for the world's future energy supply and redox flow cells offer the best combination of energy efficiency, capital cost and life cycle costs compared with other technologies. The UNSW Vanadium Redox Flow Battery has been internationally acclaimed as the most promising technology currently available for new energy storage applications in the range from several kilowatts to several megawatts range where storage times up to several hours are required. With V-Fuel's recent advances in the development of a low cost stack technology for the VRB, the financial viability of the system can now be achieved so that energy storage products can be produced for a broad market. This emerging business opportunity has the potential to generate millions of dollars in export earnings, while also helping to alleviate serious global energy supply and environmental problems. V-Fuel Pty Ltd was established in January 2005 with the support of the Victorian Government funded Centre for Energy and Greenhouse Technologies Pty Ltd to commercialise the Vanadium Redox Flow Battery developed at the University of New South Wales in Sydney. Its principal shareholder, Magnam Technologies Pty Ltd is the initial Start-up company that was formed in 2003 by two of the original inventors of the Vanadium Redox Battery Technology (VRB), Professor Maria Skyllas-Kazacos of the School of Chemical Engineering and Industrial Chemistry, UNSW and Mr Michael Kazacos. V-Fuel has developed new proprietary technology for the proven G1 VRB and has successfully secured an exclusive world-wide license for the new Generation 2 Vanadium Bromide Redox Battery (G2 V/Br) from the University of NSW commercial arm, NS Innovation Pty Ltd. With almost twice the energy density compared with the original VRB (G1), the G2 V/Br battery will allow V-Fuel to enter markets and applications where the battery weight or footprint area restrictions would preclude use of the G1 VRB. Having the same electrolyte in both half-cells, the V/Br system has no problems of cross-contamination and combines the best features of the G1 VRB system with a high energy density and wider temperature capability. A recent market analysis conducted from survey responses from a range of companies that have contacted V-Fuel to purchase redox batteries, has identified 2 primary stack products that will be developed and manufactured by V-Fuel. These are a 5 kW stack for use in small-scale systems with up to 50 kW power requirements, and a 50 kW stack for use in large-scale applications of 50 kW to several MW in size. V-Fuel is now ready to begin manufacture of 1-5 kW stacks for application in small to medium size energy storage systems and with its new low cost, high performance proprietary membrane, expects to be able to supply products at close to half the price as its current competitors.
V-Fuel Company and Technology Information Package 2008
1 INTRODUCTION
The world demand for efficient energy storage systems has been growing steadily in the
last 20 years as concerns over Global Warming and urban pollution have led to pressure
from environmental groups around the world for increased use of renewable energy
technologies. In combination with renewable resources, energy storage can increase the
value of photovoltaic (PV) and wind-generated electricity, making supply coincident with
periods of peak consumer demand. Energy storage systems can also be used to follow
load, stabilize voltage & frequency, manage peak loads, improve power quality, defer
upgrade investments and provide uninterruptible power for many industrial and
commercial applications. Batteries are ideal for storing electrical energy since they allow
high energy conversion efficiencies to be achieved (over 80%) and long storage times.
The world market for batteries is estimated at about US $15 billion each year. The
Energy Storage Association, USA (ESA), estimates that industrial batteries, as might be
used in uninterruptible power supplies, power quality applications, standby and reserve
batteries amount to US $5 billion each year. The Energy Storage Association also has
compared the performance parameters of different types of energy storage systems
ranging from flywheels, pumped hydro, capacitors and different battery technologies, and
redox flow batteries are shown to have many technical benefits over other energy storage
systems as well as an excellent combination of energy efficiency, capital cost and life
cycle costs compared with other technologies (Ref:
http://www.electricitystorage.org/tech/technologies_comparisons.htm).
Figure 1 shows a cost comparison of the redox flow battery against other battery
technologies and a clear advantage is seen for the redox systems.
Figure 1. Cost per cycle comparisons of energy storage technologies (Ref:
http://www.electricitystorage.org/tech/technologies_comparisons_percyclecost.htm)
V-Fuel Company and Technology Information Package 2008
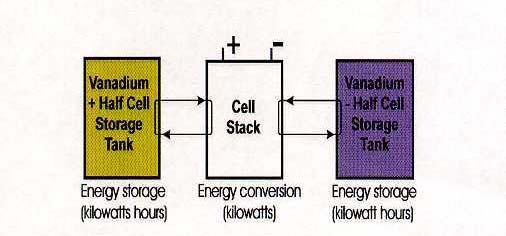
The Redox Flow Cell is an electrochemical system which allows energy to be stored in two solutions containing different redox couples with electrochemical potentials sufficiently separated from each other to provide an electromotive force to drive the oxidation-reduction reactions needed to charge and discharge the cell. Unlike conventional batteries, the redox flow cell stores energy in the solutions, so that the capacity of the system is determined by the size of the electrolyte tanks, while the system power is determined by the size of the cell stacks. The redox flow cell is therefore more like a rechargeable fuel cell than a battery (see Figure 2).
Figure 2: Redox flow cell concept with separate energy conversion and energy
storage components
While the Redox Flow Cell concept has been around for close to 30 years with several
systems evaluated by various groups around the world, only the Vanadium Redox battery
pioneered at UNSW (1 - 42) has reached commercial fruition.
2. THE UNSW GENERATION 1 VANADIUM REDOX BATTERY (VRB).
Of the redox flow cells developed to date, the vanadium redox flow battery, or G1 VRB
system, pioneered at the University of New South Wales, Australia, has shown the
greatest potential with high energy efficiencies of over 80% in large installations and
long cycle life. As illustrated in Figure 2 above, the Vanadium Redox Flow Battery
employs the V(III)/V(II) and V(V)/V(IV) redox couples in sulphuric acid as the negative
and positive half-cell electrolytes respectively. The charge-discharge reaction occurring
in the vanadium redox cell are:
At the negative electrode:
V3+ + e- Í=Î V2+
At the positive electrode:
2 + 2H+ + e Í===Î VO2+ + H2O
V-Fuel Company and Technology Information Package 2008
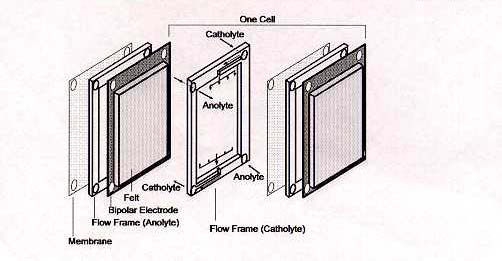
The standard cell potential E° (cell) is 1.26 Volts at concentrations of 1 mole per litre and at 25°C. Under actual cell conditions, however, the open-circuit cell voltage is 1.4 Volts at 50% state-of-charge and 1.6 Volts at 100% SOC. The electrolyte for the vanadium battery is typically 2 M vanadium sulphate in 2.5 M H2SO4, the vanadium sulphate (initially 1 M V (III) + 1 M V (IV)) being prepared by chemical reduction or electrolytic dissolution of V2O5 powder. The basic components of the redox cell are illustrated in Figure 3.
Figure 3: Basic Components of VRB cell stack
2.1 Features and Advantages of the VRB:
Most of the advantages of the vanadium battery are thus due to the use of the same
element in both half-cells which avoids problems of cross-contamination of the two half-
cell electrolytes during long-term use. This means that the electrolytes have an indefinite
life so that waste disposal issues are minimised.
Other advantages of the VRB include:
• Low cost for large storage capacities. Cost per kWh decreases as energy
storage capacity increases, typical projected battery costs for 8 or more hours of storage being as low as US$150 per kWh.
• Existing systems can be readily upgraded and additional storage capacity can
be easily installed by changing the tanks and volumes of electrolyte.
• High energy efficiencies between 80 and 90% in large installations.
• Capacity and state-of-charge of the system can be easily monitored by
employing an open-circuit cell.
• Negligible hydrogen evolution during charging
• Can be fully discharged without harm to the battery
V-Fuel Company and Technology Information Package 2008
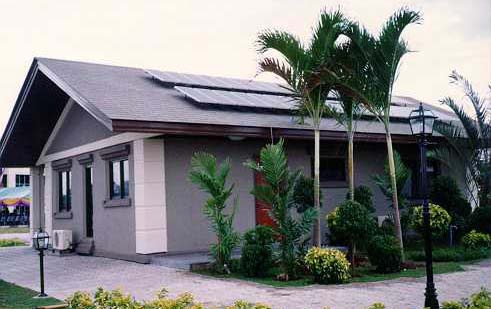
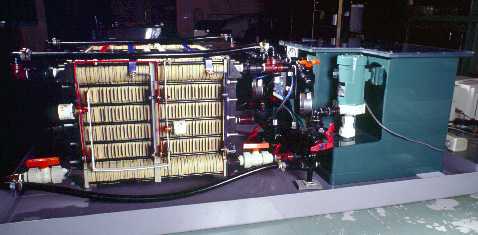
• All cells fed with same solutions and therefore are at the same state-of-charge
• No problems of cross-contamination therefore solutions have indefinite life. • Long cycle life
• Easy maintenance.
• Can be both electrically recharged and mechanically refueled
2.2 UNSW Vanadium Battery Research, Development and Demonstration Projects
The Vanadium Redox Battery (VRB) was taken from the initial concept stage by Skyllas-
Kazacos and co-workers at UNSW in 1984 through the development and demonstration
of several 1-4 kW prototypes in stationary and electric vehicle applications over a 15 year
period at UNSW. UNSW also led the world in the construction and demonstration of
VRB systems in field trials, including design and installation of the first ever VRB used
in a demonstration Solar House in Thailand (Figure 4 and ref 40), an emergency back-up
system for submarines (Figure 5) and the world's first vanadium battery powered electric
golf cart (Figure 6 and ref 41).
Figure 4: Vanadium Battery powered Solar Demonstration House in Thailand
Figure 5: Emergency Back-up Battery for Submarines (sponsored by Australian
Dept of Defence)
V-Fuel Company and Technology Information Package 2008
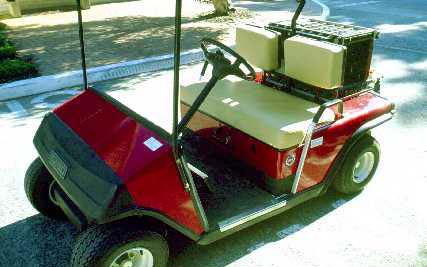
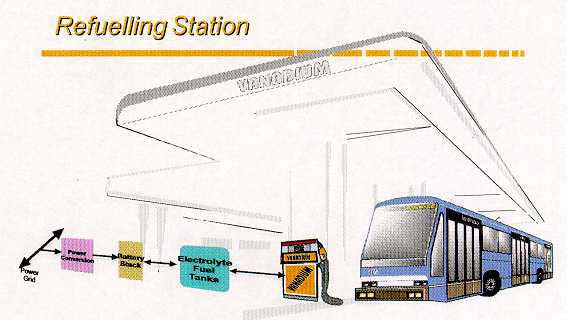
Figure 6: UNSW Vanadium Fuel Cell Powered Electric Golf Cart
Thus, a unique feature of the VRB is its ability to be recharged both conventionally or
with mechanical refueling by exchanging spent solutions at suitable refueling stations as
illustrated in Figure 7. This concept has attracted enormous interest and enthusiasm from
the community and from government and industry groups over the years and a world-first
vanadium battery powered electric golf cart, sponsored by Pacific Power was
commissioned and field tested by UNSW in 1995/96.
Figure 7: VRB refuelling station
V-Fuel Company and Technology Information Package 2008
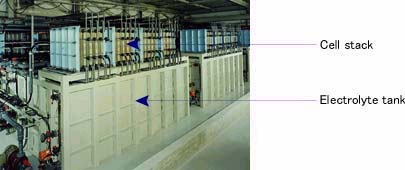
2.3 Commercialisation of the VRB
The UNSW Vanadium Redox Battery technology is currently being successfully
commercialised in a range of stationary applications such as wind, solar and load-
levelling installations around the world and the demand for these systems is growing
rapidly in North America, Japan, Europe and Australia.
In 1993 licences for the VRB were issued by UNSW to Thai Gypsum Products in
Thailand for Solar House applications, as well as to Mitsubishi Chemicals and Kashima-
Kita Electric Power Corporation in Japan where a 200kW/800kWh load-levelling
demonstration system was installed in 1997 (44). In 1998, the UNSW VRB technology
was sold to the Australia listed company Pinnacle VRB. In 1999, Pinnacle VRB entered
into a new licence agreement with Sumitomo Electric Industries (SEI) who built a 450
kW/ 1 MWh vanadium redox battery load levelling demonstration system at the Kansai
Electric Power Plant in Japan (Figure 8), as well as several other VRB systems for wind
energy storage and other stationary applications (45, 46).
Figure 8: SEI Substation Demonstration System Load leveling DC 450kW x 2h
(Ref: http://www.sei.co.jp/sn/0105/p1.html)
More recently, a 4 MW/6 MWh VRB was installed by Sumitomo on the Japanese Island
of Hokkaido to store wind power and stabilize the output shift of wind generation.
The advantage of the VRB for wind power output stabilisation is the very high cycle life
of the VRB compared with other battery systems. In fact, SEI have reported more than
12,000 charge-discharge cycles with a 25 kW VRB stack, or up to 8 years projected life.
After 8 years, it is possible to replace the membrane and extend the life of the system
even further. This means that the replacement costs of the VRB would be significantly
lower than other types of batteries.
In 2004, a 250 kW/1 MWh installation was constructed and commissioned by Pinnacle
VRB on King Island in Tasmania to provide storage energy for a wind generator for diesel
fuel displacement. This project was funded by the Australian Greenhouse Office and the
customer was Tasmania Hydro. The stacks for this system were supplied by SEI, Japan,
V-Fuel Company and Technology Information Package 2008
electrolyte was supplied by Highveld, South Africa, with other components being sourced from elsewhere. With more than 20 installations around the world totalling over 20 MWh installed capacity, the G1 VRB is now regarded as a proven technology. Achieving the necessary cost structure for each of the identified markets, however, will determine its widespread consumer take-up in the years ahead. In April, 2006, the basic G1 VRB patent expired, thereby allowing V-Fuel to commercialise G1 VRB systems around the world using its proprietary new low cost stack technology. Figure 9 that show V-Fuel's new 1-2 kW/6 kWh G1 VRB prototype that is now ready for production in stack sizes up to 5-10 kW.
Figure 9: 1-2 kW/6 kWh VRB incorporating V-Fuel's low cost stack design.
A 25-50 kW module is soon to be developed by V-Fuel for application in large MW sized
installations for load-leveling, power arbitrage and large-scale renewable energy storage.
V-Fuel Company and Technology Information Package 2008
3. SPECIFIC BENEFITS OF THE VANADIUM BATTERY IN DIFFERENT
STATIONARY APPLICATIONS.
A. Remote area power supply system (RAPPS) applications
Australia's large land mass and great distances between major urban centres, makes it particularly dependent on stand-alone power systems for remote communities, mining towns and isolated homesteads and tourist locations. Its heavy dependence on diesel fuel for diesel grids and smaller stand-alone systems is a great cost to the community, both financially and environmentally. Most RAPSS systems employ a diesel generator with or without solar panels or wind generator and a lead-acid battery for storage. Due to the poor performance and short cycle life of the lead-acid battery under deep discharge cycling, there is a heavy dependence on the diesel generator for power. The cost of diesel-fired power generation is comparatively high, typically in the range of AUD 0.14 – 0.60 per kWh. For small remote diesel generating sets in the range 1 – 15 kWe, the cost can be as high as AUD 2.50 per kWh With a low cost, efficient battery, however, considerable reduction or complete replacement of the diesel generator could be possible. The important features of the vanadium battery for this application are: - low capital and generation cost per kWh for large storage capacities - easy maintenance
B. Large-scale photovoltaic applications
VRB Advantages :
• Capacity easily upgraded by changing tanks and volumes of electrolyte. • Battery voltage chosen by tapped cells - allows change of operating condition
according to variations in solar insulation & load to achieve greater efficiency.
• Appropriate voltage chosen to control solar array performance at optimum
conditions - provides low cost, high efficiency maximum power point tracker, allows battery to operate as DC transformer.
• Capacity & SOC monitored, by open-circuit cell. • Target cost/kWh decreases as capacity increases (as low as US$150 / kWh.)
C. Large-scale wind energy storage.
Wind Energy is now emerging as the most promising renewable energy source for short
term commercialization. However, wind generators suffer from output power instability
and as with all renewable energy technologies, the energy production cannot match the
demand. A VRB wind installation by SEI in Japan has demonstrated output power
stabilization that is only feasible due to large cycle life of the VRB. More than 12,000
V-Fuel Company and Technology Information Package 2008
cycles have been demonstrated with 20 kW stacks and with suitable materials selection,
achieving more than 1.5million cycles is expected for the VRB. The life of the VRB is
measured in years rather than cycles, so theoretically an infinite number of cycles is
possible over the life-time of the battery stacks. The life-time can further be extended by
periodically replacing the membrane, so that replacement costs are also much lower than
other battery technologies while waste materials are also minimized by the re-use of the
electrolytes.
D. Load leveling and distributed power systems.
The use of energy storage allows for:
• more efficient use of energy
• deferral of capital investment in new power stations • Battery energy storage systems built in 1-2 years vs 10 years for power station • No emissions or noise so can be installed in urban areas to meet peak demands • Can defer investment in new transmission lines by installing battery plants at load
• Battery modules can be added as demand increases
In addition to the above, the most important benefit of the VRB in such applications is the
high energy efficiency (over 80% overall energy efficiency has been demonstrated in large
demonstration scale VRB systems), long cycle life and low cost per kWh.
E. Emergency back-up power and uninterruptible power systems (UPS)
Many UPS systems employ lead-acid batteries with or without a diesel generator. Due to
the poor performance and short cycle life of the lead-acid battery under deep discharge
cycling, there is a heavy dependence on the diesel generator for longer term power
generation in the event of power failure. With a low cost, efficient VRB, however,
considerable reduction or complete replacement of the diesel generator would be possible.
While both the VRB and Generation 2 V/Br system share many features in common, it is
expected that the potentially higher vanadium bromide solubility over a wider range of
temperatures will make the V/Br a superior choice in these applications that require a
small footprint area and higher energy density.
Uninterruptible power for telecommunications is an enormous potential application for the G2 V/Br, and following the power failures in the United States during 2003, USA telcom providers are moving towards fully stand-alone back-up power systems that will secure their systems against loss of communication during power failures. A VRB or V/Br system can inject large quantities of power and energy into the grid within milliseconds, aiding in the recovery from blackouts, or stabilizing the grid before failures cascade through the system. The VRB and V/Br can either be deployed at large scale (tens of megawatt hours from each unit) or through a distributed network of smaller scale facilities.
V-Fuel Company and Technology Information Package 2008
5. G1 VRB COST PROJECTIONS AS A FUNCTION OF STORAGE TIME.
5.1 Capital costs as a function of storage time
In the case of redox flow cells, the independent sizing of the system power and energy,
means that the cost per kWh of the entire battery is a function of the energy storage
capacity. The total system cost was calculated on the basis of a projected stack cost of
approximately $ 420 per kW and an electrolyte system cost of $230 per kWh (including
electrolyte, tanks, pumps, controller etc) and the data is presented in Figure 10.
G1 VRB Capital costs per kWh versus
storage time
$/kWh 300
Figure 10: Total battery costs per kWh versus storage time for electrolyte system
cost of $230 per kWh and assumed stack cost of $420/kW
This figure shows that for storage times of 2 hours, a total battery cost of just over $400
per kWh can be achieved, this dropping to less than $300 per kWh for storage times
above 4 hours.
5.2 Cost of generated power as a function of storage time
Another way of evaluating the cost competitiveness of the V-Fuel battery is to calculate
the cost per kWh of generated energy over the life of the battery installation. This
involves input data for:
1. cost per kW of the stack
2. cost per kWh of electrolyte
3. life of membrane – with periodic replacement of membrane over an assumed total
system life of 20 years
4. cost of replacement membrane
V-Fuel Company and Technology Information Package 2008
5. annual maintenance cost per kWh installed capacity.
Cost comparisons were also made between the VRB and the Lead-acid battery. The Sungel range, 2SG4500 battery was selected as base battery for building the battery bank with 5 kW nominal power output for different storage times and the annualised costs assuming a 9.7 year lifetime and a discount rate of 8% p.a. are presented below.
Figure 11: Cost of generated power per kWh as a function of storage time for VRB
and Lead-acid battery.
The important feature of this graph is that the cost of each kWh generated by the VRB
over its life, is considerably lower than that provided by lead-acid batteries for storage
times in excess of 1.5 hours. This confirms the significant cost benefit of the VRB in
applications that require long storage times, for example, renewable energy storage and
remote area power systems for residential, industrial and sustainable tourism applications
CONTACTS.
For further information, please visit the V-Fuel site at: www.vfuel.com.au
Contact details: Email: [email protected]
Phone: 61-2-9540-1939
V-Fuel Company and Technology Information Package 2008
6. REFERENCES
1. "A study of the V(II)/V(III) redox couple for redox flow cell applications", E. Sum and M. Skyllas-
Kazacos, J. Power Sources, 15, 179-190 (1985).
2. "Investigation of V(V)/V(IV) system for use in positive half-cell of a redox battery", E. Sum,
Rychcik and M. Skyllas-Kazacos, J. Power Sources, 16, 85-95 (1985).
3. "New all-vanadium redox cell", M. Skyllas-Kazacos, M. Rychcik, R.G. Robins, A.G. Fane and M.
Green, J. Electrochem. Society, 133, 1057-1058 (1986).
4. "Evaluation of electrode materials for all-vanadium redox flow cell", M. Skyllas-Kazaocs and
Rychcik, J. Power Sources, 19, 45-54 (1987).
5. "Efficient Vanadium Redox Flow Cell", M. Skyllas-Kazacos and F. Grossmith, J. Electrochem. Soc., 134,
2950-2953 (1987).
6. "Characteristics of new all-vanadium redox flow battery", M. Rychcik and M. Skyllas- Kazacos, J. Power
Sources, 22, 59-67 (1988).
7. "All-vanadium redox battery", M. Skyllas-Kazacos and R.G. Robins, US Pat. No. 4,786,567, 1986. 8. "Characteristics and Performance of 1 kW Vanadium Redox Battery", M. Skyllas-Kazacos, D.
Kasherman, R. Hong and M. Kazacos, J. Power Sources, 35, 399-404 (1991).
9. "Electrochemical Behaviour of V(V)/V(IV) Redox Couple at Graphite Electrodes", S. Zhong and M.
Skyllas-Kazacos, J. Power Sources, 39, 1-9 (1992).
10. "Electrolyte Optimization of Vanadium Redox Cell" by M. Kazacos, M. Cheng and M. Skyllas-Kazacos,
J. Appl. Electrochem., 20, 463-467 (1990).
11. "Thermal Stability of Concentrated V(V) Electrolytes in the Vanadium Redox Cell", M.Skyllas-Kazacos,
C. Menictas and M. Kazacos, J. Electrochem. Soc., 143, L86-L88, April, 1996.
12. "Vanadium Compound Dissolution", M. Skyllas-Kazacos, M. Kazacos and R. McDermott, Sth. African
Patent No. 88/9244.
13. "Chemical Modification and Electrochemical Behaviour of Graphite Fibre in Acidic Vanadium Solutions",
B.T. Sun and M. Skyllas-Kazacos, Electrochem. Acta., 36, 513-517 (1991).
14. "Modification of Graphite Electrode Materials for Vanadium Redox Flow Cell Applications - Thermal
Treatment", B.T. Sun and M. Skyllas-Kazacos, Electrochimica Acta, 37, 1253-1269 (1992).
15. "Chemical Modification of Graphite Electrode Materials for Vanadium Redox Flow Battery Application -
Part II: Acid Treatments", B.T. Sun and M. Skyllas-Kazacos, Electrochimica Acta, 37, 2459-65 (1992).
16. "Preparation and Evaluation of Composite Membrane for Vanadium Redox Battery Applications", S.C.
Chieng, M. Kazacos and M. Skyllas-Kazacos, J. Power Sources, 39, 11-19
17. "Modification of Daramic Microporous Separator for Redox Flow Battery Applications", by S.C. Chieng
and M. Skyllas-Kazacos, J. Membrane Science, 75, 81-91 (1992).
18. "Permeation Selective Separators and Processes for making such Separators", J. Chieng, M. Kazacos and
M. Skyllas-Kazacos, PCT Patent Appl., Sept. 1992, AU9200491.
19. "Characterization of Novel Composite Membrane for Redox Flow Battery Applications", T. Mohammadi
and M. Skyllas-Kazacos, J.Memb.Sci. 98, 77-87 (1995).
20. "Preparation of Sulphonated Composite Membrane for Vanadium Redox Flow Battery Applications:, T.
Mohammadi and M. Skyllas-Kazacos, J. Membrane Sci, 107, 35-45 (1995).
21. "Use of Polyelectrolyte for Incorporation of ion-exchange groups in composite membranes for vanadium
redox flow battery applications", T. Mohammadi and M.Skyllas-Kazacos, J. Power Sources, 56, 91-96 (1995).
22. "Water Transport Study Across Commercial Ion Exchange Membranes in theVanadium Redox Cell", T.
Mohammadi and M. Skyllas-Kazacos, J. Power Sources, 133, 151-159 (1997).
23. "Evaluation of Chemical Stability of Some Membranes in Vanadium Solution", T. Mohammadi & M.
Skyllas-Kazacos, J. Applied Electrochemistry, 27, 153-160, (1996).
24. "Modification of Anion-exchange Membranes for Vanadium Redox Battery Applications", T.
Mohammadi and M. Skyllas-Kazacos, J. Power Sources, 63, 179-186, 1996.
25. "Design and Development of Vanadium Redox Battery Prototype", M. Skyllas-Kazacos, M. Kazacos, P.
Wegner and R. Burford, SERDF Project, Final Report, NSW, Office of Minerals and Energy, January, 1991.
26. "Design of Vanadium Battery for Remote Area Solar Energy Storage" Simon Ting, Honours Project
Thesis, UNSW, 1988 (supervised by M.Skyllas-Kazacos)
V-Fuel Company and Technology Information Package 2008
27. "High Energy Density Electrolyte for Vanadium Redox Battery", M. Kazacos and M. Skyllas-Kazacos,
South , African Patent, 94/9140, September, 1995. International Patent Appl. No PCT/AU94/00711, November, 1994.
28. "All-vanadium redox battery with additives", M. Skyllas-Kazacos, Patent Appl. No. PCT/AU88/00472,
29. "Evaluation of NH4VO3 Derived Electrolyte for Vanadium Redox Flow Battery", C. Menictas, M. Chen
and M. Skyllas-Kazacos, J. Power Sources, 45, 43-54 (1993).
30. "State-of-Charge of Redox Cell", by M. Skyllas-Kazacos, M. Kazacos, J. Joy and B.G. Madden,
Appl. No. PCT/AU89/00252, June 1989.
31. "Vanadium charging cell and vanadium dual battery system", M. Skyllas-Kazacos, M. Kazacos and R.
McDermott, Patent Appl. No. PCT/AU88/00473, Dec. 1988.
32. "Performance of carbon plastic electrodes in vanadium redox cell", by M. Kazacos and M. Skyllas-
Kazacos, J. Electrochem. Soc., 136, 2759-2760 (1989).
33. "Fabrication and Activation Studies of Conducting Plastic Electrodes in Acidic Vanadium Solutions" by S.
Zhong, M. Kazacos, R.P. Burford and M. Skyllas-Kazacos, J. Power Sources, 36, 29-43 (1991).
34. "Conductive Carbon-Polypropylene Composite Electrodes for Vanadium Redox Flow Battery",
Haddadi-Asl, M. Kazacos and M. Skyllas-Kazacos, J. Appl. Electrochem. 25, 29-33 (1995).
35. Carbon-Polymer Composite Electrodes for Redox Cells", V. Haddadi-Asl, M. Kazacos and M. Skyllas-
Kazacos, J. Applied Polymer Science, 57, 1455-1463 (1995).
36. "Flexible Conducting Plastic Electrode and Process for its Preparation, V. Haddadi-Asl, M. Kazacos and
M. Skyllas-Kazacos, US Patent No. 5,665,212, Sept, 1997.
37. "Studies of Chemical Regeneration of Vanadium (V) for Vanadium Redox Battery", M. Abdus Samad and
M. Skyllas-Kazacos, in Proceedings 9th Australasian Electrochemical Conference, Wollongong, February 1994, pp 65-1 to 65-4.
38. "Stabilised Electrolyte Solutions, Methods of Preparation Thereof and Redox Cells and Batteries
Containing Stabilised Electrolyte Solutions" M.Skyllas-Kazacos, Aust Patent No 696452, Dec 1998, US Patent No. 6,143,443, 7 Nov, 2000.
39. "Evaluation of Precipitation Inhibitors for Supersaturated Vanadyl Electrolytes for the Vanadium Redox
Battery", M. Skyllas-Kazacos, C. Peng and M. Cheng, Electrochemical and Solid State Letters, 2, 121, 1999.
40. "Improved PV System Performance using Vanadium Batteries", R. Largent, M. Skyllas- Kazacos and J.
Chieng, Proceedings IEEE, 23rd Photovoltaic Specialists Conference, Louisville, Kentucky, May 1993.
41. "Status of the Vanadium Battery Development Programme", C. Menictas, D.R. Hong, Z.H. Yan, J.
Wilson, M. Kazacos and M. Skyllas-Kazacos, Proceedings, Electrical Engineering Congress, Sydney, November 1994.
42. "Gelled Electrolyte Vanadium Battery" M. Skyllas-Kazacos, Provisional Patent Application, PCT
/AU01/00923, 27 July, 2001.
43. "Electrochemical Apparatus for Energy Storage and/or Power Delivery Comprising Multi-Compartment
Cells" R. Zitto, PCT/GB93/02110, October, 1993.
44. "Vanadium Redox Flow Battery with Resource Saving Recycle Ability. I Production of Electrolytic
Solution for Vanadium Batteries from Boiler Soot", M. Nakajima, M. Sawahata, S. Yoshida, K, Sato, H. Kaneko, A. Negishi and K. Nozaki, Denki Kagaku, 66, 600, 1998.
45. "Development of a Redox Flow Battery System", N. Tokuda, T. Kumamoto, T. Shigematsu, H. Deguchi,
T. Ito, N. Yoshikawa and T. Hara, SEI Technical Review, No 45, Jan. 1998
46. "Feature Article: Redox Flow Batteries" SEI News, http://www.sei.co.jp/sn/0105/p1.html 47. Sir John Samuels, EFuel Technologies, Private Communication, October, 2001 48. "Small-scale Laboratory Testing and Golf-Cart Trials of 3 Molar Vanadium Electrolyte", C. Menictas
and M. Skyllas-Kazacos, Report to Pinnacle Mining NL, Feb 1998.
49. Market and Applications for Advanced Batteries . Strategic Industry Research Foundation and
Redding Energy Management, April, 1995
50. L. Peron, in "Vanadium – Geology, Processing and Applications, Proceedings COM2002, Montreal,
2002. Editors, M. Taner et al.
V-Fuel Company and Technology Information Package 2008
Source: http://www.vfuel.com.au/infosheet.pdf
Citation: Transl Psychiatry (2015) 5, e640; doi:10.1038/tp.2015.143 ORIGINAL ARTICLEPredictors of placebo response in pharmacological and dietarysupplement treatment trials in pediatric autism spectrumdisorder: a meta-analysis A Masi1, A Lampit2, N Glozier1, IB Hickie1 and AJ Guastella1 Large placebo responses in many clinical trials limit our capacity to identify effective therapeutics. Although it is often assumed thatcore behaviors in children with autism spectrum disorders (ASDs) rarely remit spontaneously, there has been limited investigationof the size of the placebo response in relevant clinical trials. These trials also rely on caregiver and clinical observer reports asoutcome measures. The objectives of this meta-analysis are to identify the pooled placebo response and the predictors of placeboresponse in pharmacological and dietary supplement treatment trials for participants with a diagnosis of ASD. Randomizedcontrolled trials (RCTs) in pediatric ASD, conducted between 1980 and August 2014, were identified through a search of Medline,EMBASE, Web of Science, Cochrane Database of Systematic Reviews and clinicaltrials.gov. RCTs of at least 14 days duration,comparing the treatment response for an oral active agent and placebo using at least one of the common outcome measures, wereincluded. Analysis of 25 data sets (1315 participants) revealed a moderate effect size for overall placebo response (Hedges' g = 0.45,95% confidence interval (0.34–0.56), Po0.001). Five factors were associated with an increase in response to placebo, namely: anincreased response to the active intervention; outcome ratings by clinicians (as compared with caregivers); trials of pharmacologicaland adjunctive interventions; and trials located in Iran. There is a clear need for the identification of objective measures of change inclinical trials for ASD, such as evaluation of biological activity or markers, and for consideration of how best to deal with placeboresponse effects in trial design and analyses.
AMERICAN ACADEMY OF NEUROLOGY MULTIPLE SCLEROSIS FELLOWSHIP CORE CURRICULUM MS Section Education Working Group Introduction Multiple Sclerosis (MS) is the most common demyelinating disease of the CNS and the second most common cause of disability among young adults. The complex and interdisciplinary management issues of the MS patient demand the participation of health care professionals from a variety of intergrated and interactive disciplines, with coordination of care often provided by the neurologist. It is therefore essential for the neurologist to have a thorough grounding in the disease across its broad spectrum, from basic scientific underpinnings including immunopathology to the broad array of its clinical manifestations and patient care needs. Conventional neurologic training usually provides brief encounters in clinic and classroom settings that foster fragmented exposure to the disease. Basic genetics, neuroimmunology and epidemiology are covered in the first two years of medical school, but often without clinical correlation. In the M3 and M4 years, emphasis is placed on clinical presentation and differential diagnosis without exploring the full bench to bedside spectrum of demyelianting disorders. The student clerkship experience with clinical MS care may be limited to interacting for a few days with an MS patient who is hospitalized for an acute and limited care need; such as an IV steroid infusion for an acute MS exacerbation. During residency, diagnosis and therapeutics are more fully explored, but often with limited individual patient continuity for what is a lifelong disorder; and often in departmental partitions that obscure the benefits of coordinated multi-disciplinary disease management. Further, pro-active care approached such as wellness management so actively favored by the MS patient are limited in the university setting. Scheduling demands and time constraints contribute to this fragmentation in MS training of the student and resident physician.With increasing financial pressures, and competition for health care resources, it is important to demonstrate that the optimal care of persons with chronic neurologic conditions such as MS is best implemented by specialist physicians (neurologists) who are most familiar with all of the medical, rehabilitative, psychosocial and vocational needs proper MS disease management requires. The AAN Section on MS Core Curriculum is designed to provide a concise yet comprehensive educational resource about MS, that may be utilized by neurologists to help understand and manage multiple facets of this complicated and interdisciplinary disease process. Basic science facts are presented and will serve as a context for understanding immunopathology and current therapeutic approaches, including immunomodulatory and symptomatic treatments. Clinical phenomenology and diagnostic evaluations are discussed in detail, including unusual presentations and symptoms, and the expanding differential diagnosis of CNS demyelianting disease beyond its "classic" and "conventional" borders. Finally, guidelines for the comprehensive management of persons with MS are provided, using information from each of the health care disciplines that are commonly involved. These latter include nursing, psychosocial and vocational strategies that are often not extensively covered in standard