Reach.reach.gov.pl
A Compendium of Case Studies that helped to shape
the REACH Guidance on Chemical Categories
and Read Across
Edited by
Andrew Worth and Grace Patlewicz
EUR 22481 EN
The mission of IHCP is to provide scientific support to the development and implementation of EU policies related to health and consumer protection. The IHCP carries out research to improve the understanding of potential health risks posed by chemicals, physical and biological agents from various sources to which consumers are exposed.
As a Research Institute, the IHCP contributes to the improvement of scientific knowledge on health care methods and consumer issues.
As a European Institution, the IHCP participates, at an international level and in collaboration with Member States Authorities, in R&D and regulatory actions intended to improve consumer tutelage.
As a European Commission Service, the IHCP acts as an independent advisor for the implementation of risk assessment, monitoring and validation of procedures aimed to insure EU citizens of the use of health and consumer services or products.
European Commission Directorate-General Joint Research Centre Institute IHCP Contact information Address: European Chemicals Bureau TP581 E-mail:
[email protected] Tel.: +39 0332 789566 Fax: +39 0332 786717 http://ecb.jrc.it/QSAR http://www.jrc.cec.eu.int Legal Notice Neither the European Commission nor any person acting on behalf of the Commission is responsible for the use which might be made of this publication. A great deal of additional information on the European Union is available on the Internet. It can be accessed through the Europa server http://europa.eu.int EUR 22481 EN ISSN 1018-5593 Luxembourg: Office for Official Publications of the European Communities European Communities, 2007 Reproduction is authorised provided the source is acknowledged Printed in Italy
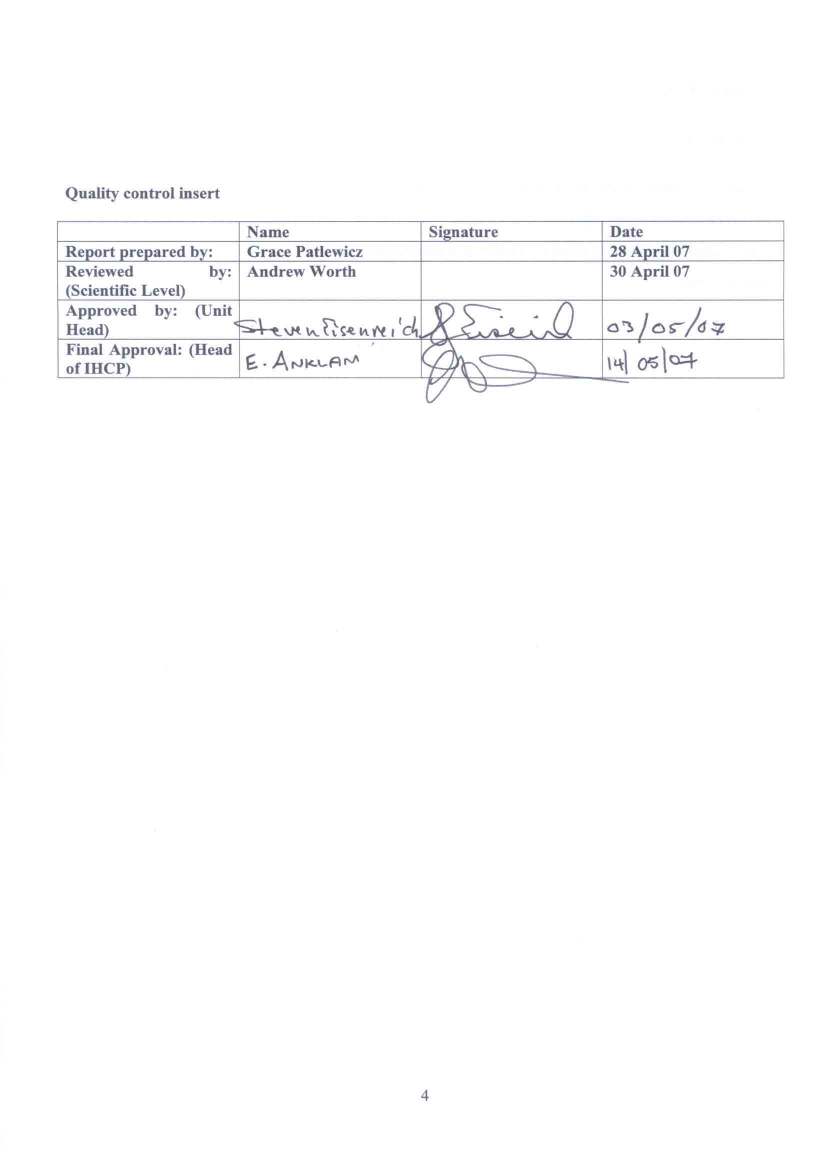
Abstract
This document is a compendium of case studies that were conducted as part of REACH Implementation
Project (RIP) 3.3-2, which was charged with developing technical guidance on the use of chemical
grouping approaches (chemical categories and read-across methods). The lessons and insights from each
of the case studies helped to shape the technical content captured in the resulting guidance document. The
case studies are presented in their original form. For ease of reference, they are grouped into two themes:
current and prospective experiences in the formation and/or use of category approaches.
The editors gratefully acknowledge the contributions of the following members of the RIP 3.3-2 drafting group:
Drafting Group Member
Andrew Worth, Grace Patlewicz
European Commission, Joint Research Centre, ECB
Jane Caley, Daniel Merckel
UK Environment Agency
Ilse Schoeters, Violaine Verougstraete
Rudolf Jäckh, Geoff Hodges
Jan Wilmer, Mike Jeffs, Pierre de Kettenis,
Konrad Gamon, Leo Heezen
Mike Comber, Mike Penman, Barry Simpson
Tala Henry, Mark Townsend
Health & Environment Canada
Ivar Lundbergh, Jörgen Henrikkson
American Chemical Council
Hydrocarbon Solvent Producers Association
Katinka van der Jagt
European Commission, DG Enterprise
European Commission, DG Environment
Contents
Generic Lessons Learned from a Review of Categories presented at OECD
Screening Information Assessment Meetings (SIAM) since April 2004
Review of Categories presented at OECD Screening Information Assessment
Meetings (SIAM) since April 2004 (SIAM 18-22)
Examples of Read-across/Category Approaches discussed by the TC NES
Examples of Read-across/category Approaches discussed by the TC C&L
Case studies on inorganic metal compounds: Read-across examples:
Cd & Cd compounds human health
Cu & Cu compounds human health
Ni & Ni compounds
Pb & Pb compounds
Grouping of Petroleum Substances
A Case Study on Polyols
Possible Application of QSAR Methods to Organic Chemicals
Investigations of Read-across & Grouping, including mechanistic
Considerations and Rationales for Outliers
Approaches to Chemical Categorization: An illustrative Example of
Approaches used by the Fragrance Industry
This document is a compilation of selected case studies that were developed during 2006 by a drafting
group established under the framework of one of the REACH Implementation Projects (RIP 3.3-2) to
develop guidance on the regulatory application of grouping approaches (chemical categories and read-
across). These case studies helped to shape the guidance in the RIP 3.3-2 Technical Guidance Document
(TGD), and are cited directly in the TGD. The case studies represent the findings, views and ideas of their
individual authors at the time of writing, and do not necessarily represent the positions of the individual
organisations with which the authors were affiliated or the consensus view of the drafting group. They are
provided solely for the purposes of transparency and completeness and should therefore not be used in
place of the consensus guidance.
1 Background – the concepts of chemical category and read-across
A chemical category is a group of chemicals whose physico-chemical and human heath and/or
environmental toxicological and/or environmental fate properties are likely to be similar or follow a
regular pattern as a result of structural similarity (or other similarity characteristics).
Within a chemical category, data gaps can be filled in various ways including through the use of read-
across. Here endpoint information for one chemical is used to make a prediction of the endpoint for
another chemical, which is considered to be "similar" in some way (usually on the basis of structural
similarity). In principle, read-across can be applied to characterise physico-chemical properties,
environmental fate, human health effects and ecotoxicity, and it may be performed in a qualitative or
quantitative manner. Read-across can be performed in a one-to-one manner (one analogue used to make
an estimation) or in a many-to-one manner (two or more analogues used).
2 Background – the application of grouping approaches under REACH
Under REACH, testing requirements for individual substances are based on the specific information
requirements shown in Annexes VI to X. As an alternative approach, Annex XI opens the possibility of
evaluating chemicals not on a one-by-one basis, but in groups.
Annex XI contains the following wording for the use of grouping methods (read-across and chemical
"Substances whose physico-chemical, toxicological and ecotoxicological properties are likely to be
similar or follow a regular pattern as a result of structural similarity may be considered as a group,
or ‘category' of substances. Application of the group concept requires that physico-chemical
properties, human health effects and environmental effects or environmental fate may be predicted
from data for reference substance(s) within the group by interpolation to other substances in the
group (read-across approach). This avoids the need to test every substance for every endpoint. The
Agency, after consulting with relevant stakeholders and other interested parties, shall issue
guidance on technically and scientifically justified methodology for the grouping of substances
sufficiently in advance of the first registration deadline for phase-in substances.
The similarities may be based on:
(1) a common functional group,
(2) the common precursors and/or the likelihood of common breakdown products via physical and
biological processes, which result in structurally similar chemicals, or
(3) a constant pattern in the changing of the potency of the properties across the category.
If the group concept is applied, substances shall be classified and labelled on this basis.
In all cases results should:
• be adequate for the purpose of classification and labelling and/or risk assessment,
• have adequate and reliable coverage of the key parameters addressed in the corresponding test
method referred to in Article 13(3)
• cover an exposure duration comparable to or longer than the corresponding test method referred
to in Article 13(3) if exposure duration is a relevant parameter, and
• adequate and reliable documentation of the applied method shall be provided."
Categories of chemicals are selected based on the hypothesis that the properties of a series of chemicals
with common structural features will show coherent trends in their physico-chemical properties, and more
importantly, in their toxicological (human health/ecotoxicity) effects or environmental fate properties.
Common behaviour or consistent trends are generally associated with a common underlying mechanism
of action, or where a mechanism of action exhibits intensity changes in a consistent manner across the
different members of a category.
The use of a category approach will mean that it is possible to identify properties which are common to at
least some members of the category. The approach also provides a basis on which to identify possible
trends in properties across the category. As a result, it is possible to extend the use of measured data to
similar untested chemicals, and reliable estimates that are adequate for classification and labelling and/or
risk assessment can be made without further testing. In addition, knowledge of the expected effects of the
category together with information on use and exposure will help in deciding not only whether additional
testing is needed, but also the nature and scope of any testing that needs to be carried out.
3 Development of technical guidance on the application of grouping approaches
To make the scientific and technical preparations for the implementation of REACH, a number of
Implementation Projects (RIPs) were set up to ensure that appropriate guidance and IT-tools for the
Chemicals Agency, Industry and Regulatory Authorities were developed. The RIP's themselves included
seven main areas. RIP 3 addressed the development of guidance documents for Industry. A subproject of
RIP 3, RIP3.3 focussed on the development of technical guidance for Industry on how to fulfil the
Information Requirements on Intrinsic Properties of substances for REACH. In particular, this project
was aimed at providing a better insight on how to use alternatives to in-vivo data such as (Q)SARs,
chemical categories, in-vitro data as well as how information on exposure could be taken into account.
Task 3 within RIP3.3 addressed the development of guidance of categories and read across; specifically
how to build, justify and use a category including a format to report the "adequate information" to justify
A Drafting Group was set up comprising some 15 contributors from Industry and Regulatory Agencies
including the US-EPA and Health & Environment Canada. The work of the group was coordinated by the
ECB together with the OECD Secretariat.
The objectives of the Task 3 Drafting Group were to develop non-prescriptive guidance that explained
and illustrated:
the commonalities and differences between SARs, read-across and categories (including
how to justify and report qualitative and quantitative read-across
how to build a category (practical details), including examples of qualitative and
quantitative read-across
how to evaluate the robustness and applicability domain of a category
how to justify and report a category proposal
The current OECD guidance on categories as described in the Manual for Investigation of HPV
Chemicals was taken as the starting point. This described the principles and approaches of chemical
categories, but it lacked the detail on the practical steps that would help an end user to formulate a
category, document it and justify it.
The guidance was then developed further based on existing cases involving chemical categories assessed
within the OECD HPV Chemicals Programme, the US HPV Challenge Programme, the EU Existing
Substances Programme, the EU activity on classification and labelling, experience gained from an OECD
Workshop on the development and use of chemical categories held in January 2004 as well as experience
from a number of other case studies some of which were research-based in nature. All case studies were
used to derive and illustrate general principles and approaches. They were not used to redevelop or
change any existing chemical category proposals.
The technical guidance developed was drafted as a standalone document to facilitate parallel submission
to the SIAM under OECD. The guidance comprised a series of chapters that reflected the approach and
workflow as is currently most applied within regulatory programmes. The guidance also addressed the
actual formation of categories for test plan and hazard assessment purposes, and made some preliminary
suggestions about presentation in the form of so-called category reporting formats.
The first set of chapters in the guidance explain relevant concepts namely what a category is, its
mechanistic basis, the close link with (Q)SARs as well as the benefits derived from using a category
approach. In addition the main approaches that are used for data gap filling: read-across, trend analysis
and QSARs are also described. The second set of chapters focus on the practical aspects for forming and
documenting analogue and chemical category approaches in a stepwise procedure as well as some
specific issues that need to be consider for certain types of categories.
This document pulls together the compendium of case studies that were carried out and from which the
lessons and insights shaped the technical content captured in the resulting category guidance. The case
studies are grouped into two themes: current and prospective experiences in the formation and/or use of
category approaches.
The current experiences theme encompasses the generic lessons learnt from a number of categories
presented at OECD Screening Information Assessment Meetings (SIAM) as well as a review of read-
across/chemical categories that have been discussed at EU Existing Substances Programme meetings and
EU classification and labelling group meetings, specifically the Technical Committee of New and
Existing Substances (TC NES) and the Technical Committee of Classification & Labelling (TC C&L). It
also includes examples of grouping approaches used to assess metal compounds, petroleum substances
and polyols – the special types of categories.
Theme 1: Current experiences in the formation and/or use of category approaches
1. Generic Lessons Learned from a Review of Categories Presented at OECD Screening Information
Assessment Meetings (SIAM) since April 2004
2. Review of Categories Presented at OECD Screening Information Assessment Meetings (SIAM)
since April 2004 (SIAM 18-22)
3. Review of recent examples of read-across/category approaches discussed by TC NES
4. Review of recent examples of read-across/category approaches discussed by TC C&L
5. Case studies on inorganic metal compounds: Read-across examples: Cd & Cd compounds human
health, Cu & Cu compounds human health, Ni & Ni compounds, Pb & Pb compounds
6. Grouping of Petroleum Substances
7. A case study on polyols
The prospective experiences theme captures one case study that investigated the feasibility of using
(Q)SAR methods to develop categories. Two further case studies explored mechanistic considerations
when forming groupings for fragrance materials as well as other chemicals.
Theme 2: Prospective experiences in the formation and/or use of category approaches
8. Possible application of QSAR methods to organic chemicals (using phthalate esters)
9. Investigations of read-across & grouping, including mechanistic considerations and rationales for
10. Approaches to Chemical Categorization: An Illustrative Example of Approaches Used by the
Fragrance Industry
Generic Lessons Learned
from a Review of Categories presented at
OECD Screening Information Assessment Meetings (SIAM)
since April 2004 (SIAM 18-22)
Jane Caley and Daniel Merckel
U.K. Environment Agency
Meena Sonowane and Amy Benson
U.S. Environmental Protection Agency
Document Coordinators:
Jane Caley, U.K. Environment Agency
Tala Henry, U.S. Environmental Protection Agency
June 2006
Generic Lessons Learned
[numbers in brackets refer to the Case Study Summary from which the Lesson Learned was derived]
TECHNICAL ISSUES
Category Formation/ Member Inclusion
1. Lesson Learned: When formulating a category, it is desirable to extend a category to include as
many structurally related substances with similar properties as possible for assessing hazard potential. Reasons for this approach include: [1, 2, 4, 5, 7, 9, 12, 13, 14, 18]
a. Assessment of a large number of chemicals as a group can be more efficient and accurate due
to the increased availability of data to the assessor(s).
b. More information and discussion to justify the category is needed for broad categories for
which data is available primarily on mixtures. [11]
c. Closely related categories submitted as "stand alone" documents, (e.g. the phosphonic acids
categories or glycol categories) need to reference the related submissions to provide further confidence in the conclusions for each individual category. However, all of the data from one category should not be directly applicable to another, but individual chemical data may be applied to other categories as analogue data, if appropriate. [1]
d. A category may be justified on more than one basis, for example both a chain length and
metabolic pathway category. Multiple justifications could increase confidence in the category. [4, 5]
e. Including an analogue chemical that has already completed SIDS/SIAM assessment can
increase confidence in a category. However, strong justification for using the analogue data is required.
f. When category members are mixtures, data (measured/modelled) on component compounds
with different carbon chain lengths can be useful supplemental information, even if they are not members of the category.
Suggested Guidance:
1) Clearly articulate that substances covered by the category boundaries need not be HPV
chemicals nor be commercially produced. Allow chemicals other than the ‘sponsored' chemicals to be included in the category as "supporting chemicals", i.e. chemicals that extend the category for the purposes of aiding in the assessment, but which are not officially category members, and hence, additional test data would not be requested as follow-on to the category assessment.
2) Under REACH, different industry sectors may need to co-operate on category assessments. A
challenge will be to convince industry to include all relevant members based on the basic properties excluding use pattern/exposure. There may be different conclusions for different members depending on hazards and uses. Develop incentives or articulate benefits for industry taking this approach, as it would be desirable for the consortium to check with other producers/manufacturers for appropriate support and information. [2,18]
3) Provide guidance for determining if/when chemical use information should be a prevailing
factor in category [vs. single substance] definition. For example, if two chemicals exist only as a mixture resulting from a reaction process, they may be considered differently when
forming a category than if they were also produced and used as discrete chemicals. [see Case Study #18]
2. Lesson Learned: Even when a category justification appears robust, it is possible to reach different
conclusions (and thus, recommendations) for each category member based on biological/toxicological endpoints in different species (e.g. mammalian vs. fish), a trend that is revealed in chemical or toxicological properties, or different use patterns. For example, a trend of increasing aquatic toxicity and bioaccumulation potential with increasing molecular weight could result in the recommendation that the highest molecular weight member is a priority for further work, whereas other category members are not according to a quantitative "cut-off" criterion. This constitutes a "sub-group" approach. [4]
Suggested Guidance: Articulate this point in the guidance.
Read-Across/Extrapolation
3. Lesson Learned: There was disagreement among the workgroup members regarding how and under
what circumstances data from mixtures can be applied to single chemicals. For example: • It is not appropriate to use data for mixtures in a category to represent single substances in a
category (e.g., in the case of biodegradation). [11]
• Surrogate chemicals used to provide supporting data can be a mixture (which can include some/all
of the category members). Composition and purity of the surrogate chemical must be clearly stated and its use as a surrogate clearly justified. Further, the surrogate may not be an appropriate surrogate for all chemicals or endpoints [2].
Suggested Guidance: Develop additional guidance on how and under what circumstances data from
single chemicals or pure homologues can be applied to mixtures and vice versa. A particular case to
address is where the pure homologue, which is not commercially supplied, shows higher or lower
toxicity than the commercial mixture. [9]
4. Lesson Learned: Data gaps may be appropriately filled using modelled data in some cases.
However, models need to be applied to fill data gaps only within the chemical and endpoint context for which they were designed. Further, the models and rationale for their selection for use in filling particular data gaps needs to be transparent to the assessor. [14]
Suggested Guidance: Additional guidance on the use of (Q)SARs and other models for filling data
gaps should be developed, particularly for human health endpoints. [2]
Data Completeness/Adequacy
5. Lesson Learned: It is important to provide a clearly defined category and a well-reasoned category
justification. Identity of category chemicals (and/or analogues) and category justifications are often
unclear or incomplete. [1, 4, 6, 7, 8, 9, 10, 11, 15]
Suggested Guidance: Depending on scope of the Guidance document, discuss specific data
recommendations/requirements or provide examples of categories that illustrate the types of data that
are desired, as summarized below:
• Structural formulae for all category members need to be included for chemical identity and to
demonstrate similarities in chemical structure for categories (partly) based on structure. [6]
• When using a chemical that has been assessed previously (i.e. agreed in a previous SIAM) as a
supporting chemical, the documents need to be clear about which chemical(s) are ‘category members' and which chemical(s) are ‘supporting chemical(s). [8]
• Purity/impurity information of the tested substance needs to be included in the individual robust
summaries (in the SIDS Dossier/IUCLID), when an "other" tested substance is used in the study and the specific information is not covered under the identity section (in the SIDS programme sections 1.1-1.4 of the Dossier). [8]
• For categories that include mixtures, detailed product composition information. For example, for
category with mixtures with different alkyl chain lengths, physicochemical property information (e.g. proportion of alkyl chain lengths, degree of branching, water solubility) is needed to determine how the components of the mixture relate to each other and other category members as well as to identify or discount trends. [7, 9]
• For categories that include both mixtures and pure homologues as analogues, composition of the
tested substances needs to be explicitly discussed. [9]
• Toxicity values for individual category members should be provided in a tabulated format and
discussed in the text whenever feasible and possible. Discrete data should not be summarized as ranges unless absolutely necessary (e.g., mixtures with multiple homologues as analogues). [10]
• For categories that are formed on the basis of relevant trends (i.e. ‘continuum categories'). If data
indicate there is any trend, the data and the trend need to be described. In addition, the direction and magnitude of the trend should be described. [4, 8]
• For categories based on common metabolic pathways, include appropriate data or discussion on
how these chemicals are related structurally, functionally, and metabolically. [15]
Chemical-Specific Considerations
6. Lesson Learned: Experience with certain chemical categories provide lessons related to the types of
chemicals included in the category. These lessons are not universally applicable to all categories, but should be considered when creating and assessing categories of related chemicals.
Suggested Guidance: Depending on scope of the Guidance document, discuss specific chemical-
related issues or provide some examples of categories that illustrate the considerations summarized
below:
a. For categories including ionisable compounds several properties related to behaviour of the
ionisable compounds need be considered, including:
i. Water solubility and dissociation behaviour in aqueous solution should be similar or
follow a predictable trend among category members. [1, 5, 19]
ii. pH effect on behavior in aqueous solution and toxicological properties (e.g., irritation).
iii. Effect(s) of the counter-ion. It is possible that the counter-ion(s) may pose hazards of
greater concern than the common cation or anion on which the category is based (e.g., metal counter-ions that are inherently hazardous on their own). Under such circumstances, it may be of limited utility to group and assess substances by the component which is expected to have the least effect. [1, 5]
iv. Differentiation between toxicity stemming from pH effects alone and a combination of
pH effects and inherent toxicity. Comparison of unbuffered test results with tests results that include pH buffering (in accordance with OECD test guidelines) is preferred. [6, 19]
b. Functionally or structurally related salts and acids can be included in a single category if their
behaviour in solution, environmental and toxicological properties are similar. [17]
c. For categories that include mixtures with different alkyl chain lengths, physicochemical
property information (e.g., distribution of alkyl chain lengths, water solubility) is needed to determine how the components of the mixture relate to each other and other category members as well as to identify or discount trends. [9]
d. For categories that include reactive chemicals, the reaction/degradation products must be of a
similar nature for each member of the category for the category justification to be credible. [16]
PROCEDURAL ISSUES TO CONSIDER DEVELOPING GUIDANCE FOR
1. When using a chemical that has been assessed previously (i.e. agreed in a previous SIAM) as a
supporting chemical, conclusions drawn based on the supporting chemical must be consistent with conclusions drawn in the earlier assessment/SIAM. Development of a cross-checking/referencing tool is recommended (This could also apply under REACH when using supporting data from a chemical that has already been registered and evaluated). [4, 8]
2. New information generated on a chemical that has been assessed in an earlier SIAM should be
included in the dossier being presented, if that information is relevant to address a particular SIDS endpoint for any/all chemical(s) in the current category dossier. Previously submitted information generated on a chemical that has been assessed in an earlier SIAM needs to be drawn from the previous dossier. [8]
3. In some cases, individual chemicals are HPV only as part of a mixture. Assessment of the mixture
does not qualify the individual isomers as having gone through the SIDS/SIAM process, if their data have been used only as analogous information. However, if the individual components of the mixture become HPV apart from the mixture, they will need to be sponsored separately. [18]
4. Data matrices should routinely be included in submissions to show where data are available and
where read-across is being applied to the category members. [13]
5. For "continuum" categories, discuss the assessment according to individual SIDS endpoints organized
by the trend in toxicity of category members. Summaries including graphic illustrations of the trends in data (e.g. linearly increasing aquatic toxicity with chain length) are very useful. This approach worked well for a small (7 substances) and relatively data rich category. [20]
EXAMPLES OF "GOOD" CATEGORY SUBMISSIONS
Based on the workgroup reviews, the following categories were deemed good examples by virtue of their
well reasoned and supported category formation, consideration of chemical properties and hazard in
category formation, data completeness, and overall presentation:
1. Category Name: Epoxidized Oils and Derivatives (EOD) - SIAM: 22 [15]
2. Category Name: Diethylene Glycol Ethers - SIAM: 21 [13]
3. Category Name: High Molecular Weight Phthalate Esters – SIAM: 19 [Not reviewed for this
Review of Categories presented at
OECD Screening Information Assessment Meetings (SIAM)
since April 2004 (SIAM 18-22)
Environmental Reviews:
Jane Caley and Daniel Merckel
U.K. Environment Agency
Human Health Reviews:
Meena Sonowane and Amy Benson
U.S. Environmental Protection Agency
Document Coordinators:
Jane Caley, U.K. Environment Agency
Tala Henry, U.S. Environmental Protection Agency
June 2006
Case Study #1 (Limited Health & Full Environment Review)
Category Name: 3 related categories:
ATMP and salts -Phosphonic Acid Compounds Group 1
HEDP and salts - Phosphonic Acid Compounds Group 2
DTPMP and salts - Phosphonic Acid Compounds Group 3
SIAM: 18
Category members:
Sub-category & Chemical name
ATMP and salts -Phosphonic Acid Compounds Group 1
Amino tris(methylenephosphonic acid) (ATMP)
Amino tris(methylenephosphonic acid), xNa Salt
Amino tris(methylenephosphonic acid), Na Salt
Amino tris(methylenephosphonic acid), 2Na Salt
Amino tris(methylenephosphonic acid), 3Na Salt
Amino tris(methylenephosphonic acid), 4Na Salt
Amino tris(methylenephosphonic acid), 5Na Salt
Amino tris(methylenephosphonic acid), 6Na Salt
HEDP and salts - Phosphonic Acid Compounds Group 2
1-Hydroxy-1,1-ethane-diphosphonic acid (HEDP)
1-Hydroxy-1,1-ethane-diphosphonic acid, xNa Salt
1-Hydroxy-1,1-ethane-diphosphonic acid, Na Salt
1-Hydroxy-1,1-ethane-diphosphonic acid, 2Na Salt
1-Hydroxy-1,1-ethane-diphosphonic acid, 3Na Salt
1-Hydroxy-1,1-ethane-diphosphonic acid, 4Na Salt
1-Hydroxy-1,1-ethane-diphosphonic acid, 5Na Salt
1-Hydroxy-1,1-ethane-diphosphonic acid, xK Salt
1-Hydroxy-1,1-ethane-diphosphonic acid, K Salt
1-Hydroxy-1,1-ethane-diphosphonic acid, 2K Salt
1-Hydroxy-1,1-ethane-diphosphonic acid, 3K Salt
1-Hydroxy-1,1-ethane-diphosphonic acid, 4K Salt
1-Hydroxy-1,1-ethane-diphosphonic acid, 5K Salt
DTPMP and salts (Phosphonic Acid Compounds Group 3) Diethylene triamine penta(methylene phosphonic acid)
Diethylene triamine penta(methylene phosphonic acid), xNa Salt
Diethylene triamine penta(methylene phosphonic acid), Na Salt
Diethylene triamine penta(methylene phosphonic acid), 2Na Salt
Diethylene triamine penta(methylene phosphonic acid), 3Na Salt
Diethylene triamine penta(methylene phosphonic acid), 4Na Salt
Diethylene triamine penta(methylene phosphonic acid), 5Na Salt
Diethylene triamine penta(methylene phosphonic acid), 6Na Salt
Diethylene triamine penta(methylene phosphonic acid), 7Na Salt
Diethylene triamine penta(methylene phosphonic acid), 8Na Salt
Diethylene triamine penta(methylene phosphonic acid), 9Na Salt
Diethylene triamine penta(methylene phosphonic acid), 10Na Salt
Brief description of category:
These are 3 closely-related categories of alkyl phosphonic acids and their sodium or potassium salts. The
different salts are prepared by neutralising the acid to a specific pH. The effect of the counter-ion
(sodium/potassium) is assumed to be insignificant and the category members will be fully dissociated in
dilute aqueous solutions.
The dominant characteristic across all three categories is the presence of several phosphonic acid
functions, which can ionise in aqueous solution to phosphonate anions. All category members are highly
adsorbing, highly water soluble and have similar use patterns. They all chelate metals ions so they have
the potential to disrupt bioavailable concentrations of metallic cations in the blood of fish and
invertebrates and to cause nutrient depletion to algae and plants by complexing trace metal cations.
Direct read-across is used for most endpoints. Dermal and irritation studies are considered separately for
the acid and salts.
The ATMP category was extended to cover the single sodium salt, although this substance is not
commercially produced and has no CAS number.
Category issues raised during comment/SIAM:
The main concern was justification of the conclusion reached for genotoxicity (human health issue).
There was also a lot of discussion around the use of data from Industrial Bio-test Laboratories Inc, who
were investigated by the US FDA for falsification of results of certain studies, but this is not directly
relevant to the category justification.
Generic lessons learned:
• For categories including ionisable compounds, the effect of the counter-ion needs to be considered. It
is possible that the counter-ion(s) may pose hazards of greater concern than the common cation or anion on which the category is based (e.g., metal counter-ions that are inherently hazardous on their own). Under such circumstances, it may be of limited utility to group and assess substances by the component which is expected to have the least effect. In other cases, it may be concluded that effects of the counter-ion are insignificant and therefore need not be taken into account in the assessment.
• When comparing acids and their salts, differences arising from pH effects should be considered. For
example, skin and eye irritation are likely to be different for an acid compared with its salt.
• It is possible to extend a category to include substances which are not commercially produced but
which are covered by the category boundaries.
• For closely related categories, (in this case, structurally related phosphonic acids) it can be helpful to
include a link between them to provide further confidence in the conclusions for each individual category.
Lessons learned specific to the category:
• Health: Category members (acids vs. salts) show a difference in skin and eye irritation effects
connected with pH.
• Environment: The discussion of nutrient limitation effects was supported by the use of data from
DTPMP and other chelating agents, including EDTA. It was helpful to widen the category approach to 3 sub-categories.
Case Study #2 (Health & Environment Review)
Category Name: Ethylene Glycol
SIAM: 18
Category members:
Diethylene glycol
Triethylene glycol
Pentaethylene glycol
Brief description of category:
A chain length category based on the oxyethylene unit, (CH2 CH2O). Each category member has a
structure HO(CH2 CH2O)nH where n = 1, 2, 3, 4, or 5. The category boundary appears to be at n = 6-8,
when absorption from ingestion decreases and certain physicochemical attributes change significantly and
the materials start to become solids.
Polyethylene Glycol 200 (PEG 200) is used to provide supporting data, mainly for the health assessment.
This substance is a mixture with n = 2-8 with an average value of 4. It is used to fill quantitative data gaps
for repeated dose toxicity and developmental toxicity, and provides supporting data for acute toxicity and
carcinogenicity.
Each category member has a different use pattern, some of which are not interchangeable. For example,
the major use of Ethylene glycol is the manufacture of polyethylene terephthalate (PET) whereas the
major use of Triethylene glycol is natural gas dehydration.
QSARs are used to support the toxicity and ecotoxicity data.
Category issues raised during comment/SIAM:
For human health, QSAR estimations were included. However, several comments suggested that there
was not enough discussion of the models in the Dossier; thus, the commenters suggested the estimations
be included only in an Appendix.
PEG 200 seems to have a different genotoxicity profile compared with most of the members of the
category, although tetraEG did have some equivocal chromosomal aberrations data.The submitter
proposed additional genetic toxicity testing (i.e., a CHO/HGPRT assay on pentaEG). However, given the
availability of data for the category members, the additional test did not seem necessary. If the testing
was still to be done, it should be described as a post-SIDS activity.
The acute Daphnia toxicity of TetraEG does not appear to fit the trend in the ecotoxicity data, showing
higher toxicity than expected. However, new data on brine shrimp confirmed low toxicity across the
group.
Generic lessons learned:
• Category members need not have similar uses. Under REACH, different industry sectors may need to
co-operate on category assessments.
• Surrogate chemicals used to provide supporting data can be a mixture (which can include some/all of
the category members). Composition and purity of the surrogate chemical must be clearly stated and its use as a surrogate clearly justified. Further, the surrogate may not be appropriate surrogate for all chemicals or endpoints.
• Additional testing should be described as post-SIDS work if all SIDS endpoints have been met. If all
endpoints have not been met, the case should not be presented at a SIAM.
• Because QSAR models for human health endpoints are not as accepted as they are for ecotoxicity
endpoints, additional guidance on the use of QSARs for filling data gaps should be developed.
Lessons learned specific to the category:
• Health: Other than tetraEG, which did have some equivocal chromosomal aberrations data, PEG 200
had a different genotoxicity profile than most members of the category and therefore, is not a good surrogate for this endpoint.
• Environment: None
Case Study #3 (Health Review Only)
Category Name: Propylene Glycol Phenyl Ether (isomers)
SIAM: 18
Category (chemical) members:
Propylene Glycol Phenyl Ether
alpha isomer, secondary alcohol
beta isomer, primary alcohol
Brief description of chemical (not a true category):
Monopropylene glycol ethers may exist in two isomeric forms, alpha and beta. The alpha form (a
secondary alcohol) is thermodynamically favoured during synthesis and accounts for the majority of the
glycol ether mass. The beta form (a primary alcohol) is an impurity in the synthesis process.
All tests were conducted on the commercial mixture, which contains >85% alpha isomer and <15% beta
isomer. The individual isomers are not separated nor produced as individual chemicals. A category
approach was not used since data were available on the commercial mixture.
Category issues raised during comment/SIAM:
There was some confusion regarding which chemical(s) were being sponsored versus which chemical(s)
were tested. This confusion stems from the fact that the commercial product is commonly referred to as
CAS# 770-35-4 (for the alpha isomer) and the CAS# assigned to the commercial mixture (41593-38-8) is
not commonly used. The commercial product is listed under both CAS#s because modern production
methods result in alpha isomer content in excess of 85% and beta isomer content less than 15% and the
individual isomers are not separated nor produced as individual chemicals.
Additional clarity on the identity of the commercial substance and the substance tested were suggested,
for example:
- specifying typical concentration range of alpha- and beta-isomers
- specifying which compound(s) were being sponsored versus which compound(s) were tested (i.e., the
alpha isomer CAS number sponsored, as in the SIAP, but testing was conducted using the commercial
mixture).
- establishing, in the documents, that the toxicity, physicochemical and other relevant properties of the
various forms are similar and are suitable for consideration as a single set or that the amount of the beta
isomer is insignificant compared to the alpha isomer (either should be adequately supported with relevant
data).
Generic lessons learned:
• Information for SIDS cases with multiple CAS numbers that do not constitute a category need to be
very clearly described so it does not appear that a category approach has been used, when it has not.
Lessons learned specific to the category:
• Health: None; category approach was not used.
• Environment: Not Reviewed
Case Study #4 (Health and Environment Review)
Category Name: Short Chain Alkyl Methacrylate Esters
SIAM: 18
Category members:
MMA; Methacrylic Acid, Methyl
(Reference
chemical for Propenoate
category)
EMA; Methacrylic Acid, Ethyl
Methacrylate 97-63-2
i-BMA; Methacrylic Acid, I-Butyl
Methacrylate 97-86-9
Monomer; Iso-Butyl, 2-methyl-2-Propenoate
n-BMA; Methacrylic Acid, n-Butyl
Methacrylate 97-88-1
Monomer; n-Butyl, 2-methyl-2-Propenoate
2-EHMA; Methacrylic Acid, 2-
Brief description of category:
The category is defined as methacrylate esters of straight and branched C2 to C8 alcohols. A chain length
category based on the methacrylate ester functional group. This is a standard chain length category, with
trends of increasing Log Kow and boiling points with increasing molecular weight and decreasing water
solubility and vapour pressures with increasing molecular weight.
MMA was used as a robust reference chemical for the category.
Methyl methacrylate (MMA), the C1 ester, is data rich and already has an agreed SIAR (SIAM #11) and
E.U. Risk Assessment.
Based on their clear structure activity relationship with respect to environmental toxicity, distribution and
fate, and metabolism and toxicity in mammalian systems, these chemicals are considered together as a
category.
• Health:
o The category is also a metabolic pathway category since short-chain alkyl-methacrylate esters
are very rapidly metabolized by non-specific carboxylesterases to methacrylic acid and the structurally corresponding alcohol in several tissues.
o The half-life of disappearance of the parent ester from the body is in the order of minutes.
o Data on metabolites were used for the repeated toxicity and developmental endpoints.
• Environment:
o Toxicity to aquatic organisms increases with increasing lipophilicity and molecular weight.
o EPIWIN/ECOSAR estimated values were not used to support ecotoxicity data.
o One category member, 2-EHMA is recommended as a candidate for further work for the
environment, due to its potential for bioaccumulation and aquatic toxicity.
Category issues raised during comment/SIAM:
A stronger justification needs to be provided for using data for the reference substance, methyl
methacrylate (MMA), for the four category members that lack data. Close structural analogues, structural
activity trends, and common metabolism pattern of the parent ester to methacrylic acid and corresponding
alcohol have been used to address the endpoints for those chemicals lacking these data. In particular,
SIAM wanted a more detailed justification of the use of a category approach and the use of MMA as a
predictor for carcinogenicity for the category members.
A concern was raised regarding the use of supporting data on an analogue chemical that was assessed at a
previous SIAM. The issue was whether the recommendations for the category members need to be
consistent with the supporting analogue chemical. In this case, the analogue data was not heavily relied
upon for the category members and the conclusions for the category members were based on data and
properties that were different from the analogue chemical. Thus, the conclusion for the analogue (MMA)
is not appropriate for these esters.
Generic lessons learned:
• When formulating a category, industry should look for other structurally-related substances which
may fit, not just those substances produced by the consortium.
• A category may be justified on more than one basis, for example both a chain length and metabolic
pathway category.
• The category chemicals need to be brought together to identify the trends in behaviour. If data
indicate there is trend in toxicity, for example, it needs to be stated accordingly.
• Including a chemical that has already been evaluated/assessed can increase confidence in a category.
However, strong justification for using analogue data is needed.
• Even when category justification appears robust, it is possible to reach different conclusions for each
category member based on biological/toxicological endpoints in different species (e.g. mammalian vs. fish) or based on a trend that is revealed in chemical or toxicological properties. (In this case, this is due to a trend of increasing aquatic toxicity and bioaccumulation potential with increasing molecular weight ended with the conclusion that the highest molecular weight member is a priority for further work, whereas the other members are not.)
• Should consistency should be maintained in conclusion/recommendation for an analogue chemical
that has been discussed in earlier the SIAM if the analogue is used to support the majority of category chemicals and when no data are available on any of the category chemicals.
Lessons learned specific to the category:
• Health: A stronger justification needs to be provided for using data for the reference substance,
methyl methacrylate (MMA), for the four category members where they lack data.
• Environment: One category member, 2-EHMA is recommended as a candidate for further work for
the environment, due to its potential for bioaccumulation and aquatic toxicity (i.e., because it represented a "high-end" of a trend of increasing toxicity and bioaccumulation potential).
Case Study #5 (Environment Review Only)
Category Name: Gluconates
SIAM: 18
Category members:
Chemical
Structural formula
Name
D-Gluconic acid
Glucono-delta-lactone 90-80-2
Sodium gluconate
Calcium gluconate
Potassium gluconate
Brief description of category:
The category is an ‘acid & salt category ‘and focuses on the gluconate anion. Gluconic acid and its
mineral salts freely dissociate to the gluconate anion and the respective cations. Glucono-delta-lactone
(GDL), the 1,5-inner ester of gluconic acid, is formed from the free acid by the removal of water. On the
basis of these spontaneous chemical rearrangements, glucono-delta-lactone, gluconic acid and its sodium,
calcium and potassium salts are considered as a category, with all members sharing the same
representative moiety, the gluconate anion.
The assessment focuses on the gluconate anion and does not deal with specific effects of the cations.
However, for repeated dose toxicity, potential side effects were attributed to high doses of cation intake.
Gluconic acid also has another inner ester, the 1,4-lactone, which was not included in this category. It is
not stated why this lactone was excluded but following questions from other member countries, it was
stated that the 1,4-lactone is of no commercial interest.
Category issues raised during comment/SIAM:
It would not be possible to extend the group to other substances where the counter ion may be more toxic.
Indeed, in some ways it seems less useful to group substances by the component which is expected to
have the least effect.
Further justification required for inclusion of the glucono-delta-lactone, taking into consideration
metabolic fate and proportion of the metabolic product. [This question highlights that this is also a
metabolic category].
Generic lessons learned:
• For categories including ionisable compounds, the effect of the counter-ion needs to be considered. It
is possible that the counter-ion(s) may pose hazards of greater concern than the common cation or anion on which the category is based (e.g., metal counter-ions that are inherently hazardous on their own). Under such circumstances, it may be of limited utility to group and assess substances by the component which is expected to have the least effect. In other cases, it may be concluded that effects of the counter-ion are insignificant and therefore need not be taken into account in the assessment.
• A category may be justified on more than one basis, for example both a chain length and metabolic
pathway category.
• Substances which are good analogues for a category, should be included to strengthen a category.
Such analogues need not be HPV chemicals (i.e. have no commercial interest).
Lessons learned specific to the category:
• Health: For category members with a common cation or anion, the effect(s) of the counter-ion needs
to be considered and discussed.
• Environment: For category members with a common cation or anion, the effect(s) of the counter-ion
needs to be considered and discussed.
Case Study #6 (Health & Environment Review)
Category Name: Maleic Acid and Malic Anhydride
SIAM: 18
Category members:
Maleic anhydride
Brief description of category:
Category is based on metabolic series approach. Maleic anhydride is readily hydrolyzed to maleic acid
under aqueous conditions and thus these two chemicals are considered analogous. The only difference is
that maleic hydride has immediate effects at the site of contact due to the exothermic reaction from the
hydrolysis of maleic anhydride to maleic acid and also has a potential to form haptens by acylating with
amino acids, resulting in an immunological response.
Maleic acid is an intermediate in the production of maleic anhydride. Most of the maleic anhydride is
used in unsaturated polyester resins and smaller amounts are used in production of fumaric and malic
acid, as additives, copolymers, and agricultural chemicals, etc.
Mammalian toxicity studies (reproductive, developmental, and in vivo chromosomal aberrations)
conducted with maleic anhydride are used to infer toxicity of maleic acid. Ecotoxicological study results
reflect pH-dependant toxicity because of maleic anhydride being converted to and measured as maleic
acid.
QSARs were not used to support any toxicity and ecotoxicity data.
Category issues raised during comment/SIAM:
Maleic acid has two isomers, cis and trans. However, information is available only on the cis isomer and
no information was available on the trans isomer whether it exists as a commercial product or has been
isolated as a testing substance.
For the environmental ecotoxicity testing concern regarding the origin of toxicity seen in the maleic
anhydride tests was raised; was this due to low pH (reaction of parent with water to form maleic acid as
discussed above) only, or was it a combination of pH and the chemical's intrinsic toxicity? Comparison
with buffered tests indicated that pH alone was the cause.
Generic lessons learned:
• Structural formulas for category members need to be included.
• For categories including ionisable compounds, either as original category members or degradation
products it is essential to differentiate between toxicity stemming from pH effects alone and a combination of pH effects and inherent toxicity. Comparison of unbuffered test results with tests results that include pH buffering (in accordance with OECD test guidelines) is preferred.
Lessons learned specific to the category:
• Health: None
• Environment: Maleic anhydride rapidly hydrolyses to the category member maleic acid, so it is
essential to differentiate between toxicity stemming from pH effects alone and a combination of pH
effects and inherent toxicity. In this case this was satisfactorily done by comparison of results from aquatic tests performed with and without pH buffering.
Case Study #7 (Health Review Only)
Category Name: Higher Olefins
Category members:
Brief description of category:
The category consists of nine members with carbon numbers from C6 to C18 mono-olefins (C6, C7, C8,
C9, C10, C12, and C10-13 internal olefins and C16 and C18 linear olefins). The internal olefins are
predominantly linear with a small amount of branched material as impurities. The data indicate that
changing carbon number, location of the double bond or addition of branching does not alter the
mammalian health and biodegradation endpoints and helps to indicate increasing or decreasing trends for
ecotoxicity. The data indicate an increasing or decreasing trend from the shortest alpha or internal olefin
(C6) to the longest alpha or internal olefin for various physicochemical properties and ecotoxicity
endpoints, whereas there is no apparent critical differences across category members for biodegradation
and health effect endpoints. Therefore, data for linear alpha olefins and linear and branched internal
olefins are used to characterize the human and environmental health hazards for this category.
QSARs were used to support ecotoxicity data.
Category issues raised during comment/SIAM:
Many more higher olefins exist in the OECD HPV Database and since data for some non-HPV substances
were used as supporting data for this category, the question was raised why not bring forward such other
non-HPV chemicals (29 are listed in the comment) under this category. Although this concept appears
well-founded, the chemicals in this category are sponsored by at least one member company of the
consortium. Because of the substantial amount of work that is involved in preparing the necessary
documents, if the producers of the other chemicals are willing to sponsor their chemicals, the Higher
Olefins consortium is willing to work with other higher olefin producers.
Identity of category members needs to be clearly defined as to the proportion of branched components
and degree of branching.
Generic lessons learned:
• All category members need to be clearly identified, including proportion of branched components and
degree of branching.
• Inclusion of other structurally related chemicals with similar properties that are not included in the
proposed category will be beneficial in assessing hazard potentials of a large number of chemicals as
a group. The consortium needs to check with other producers/manufacturers for providing appropriate support and information.
Lessons learned specific to the category:
• Health: None
• Environment: Not Reviewed
Case Study #8 (Health & Environment Review)
Category Name: Monoethylene Glycol Ethers
SIAM: 19
Category members:
Ethylene glycol propyl ether (EGPE)
Ethylene glycol butyl ether (EGBE)
Ethylene glycol butyl ether acetate (EGBEA)
Ethylene glycol n-hexyl ether (EGHE)
Brief description of category:
All category members have similar molecular structures with a backbone of glycol ether. The only
difference in these molecules is in the alkyl functional groups —propyl, butyl, or hexyl, and whether or
not the hydroxyl group is free or acetylated. Ethylene glycol butyl ether acetate—the acetylated glycol
ether—metabolizes rapidly in vivo to the corresponding ethylene glycol butyl ether, making it adequate
for the inclusion in the category and thus it is reasonable to extrapolate mammalian toxicity data for the
corresponding ether to address the data gaps for the acetate. However, in the environment, conversion of
ethylene glycol butyl ether acetate is not expected to be rapid. For this reason, ecotoxicity data for the
corresponding ether cannot be extrapolated to the acetate. Thus in addition to the glycol ether data, all
ecotoxicity data for the acetate have been provided.
Use patterns for all category members appear to be the same, with associated emissions predicted to be
similar.
EPIWIN/ECOSAR estimated values have been used to support ecotoxicity data.
Category issues raised during comment/SIAM:
EGBE data are used throughout the assessments to support the category member EGBEA, for which there
were data gaps. However, it is not formally a part of the category as it already has a SIDS dossier and
SIAR that were agreed upon at SIAM 6 (sponsored by Australia). Data used for the substance needs only
to be drawn from this source.
Suggestions were made to update EGBE dossier with available new information e.g., new cancer data and
new ecotoxicity information; however, the purpose of this dossier is not meant to be an update for EGBE.
In addition, carcinogenicity is not a SIDS endpoint and as mentioned in the category justification, EGBE
ecotoxicity data are not needed for EGBEA because aquatic toxicity of EGBE is different from that of
EGBEA and could not be extrapolated to EGBEA. All endpoints for EGBEA have addressed by
experimental data. It was suggested that an inclusion of the recently conducted repeated-dose (inhalation)
toxicity study on EGBE should not be reviewed since the EGBE dossier was agreed at SIAM 6.
However, this study was conducted by more relevant route of exposure and therefore, was appropriate to
include in this dossier.
Metabolism of EGBEA was partially explained in the SIAR based on EGMEA and EGEEA; a suggestion
was made to include those robust summaries in the IUCLID. However, general information about other
glycol ethers that are not the category members would not have made the category robust.
Clarification was suggested in the statement of how acute toxicity changes with molecular weights (e.g.,
oral LD50 values in rats for all category members range from 739 (EGHE) to 3089 mg/kg bw (EGPE),
with values increasing with decreasing molecular weight).
When using data for a chemical previously assessed at the SIAM, it needs to be verified whether the same
studies drew similar conclusions. For example, NOAELs derived for reproduction toxicity should be
similar in both dossiers.
When documenting uses, include specific uses of individual category chemicals, if available, and varied.
The purity/impurity information of the marketed substance needs to be indicated in Dossier sections 1.1-
1.4. This section encompasses all synonyms. When the test substance is indicated as "other", then it
needs to be specified in the appropriate robust summary and its purity/impurity information needs to be
included.
Generic lessons learned:
• When making statements regarding how toxicity varies among the category members, be more
specific, e.g., provide information if there is a trend in toxicity and molecular weight, etc.
• Purity/impurity information of the tested substance needs to included in the SIDS dossier, when the
"other" tested substance is used in the study and the specific information is not covered under sections 1.1-1.4.
• When using a chemical that has been assessed previously (i.e. agreed in a previous SIAM) as a
supporting chemical, the documents need to be clear about which chemical(s) are ‘category members' and which chemical(s) are ‘supporting chemical(s).
• When using a chemical that has been assessed previously (i.e. agreed in a previous SIAM) as a
supporting chemical, conclusions drawn based on the supporting chemical must be consistent with conclusions drawn in the earlier assessment/SIAM. OECD needs to develop cross checking/referencing tool (This could also apply under REACH when using supporting data from a chemical which has already been registered and evaluated).
• New information generated on a chemical that has been assessed in an earlier SIAM should be
included in the dossier being presented, if that information is relevant to address a particular SIDS endpoint for any/all chemical(s) in the current dossier. Previously submitted information generated on a chemical that has been assessed in an earlier SIAM need not be included as a category member and data used for the substance needs only to be drawn from the previous dossier.
Lessons learned specific to the category:
• When structurally related ethers and ether acetates are proposed as a single category, data (trends)
should be assessed to determine if splitting into ether and acetate sub-categories is more appropriate for relevant endpoints/sections.
• Health: None
• Environment: An example of the "sub-group" approach within a category for specific endpoints. As
described, all members are ethylene glycols; three category members are glycol ethers but one is a glycol ether acetate. The acetate was considered separately from the ethers, as the acetate group is only slowly hydrolysed at environmental pH to the alcohol. This approach seems warranted as the departure from the common alcohol functionality displayed by the majority of category members is fairly great (alcohol to acetate), and in addition the acetate appeared to be more acutely toxic.
Case Study #9 (Health & Environment Review)
Category Name: Linear Alkylbenzene Sulfonates
SIAM:20
Category members:
Decylbenzene sulfonic acid, sodium salt
Dodecylbenzene sulfonic acid, sodium salt
Tridecylbenzene sulfonic acid, sodium salt
Undecylbenzene sulfonic acid, sodium salt
C10-16 Monoalkylbenzene sulfonic acid, sodium salt
C10-13 Alkylbenzene sulfonic acid, sodium salt
C10-14 Alkyl deriv benzene sulfonic acid, sodium salt
C10-14 Monoalkylbenzene sulfonic acid, sodium salt
C10-13 Alkyl deriv benzene sulfonic acid, sodium salt
4-C10-13-sec Alkyl deriv benzene sulfonic acid, sodium salt
Brief description of category:
The LAS molecule contains an aromatic ring sulfonated at the para position and attached to a linear alkyl
chain at any position other than at the terminal carbons. The alkyl chain usually has 10 to 14 carbon
atoms; and they are 87 to 98% linear. Although commercial LAS consists of more than 20 unique
components, the ratio of the various homologues and isomers (which represent different alkyl chain
lengths and aromatic ring positions on the linear alkyl chain) is fairly constant in current products; the
weighted average carbon number of the alkyl chain based on production volume per region is between
11.7-11.8.
LAS is a category because of the similarity of the different mixtures, the commercial uses, the fate
endpoints, the health effects, and environmental effects. It is the primary cleaning agent used in many
laundry detergents and cleaners, and is present at concentrations up to 25 percent in consumer products,
and up to 30 percent in commercial products, with the exception of one reported product, which is a 45
percent concentrated solid form that is mechanically dispensed into diluted solution for dishwashing.
As expected with a chain length category, trends in properties are seen with increasing alkyl chain length.
For example, Kd values for LAS increase with increasing alkyl chain length of LAS homologues and
measured fish BCFs generally increase with increasing alkyl chain length. Aquatic toxicity is greater for
LAS with longer carbon chains. This is supported by QSAR predictions of aquatic toxicity. The QSARS
for acute toxicity were used to normalize the experimental NOEC values to NOECs for C11.6 LAS, the
average commercial mixture. [Note – a similar approach was used for the ICCA assessment of Long
Chain Alcohols].
Category issues raised during comment/SIAM:
Two members of the category are not HPVs. It was suggested that they not be included, however, the
chemicals were retained because convention in the SIDS program has been that non-HPV chemicals can
be used in categories to fill certain endpoints.
For substances that are mixtures with different alkyl chain lengths, physical property information (and the
trends in physicochemical properties) for the different chain lengths is desirable. This issue applies to
categories as well as to mixtures.
Additional composition information was needed (e.g., distribution of alkyl chain lengths) to determine
how the members relate to each other within the category.
There was a request for more matrices to determine read-across. However, because the mixtures are very
similar to each other, the data matrices were considered unnecessary.
The category members are mixtures, whereas some data are available for more pure compounds. The
difference between the structures of the test substances and the category members needs to be discussed.
Aquatic toxicity data is available for both commercial products (mixtures) and pure C13 and C14
homologues. The pure homologues showed higher toxicity than the commercial mixtures but data for the
pure homologues was not used to drive the recommendation since they are not commercially supplied.
Member countries requested further justification of the approach for normalising experimental NOECs
from aquatic toxicity tests to NOECs for the C11.6 average LAS commercial mixture.
Generic lessons learned:
• Category members need not be HPV chemicals. Good analogue chemicals strengthen the category
(i.e. read-across).
• As much detail on product composition (as well as physicochemical properties) as possible needs to
be provided so that an independent assessment of the category can be made.
• When category members include both mixtures and pure homologues, the differences between the
structures of the test substances and the category members need to be clearly presented.
• For substances that are mixtures with different alkyl chain lengths, physical property information
(e.g., distribution of alkyl chain lengths) is needed to determine how the members relate to each other within the category (e.g. identify or discount trends). This issue applies to categories as well as to mixtures.
• Additional guidance is required on how data from pure homologues should or should not be applied to
mixtures, particularly in cases where the pure homologue, which is not commercially supplied, shows higher or lower toxicity than the commercial mixture.
Lessons learned specific to the category:
• Health: None
• Environment: None
Case Study #10 (Health & Environment Review)
Category Name: Persulfates
Category members:
Ammonium persulfate
Potassium persulfate
Sodium persulfate
Brief description of category:
The category chemicals have similar chemical structure and physicochemical properties. These inorganic
substances differ only by the cationic portion of the salt. The anionic part is identical and based on the
available data; the potassium and sodium salts are expected to display similar environmental,
ecotoxicological and mammalian toxicological properties. Dissolution of the ammonium cation may
complicate the toxicological profile of ammonium persulfate.
QSARs were not used to support any toxicity and ecotoxicity data.
Category issues raised during comment/SIAM:
A minor comment was made concerning including acute toxicity values for each category member rather
than providing them as a range.
Ammonium persulfate is the "odd one out". Unlike the other two members which when dissociated in
solution exist as simple alkali metal cations and the persulfate anion, the ammonium cation will also be in
equilibrium with ammonia depending on the solution's pH. Dissolution of ammonium persulfate will also
affect pH itself (cf case study #6). This may complicate the toxicological profile of ammonium
persulfate.
Generic lessons learned:
• Include individual toxicity values for category members where feasible and possible.
Lessons learned specific to the category:
• Health: None
• Environment: Ammonium persulfate is the "odd one out". Unlike the other two members which when
dissociated in solution exist as simple alkali metal cations and the persulfate anion, the ammonium cation will also be in equilibrium with ammonia depending on the solution's pH. Dissolution of ammonium persulfate will also affect pH itself (cf case study #6). This may complicate the toxicological profile of ammonium persulfate.
Case Study #11 (Health & Environment Review)
Category Name: C9 Aromatic Hydrocarbon Solvents
SIAM: 21
Category members:
1,2,4-trimethylbenzene
1,3,5-trimethylbenzene
ethylmethylbenzene (mixed isomers)
solvent naphtha, (petroleum),
Brief description of category:
The C9 Aromatic Hydrocarbons Solvents Category is first composed of petroleum naphtha hydrocarbon
mixture, "Solvent naphtha, (petroleum), light aromatic" (CAS number 64742-95-6), which distils within a
narrow boiling range ( 160-175ºC). Light Aromatic Solvent Naphtha, hereafter referred to as C9
Aromatic Naphtha, is a mixture of individual isomers that predominantly have 9 carbon atoms with a
benzene ring and a limited number of short-chain alkyl constituents. Typical constituents may include:
three methyl groups (e.g., 1, 3,5-trimethylbenzene and 1,2,4-trimethylbenzene), an ethyl and a methyl
group, one propyl group (e.g., isopropyl benzene), and small percentages of C8 and C10 aromatic
hydrocarbons (e.g., mixed xylenes).
This category also includes three of the individual C9 aromatic isomers (1,2,4-trimethylbenzene, 1,3,5-
trimethylbenzene, and mixed ethylmethylbenzenes), that have relatively limited production and are used
primarily as chemical intermediates. The justification for including the isolated C9 aromatic isomers in
the category with the C9 aromatic naphtha is:
(1) Trimethylbenzene (TMB) and ethyltoluene (ET) isomers are major constituents in the C9 aromatic
naphtha. The C9 aromatic naphtha (CAS# 64742-95-6), which was the subject of a 1985 U.S. TSCA test rule, was required to have a minimum total ET-TMB content of 75%. Commercial C9 aromatic naphtha typically contains 1,2,4-trimethylbenzene at 20-45%, 1,3,5-trimethylbenzene at 8-15%, and mixed ethylmethylbenzenes at 25-35%.
(2) All category members have similar physicochemical and fate properties. (3) Existing data indicate that the toxicity of the isolated C9 aromatic isomer substances is similar to that
of the C9 aromatic naphtha.
Category issues raised during comment/SIAM:
Significant issues were related to category member inclusion and data completeness/adequacy.
Category Member Inclusion Issues:
1) It was suggested that the SIAR, based on "C9 aromatic hydrocarbon solvents" was too broad and that
the conclusions regarding human health should more closely match the available data rather than being
attributed to the entire category without a stronger basis.
2) It was suggested that the following three chemicals (that are on the OECD HPV list) to be added to the category (note: the sponsor did not add the chemicals):
611-14-3: 1,2-Ethylmethylbenzene 622-96-8: 1,4-Ethylmethylbenzene 25551-13-7: Trimethylbenzene (mixed isomers)
3) See Case Studies #4 and #5 for related category member inclusion issues.
Data Issues:
1) The physicochemical and fate properties were not available and were not similar for all category
members as had been stated in the SIAR. Therefore, the category discussion needs to be clearer as to
which data are available and the SIDS documents need to describe cases (e.g., biodegradation) where data
were not similar.
2) It was suggested that a ready biodegradability test according to the OECD TG 301 and GLP for the
substance 1,2,4-trimethylbenzene (TMB) OR 1,3,5-TMB be conducted to confirm the conclusion on the
ready biodegradability of all members of the category. If the new study showed no ready
biodegradability, the justification of the category would need to be revised and other biodegradability
tests may need to be performed. As a result, the assessment was not agreed upon and will be
presented at a future SIAM for agreement.
3) Data gaps for genotoxicity were noted. The C9 aromatic naphtha mixture could be about 33%
ethylmethylbenzenes and there should be some genotoxicity data on this class of compounds. If there are
data, they should be included since the SIAR is not specifically to C9 aromatic naphtha but more broadly,
for the C9 aromatic hydrocarbon solvents category. The sponsored mixture also contains significant % of
n-propylbenzene and cumene; key genotoxicity endpoints for these chemicals (or at least some discussion
ofthem) should be included in the SIAR.
Another commenter noted that the SIDS dossier only contains information on the mammalian toxicity and
genotoxicity studies on 1,2,4-TMB and 1,3,5-TMB that are cited in the SIAR (and no acute mammalian
toxicity data were included). Toxicity studies on these compounds were only generally used in the SIAR
if a particular toxicity end-point was not covered by a study on the mixture (C9 aromatic naphtha). The
SIDS dossier and the SIAR should contain a wider range of data for these compounds because they are
category members (and it appears that some acute and genotoxicity data are available for both
compounds). The US response was to suggest adding the genotoxicity data if they are adequate.
4) Additional ecotoxicity data should be gathered to support the statement that the toxicity of the isolated
C9 aromatic isomer substances is similar to that of the C9 aromatic naphtha (i.e., fish toxicity data were
available only for 1,2,4-TMB and C9 aromatic naphtha [CAS No. 64742-95-6] and daphnia and algae
toxicity data were available only for C9 aromatic naphtha). Because methylethylbenzenes are a
generalised structure of 3 isomers, it was also suggested that two of these isomers (i.e. 1,3-ethyltoluene
and 1,4-ethyltoluene; the two isomers present at highest concentration in solvent naphtha) be tested in
order to check that the position of the substitution on the benzene ring has no effect on the
ecotoxicological profile of the substance. Additional data were to be added for 1,2,4-trimethylbenzene
and 1,3,5-trimethylbenzene; these data are expected to cover the ethylmethylbenzenes (as suggested by
computer modelling).
5) For mixed ethymethylbenzene, the IUCLID contained data mainly on the substance 1-ethyl-3-
methylbenzene (CAS No. 620-14-4). A commenter noted that the other two components should have
been taken into account as well. The US Response was to add more data.
6) For mixed ethymethylbenzene, a commenter notes that no mammalian toxicity data were contained in
IUCLID – however, the commenter found data to add. The U.S. response was that we would consider
adding some information on the additional chemicals.
Generic lessons learned:
• It is important to provide a well-reasoned category justification/discussion.
• It is important to provide all of the data available for each chemical in a category. Generally, broad
categories with data primarily on mixtures will require more information and discussion to justify the category.
• It is not appropriate to use data for mixtures in a category to represent single substances in a category
(e.g., in the case of biodegradation).
Lessons learned specific to the category:
• Health:
• Environment:
Case Study # 12 (Environment Review Only)
Category Name: Hydrotropes
SIAM: 21
Category members:
Chemical name
Xylenesulfonic acid, sodium salt
Toluenesulfonic acid, sodium salt
Xylenesulfonic acid, ammonium salt
Cumenesulfonic acid, sodium salt
28348-53-0 and 32073-22-6
Cumenesulfonic acid, ammonium salt
Supporting Substances:
Chemical name
Xylenesulfonic acid, sodium salt
Xylenesulfonic acid, calcium salt
Xylenesulfonic acid, potassium salt
Toluenesulfonic acid, potassium salt
Brief description of category:
6 category members, 4 supporting substances. Category formed based on structural similarities, use
pattern, environmental fate, and (eco)toxicological properties. Category is comprised of 3 sub-groups
(methyl, ethyl and 2-propyl substituted benzenes) and a range of positional isomers (varying locant for
Me, Et, iPr group on the ring; ortho, meta or para). However the sulfonic acid has a greater effect on
physical-chemical properties than stepwise increase in substituent carbon chain length, so read-across
from one sub-group to another is deemed valid.
Category issues raised during comment/SIAM:
Generic lessons learned:
• It might be preferable to include all substances that are within the scope of the category (i.e. the
supporting substances). Industry's decision not to do so was because the excluded substances were not HPV.
Lessons learned specific to the category:
• Health: Not Reviewed
• Environment: None
Case Study #13 (Health Review Only)
Category Name: Diethylene Glycol Ethers
SIAM: 21
Category members:
Diethylene glycol ethyl ether (DGEE)
Diethylene glycol ethyl ether acetate (DGEEA)
Diethylene glycol propyl ether (DGPE)
Diethylene glycol butyl ether acetate (DGBEA)
Diethylene glycol n-hexyl ether (DGHE)
Brief description of category:
Using a category approach for screening level hazard assessment for the glycol ethers is appropriate based
on similarities in molecular structure and functionality (with a generic molecular structure of HO(CH2
CH2O)2 R or CH3C(=O)O(CH2 CH2O)2 R), where R = a straight chain alkyl group (ethyl, propyl, butyl, or
hexyl). The diethylene glycol ethers contain a free hydroxyl group at the end of the molecule. The
diethylene glycol ether acetates differ from the ethers in that the hydroxyl group is esterified with an
acetic acid group.
The category members and supporting chemicals demonstrate similar physical and environmental fate
properties. The diethylene glycol ethers in the category exhibit a trend in aquatic toxicity (which is also
supported using analogues). As the molecular weight and log Kow increase, the toxicity also increases.
The same trend can be observed for the acetates. Each group (ethers and acetates) must be treated as
subcategories because of the marked differences in functionality and the fact that the acetates only slowly
hydrolyse to the alcohol (ether) under environmentally relevant aqueous conditions.
In mammalian systems, the diethylene glycol ethers have similar metabolic pathways – they are poor
substrates for alcohol dehydrogenase (Dow Chemical Company, 1982) and good substrates for
cytochrome P-450 (Kawamoto et al., 1990). Further, a major metabolite of DGEE, (68% in urine) was
(2-ethoxyethoxy)-acetic acid (Miller, 1987); a major metabolite of DGBEA was identified as 2-(2-
butoxyethoxy)-acetic acid (Deisinger and Guest, 1985a). Also, DGEEA and DGBEA are included
because evidence demonstrates that DGBEA is rapidly hydrolyzed to DGBE by esterases present in
blood.
The mammalian toxicities show a pattern consistent with the molecular and metabolic similarities of the chemicals. Valid repeated dose oral toxicity studies conducted with DGEE, DGPE, DGBEA, DGHE and the supporting chemical DGBE (ranging in duration from 30 days to 2 years) generally report kidney and liver toxicity, absolute and/or relative changes in organ weights, and/or some changes in hematological parameters at high doses. The majority of studies show no reproductive or developmental toxicity for the diethylene glycol ethers in this category.
Category issues raised during comment/SIAM:
The category approach was clear and well-documented, with good choices for the supporting chemicals.
The similarities show that the chemicals could be appropriately grouped together (although additional
discussion of trends was requests; and added). The conclusions for the category outlined in the SIAR and
SIAP appear to be sound.
Only selected members of ‘diethylene glycol ethers' were included. Absence of diethylene glycol
monomethyl ether was noted. Diethylene glycol butyl ether was used only a supporting chemical because
it was presented at an earlier SIAM, whereas DGBEA (the acetate) was included in the current category.
Addition of a table listing the reliable studies available for the category members for all health end points
would be useful.
Generic lessons learned:
• Future submissions should be as complete as possible and should aim to include the most relevant
chemicals for a cohesive category. Incentives for industry sponsors to include all chemicals that are covered by the definition of a category would help achieve this goal.
• Data matrices included in submissions would improve clarity and facilitate easier review.
Lessons learned specific to the category:
• Overall, this category submission [Category - Diethylene Glycol Ethers] was considered to be very
good; the category justification and supporting chemicals were well supported, data was complete, and conclusions in the SIAR and SIAP were deemed sound.
• When structurally related ethers and ether acetates are proposed as a single category, data (trends)
should be assessed to determine if splitting into ether and acetate sub-categories is more appropriate for relevant endpoints/sections.
• Health: • Environment:
Case Study #14 (Health & Environment Review)
Category Name: Amine Oxides
SIAM: 22
Category members:
1-Dodecanamine, N,N-dimethyl-, N-oxide
1-Tetradecanamine, N,N-dimethyl-, N-oxide
Amines, C10-16-alkyldimethyl, N-oxides
Amines, C12-18-alkyldimethyl, N-oxides
Supporting members (later added to the category):
Chemical name
Decanamine, N,N-dimethyl-, N-oxide
Hexadecanamine, N,N-dimethyl-, N-oxide
Octadecanamine, N,N-dimethyl-, N-oxide
Amine oxides, cocoalkyldimethyl
Amines, C10-18-alkyldimethyl, N-oxides
Amines, C12-16-alkyldimethyl, N-oxides
Ethanol, 2,2'-iminobis-, N-coco alkyl derivs.,
Ethanol, 2,2'-(dodecyloxidoimino)bis-
Ethanol, 2,2'-(octadecyloxidoimino)bis-
Ethanol, 2,2'-iminobis-, N-tallow alkyl derivs.,
Ethanol, 2,2'-[(9Z)-9-octadecenyloxidoimino]bis- 93962-62-0
Brief description of category:
The justification for grouping the amine oxides into a category is based on their structural and functional
similarity. All of the substances in this category are surfactants, and have a polar "head" (the amine
oxide) and a relatively inert hydrophobic "tail" (the long alkyl substituent). The structural variations in
the category are three-fold: 1) the nature of the second and third substituents on the amine are either
methyl groups or hydroxyethyl groups; 2) the long alkyl chain ranges in length from 8 to 20 carbons; and
3) the long alkyl chain may contain one or two double bonds as in C18:1 (oleyl) or C18:2 (linoleyl).
Commercial amine oxides are either alkyl dimethyl or alkyl dihydroxyethyl amine oxides. These two
categories contain 2 methyl groups or 2 hydroxyethyl groups, respectively, which are attached to the
tertiary nitrogen. Alkyl chain lengths range from 8 to 20. The predominant lengths for these chains are
12 and 14, with average chain lengths for the mixtures of 12.9 to 13.5; one exception regarding the chain
length is for the tallow-derived compound. All CAS numbers represent complex mixtures of various
chain lengths, with the predominant chain length cited as the chemical name for the category members.
The nomenclature and CAS identifications for these amine oxides are based on historical and
geographical considerations, not on significant differences in the structure and composition of the
commercial substances.
Typical structures for amine oxides are as follows:
C12 dimethyl amine oxide
C12 dihydroxyethyl amine oxide
The chemical behaviors of the amine oxides are expected to be very similar. Differences relate to the
alkyl substituents (i.e., their nature [methyl, hydroxyethyl] and the number of carbon atoms in the alkyl
chain [8 to 20]). The presence of methyl- vs. hydroxyethyl-substituents affects the basicity of the
nitrogen only marginally, and the hydroxyethyl group lends more bulk to the hydrophilic head-group of
the surfactant. The length of the longest alkyl substituent does not alter the chemical reactivity of the
molecule, but does affect its physical properties.
Category issues raised during comment/SIAM:
At SIAM it was decided to include the 11 supporting substances in the category, increasing the CAS
number count from 4 to 15. There had been some confusion amongst member countries previously about
which chemical members constituted the category.
Several potential differences among category members were noted. Characteristics of ethyl and
hydroxyethyl members may vary more than just in bulk of head group; hydrophilicity was one possible
difference noted. It was also suggested that unsaturated analogues among the hydroxethyl members may
react differently. Lack of data to demonstrate that hydroxyethyl compounds have similar behaviour
across a range of endpoints was noted.
Due to greater dissimilarity between ethanolamines and the four main amine oxide substances as
compared to the other six supporting amine oxides (which are dimethyl amine oxides), it was suggested to
split the category into two subcategories (if the supporting substances are to be category members), or
minimize read across from the ethanolamines to the main substances (if they are intended to be
supporting only). Ecotoxicity and biodegradation data for ethanolamines are quite similar to the dimethyl
amine oxides.
For substances that are a complex mixture of various chain lengths, values for certain physicochemical
properties should be presented for each carbon chain length present in the complex mixture (substance).
Although this has been partially addressed using EPIWIN, some endpoints have been filled by computer
modelling but others have not. The values were presented only for those endpoints for which it is
considered acceptable to use such modelled data.
The commenter at SIAM suggested that consideration should been given as to how biodegradation rate
would vary between components with longer and shorter chain alkyl groups. The US responded that
longer chain-lengths are more sorptive and that ready biodegradation seems to apply up to a C17.2 AO,
suggesting that biodegradation in soil would apply for the longer chain-lengths (but no data exist to
support conclusions regarding variability in biodegradation rates).
In the discussion of chronic toxicity to fish, daphnia, algae, a "normalized" (mean) value for carbon chain
length (C12.9) was used. It was suggested that values for each of the category members in the dossier
and in the SIAR be added, or at least a range provided, to better represent the actual toxicities observed
for individual category members.
It appeared that all category members (i.e. CAS numbers) displayed acute ecotoxicity according to GHS
Acute I classification to at least one aquatic organism. This is due to the complex nature of each member,
having a range of carbon chain lengths, but all containing a proportion of the more toxic chain length
components. For this reason a sub-group approach was not used for the environment, and all members
were considered together.
Generic lessons learned:
• Although there is guidance that suggests that values be provided for each category member when
presenting the documents at SIAM, the guidance has not been practiced in most cases.
• Information on individual compounds with different carbon chain lengths can be useful supplemental
information (measured/modelled) when category members are mixtures.
• Data gaps may be appropriately filled using modelled data in some cases. However, because certain
endpoints can be modelled for certain chemicals, does not imply that all data gaps can be filled using a single model (i.e., models are not "one-size-fits-all", rather, they need be applied within the context for which they were designed).
Lessons learned specific to the category:
• Health:
• Environment:
o Each category member is a complex mixture of chain lengths. As certain chain lengths are more
toxic than others and these chain lengths appeared to be present in each category member, all members were considered together for the environmental assessment.
o Each category member is a complex mixture of chain lengths. A conclusion for biodegradation
based on tests on mixtures is not strictly possible to derive according to current OECD guidance.
However it may be possible to infer from the results that most of the chain lengths are likely to be
readily biodegradable. This issue is likely to come up time and time again for commercial
products under REACH.
Case Study #15 (Health & Environment Review)
Category Name: Epoxidized Oils and Derivatives (EOD)
SIAM: 22
Category members:
Fatty acids, tall-oil, epoxidized, 2-ethylhexyl esters (ETP)
9-Octadecanoic acid (Z)-,epoxidized,
ester w/propylene glycol (EODA) Epoxidized soybean oil (ESBO)
Epoxidized linseed oil (ELSO or ELO
Brief description of category:
The category chemicals are epoxidized esters of naturally occurring; long chain fatty acids, primarily the
C18 acids (oleic, linoleic, and linolenic acids) with primary alcohols, diols, or triols. There is a
considerable overlap in the composition of the fatty acid portions of these products. These materials are
considered a category for purposes of environmental and health hazard screening assessments because of
their similarities in metabolism in microbial, aquatic, and mammalian systems. It is well understood that
fats in this category will be metabolized by carboxylesterases of fish, aquatic invertebrates and mammals
resulting in a mixture of epoxidized fatty acids and 2-ethylhexanol from ETP, epoxidized fatty acids and
propylene glycol from EODA, and epoxidized fatty acids and glycerol from ESBO and ELSO. Being the
primary constituents of the metabolic process, the epoxidized fatty oils are considered as representative
chemicals to assess potential hazards. Alcohols, the minor constituents of the metabolic products are not
produced in sufficient quantities to influence the toxicity profile of the EOD materials. In addition, the
hazard profiles of these alcohols have been assessed in previous SIAMS.
QSARs were not used to support any toxicity and ecotoxicity data.
Basically, all EODs are used in plasticizers to keep plastics and rubber soft and pliable. ESBO and ELSO
are approved by US EPA for use as inert ingredients in pesticides.
Read-across is important in the category:
Read Across from
Read Across to
Endpoint
EODA, ESBO, ELSO
Biodeg., genetic tox., repro tox, developmental tox
acute fish, daphnia and algae
Chronic Daphnia Tox*
*Test abandoned due to unmeasurable concentrations of test material. Same situation inferred for the other, less soluble cat.members.
For the environment, only non-standard acute tests with fish and daphnia were available for one category
member, ESBO. A chronic test was started with the most water soluble category member, EODA, but no
test substance was measured above the 0.02 mg/l LoD using the water accommodated fraction (WAF)
approach. Therefore the test was discontinued. ESBO also had valid algal inhibition data, again read-
across to the other category members. Given the high log Kow values for all 4 category members and
comparably low water solubility in addition to similar structural features, the read-across was stated to be
robust.
Category issues raised during comment/SIAM:
Lack of metabolism data on these chemicals made category justification questionable. However, the
common knowledge of fat metabolism by lipases and esterases, low toxicity shown in the repeated-dose
toxicity studies, and available potential hazard information on minor metabolites strengthens the
justification for grouping these chemicals into the category.
Originally the only algal study (ESBO) was deemed invalid, and given the lack of valid guideline ecotox
studies it was recommended that another algal study be conducted with one category member. The algal
study was re-evaluated and found to be valid; read-across was then deemed valid.
In addition it was recommended that a further attempt be made towards a chronic daphnia study.
Justification for discontinuing the original test was provided and deemed satisfactory.
Differences in available biodegradation tests with one category member indicated a discrepancy in ready
vs. non-ready biodegradation status. Justification given and biodegradation status confirmed and
subsequently read-across applied.
Generic lessons learned:
• When grouping chemicals together based on common metabolic pathways, include appropriate data or
discussion on how these chemicals are related, structurally, functionally, or metabolically.
• Good example of an organic category in which members have similar functional groups and overall
structural arrangement and physico-chemical properties, but differences in size/chain length and numbers of functional groups/side chains which seem not to affect its validity. As mentioned read-across is heavily relied upon for the category; similarities in Log Kow, water solubility and structure help to justify this.
Lessons learned specific to the category:
• Health: Provide strong and appropriate justification when metabolism data are not available.
• Environment:
• The read-across used is simplified for ecotoxicity in that it is essentially qualitative rather than
numeric; i.e. effects are defined as only to be seen above water solubility limit. The "continuum" category approach does not apply.
• The use of QSARs could have further justified the ecotoxicity read-across within the category, by
the comparison of QSAR data between members that were read-across.
Case Study #16 (Environment Review Only)
Category Name: Methanolates
SIAM: 22
Category members:
Sodium Methanolate (NaOMe)
Potassium Methanolate (KOMe)
Brief description of category:
Two members have similar physico-chemical properties, rapid reaction in water to give similar
degradation products (methanol and sodium/potassium hydroxide), and use patterns. Both NaOH and
KOH agreed low hazard for the environment. The primary hazard issue is with methanol toxicity.
Category issues raised during comment/SIAM:
A minor issue was raised regarding the treatment of methanol, the degradation product from both
category members. As methanol is a concern for human health (as per its SIDS assessment), this needed
to be mentioned, although it did not influence the conclusion for this category.
Generic lessons learned:
• For categories that cover reactive chemicals, the reaction/degradation products must be of a similar
nature for each member of the category to be credible.
Lessons learned specific to the category:
In this category the compounds react rapidly with water to form similar compounds and methanol.
Methanol is the only degradation product of concern (human health), but did not affect the conclusion for
the category. Methanol has its own SIDS assessment.
• Health: Not Reviewed.
• Environment: None
Case Study #17 (Health & Environment Review)
Category Name: Primary Amyl Acetate
SIAM: 22
Category (chemical) members:
Primary Amyl Acetate (Mixed Isomers), mixture composed of:
Chemical name
65% 1-pentyl acetate
35% 2-methyl-1-butyl acetate
Brief description of category (not true category):
Not a category but a mixture composed of two isomers as a result of the reaction process, and supplied as
such. Physico-chemical properties, where available, for the two isomers are presented in addition to
values for the mixture. Read-across from 1-propyl acetate (CAS No. 109-60-4) and 1-butyl acetate (CAS
No.123-86-4) is used to support the acute fish toxicity data generated using Primary Amyl Acetate.
The SIDS document presents data for the mixture, which is a reaction process-derived commercial
mixture of two isomers. Additional data for the individual components are presented in support of data
for the mixture. The individual components were not sponsored and are not HPV chemicals in the U.S.
Category issues raised during comment/SIAM:
The mixture has no single CAS number.
The assessment was of the composite mixture only, with data from the isomers used as support for the
mixture.
The individual isomers are not HPVs in the U.S. Inclusion of the individual isomers as "supporting"
information to the mixture does not qualify the individual isomers as having completed the SIDS/SIAM
process.
Generic lessons learned:
• In some cases, individual chemicals are HPV only as part of a mixture. Assessment of the mixture
does not quality the individual isomers as having gone through the SIDS/SIAM process, if they have been used only for "supporting" or analogue information. If the individual components of the mixture become HPV apart from the mixture, they will need to be sponsored separately.
• Under REACH a challenge will be to convince industry to include all relevant members based on
the basic properties excluding use pattern/exposure (and come to different conclusions for different members depending on hazard and use).
Lessons learned specific to the category:
• Health: None
• Environment: None
Case Study #18 (Health & Environment Review)
Category Name: Primary Amyl Alcohol
SIAM: 22
Category (chemical) members:
Primary Amyl Alcohols (Mixed Isomers), mixture composed of:
Chemical name
65% 1-pentyl alcohol
35% 2-methyl-1-butyl alcohol
Brief description of category (not true category):
Not a category but a mixture composed of two isomers as a result of the reaction process, and supplied as
such. Physico-chemical properties, where available, for the two isomers are presented in addition to
values for the mixture.
The SIDS document presents data for the mixture, which are reaction process-derived commercial
mixtures of two isomers in each case. Additional data for the individual components are presented in
support of data for the mixture. The individual components were not sponsored and are not HPV
chemicals in the U.S.
Category issues raised during comment/SIAM:
The mixture has no single CAS number.
The assessment was of the composite mixture only, with data from the isomers used as support for the
mixture.
The individual isomers are not HPVs in the U.S. Inclusion of the individual isomers as "supporting"
information to the mixture does not qualify the individual isomers as having completed the SIDS/SIAM
process.
Generic lessons learned:
• In some cases, individual chemicals are HPV only as part of a mixture. Assessment of the
mixture does not quality the individual isomers as having gone through the SIDS/SIAM process, if they have been used only for "supporting" or analogue information. If the individual components of the mixture become HPV apart from the mixture, they will need to be sponsored separately.
• Under REACH a challenge will be to convince industry to include all relevant members based
on the basic properties excluding use pattern/exposure (and come to different conclusions for different members depending on hazard and use).
Lessons learned specific to the category:
• Health: None
• Environment: None
Case Study # 19 (Environment Review Only)
Category Name: Bicarbonate Special
SIAM: 22
Category members:
Sodium Bicarbonate
Sodium Carbonate
Ammonium Bicarbonate
Brief description of chemicals (not a true category):
Not a true category in that commercial material is a reaction mixture of the 3 components [NaHCO3
(81%), Na2CO3 (13%), NH4HCO3 (3%)]. Separate SIDS dossiers are referred to for each component
(NaHCO3 and Na2CO3 in SIAM 15; NH4HCO3 separately in SIAM 22) hence the category approach is
applied.
Similarities:
• all 3 are stable solids except NH4HCO3 which decomposes with heat
• all 3 components dissociate to Na+/NH +
4 and HCO2-/CO3 in aqueous solution
• bicarbonate and carbonate ion can be considered as interchangeable in aqueous solution with the
relative concentrations of each being pH dependent, regardless of the form released
• all 3 have similarly low toxicological profiles; exceptions are NH3 corrosivity and sodium carbonate is
harmful by inhalation
3/NH4 cation cause concern for the environment
Category issues raised during comment/SIAM:
The use of a category approach was questioned because all of the proposed category members have been
assessed separately. The category approach was upheld as it was felt it was the best approach to this
mixture (the result of a complex reaction and not a formulation).
Generic lessons learned:
• For categories including ionisable compounds, water solubility and dissociation behaviour in aqueous
solution should be considered. These characteristics should be similar or follow a predictable trend among category members. In this case, the (effectively) complete dissociation of all 3 components reinforces the category approach.
• For categories including ionisable compounds, pH effect on behaviour in aqueous solution must be
Lessons learned specific to the category:
• Health: Not Reviewed • Environment:
o Read-across not important in this case - NH3/NH4 cation assessed separately to other
components. HCO2-/CO 2-
3 essentially the same in solution (depend on pH).
o In this case, the (effectively) complete dissociation of all 3 components reinforces the category
o In this case the bicarbonate and carbonate anions are essentially the same (concentrations
governed by solution pH), which strengthens the category approach.
Case Study #20 (Environment Review Only)
Category Name: Oxo Alcohols C9 to C13
SIAM: 22
Category members:
Chemical name
Alcohols C8-C10-iso, C9 rich
Isononyl alcohol
Alcohols C9-C11-iso, C10 rich
Isodecyl alcohol
2-Propylheptan-1-ol
Alcohols C11-C14-iso, C13 rich
Isotridecan-1-ol
Brief description of category:
Straight chain alkyl alcohols with one methyl or ethyl side chain except one member (propyl side chain);
total number of carbon atoms (inc. side groups) C9 – 13; a small "continuum" category. All, except 2-
propylheptan-1-ol, are manufactured in the same way and the category is justified by 4 factors: similarity
in chemical structure, physical-chemical properties, environmental fate and toxicological mode of action.
Category issues raised during comment/SIAM:
The category member with the propyl side chain was highlighted as a possible outlier to the category.
However, the 4 factors considered in the category justification all support its inclusion.
Generic lessons learned:
• For this "continuum" category the approach taken was to divide the assessment discussion into a
separate paragraph describing specific endpoints for each member for the (eco)toxicological sections. Summaries including graphical illustrations of the trends in data (e.g. linearly increasing aquatic toxicity with chain length) are very useful. This approach worked well as the category is both small (7 substances) and for the most part data rich.
Lessons learned specific to the category:
• Health: Not Reviewed
• Environment: The formation of sub-groups within a "continuum" category can be elaborated with
consideration of the relevant endpoint(s). In the HPV programme 2 category members (C13) exhibited higher ecotoxicity than the other members and so formed a sub-group for the conclusions in the assessment.
Summary of Discussions on the Use of QSARs, Read-Across and Grouping
in the Technical Committee on New and Existing Substances (TC NES)
Ivanka Tsakovska, Evelin Fabjan & Andrew Worth
European Chemicals Bureau
January 2007
SUMMARY OF DISCUSSIONS ON THE USE OF QSARs, READ-ACROSS AND GROUPING IN THE TECHNICAL COMMITTEE ON NEW AND EXISTING
SUBSTANCES (TC NES)
Results obtained by screening ECB electronic archives. Many of the source documents are available on the ECB website (ESIS)
Name / CAS
Approach
Source3 / Discussion
Edetic acid (EDTA)
Final RAR, 2004
Mutagenicity: Only a few data from genotoxicity assays were available, so data from structurally related EDTA
sodium salts (in vitro, Na3EDTA and in vivo, Na2EDTA) were used to conclude that EDTA is not mutagenic to humans.
logKow: The value used in the risk assessment is calculated according to Hansch and Leo.
Final RAR, 2004
Mutagenicity: The in vivo mutagenicity of aniline is supported by mutagenictiy data on the structurally related
substances 4-aminophenol and azobenzene. Conclusion: Aniline is classified as a category 3 mutagen.
Draft RAR, 2002
Aquatic compartment: QSAR estimations were used for comparative purposes. General agreement between
QSAR estimations and experimental values was found for toxicity to fish, to aquatic invertebrates, and aquatic
plants.
Trichloroethylene
Final RAR, 2004
Aquatic compartment: ECOSAR was used to estimate values for ecotoxicity endpoints for various species (algae,
daphnid, mysid, fish, earthworm). The predicted short-term toxicity values suggested a similar sensitivity across the
three types of organisms (fish, invertebrates, and algae).
Final RAR 2002
Mutagenicity: Mutagenicity data for structurally-related acrylic compounds (methyl methacrylate, ethyl acrylate,
methyl acrylate, butyl acrylate) were used as supporting information to conclude that acrylic acid is unlikely to be
mutagenic in vivo.
Methacrylic acid
Final RAR, 2002
Mutagenicity: Methacrylic acid is negative in a bacterial gene mutation test. Further test data on methacrylic acid
was lacking. However, taking into consideration the data on the structurally related substance methyl methacrylate -
which indicate that this substance does not express a genotoxic potential in vivo – it was concluded that there is no
need for further testing.
Developmental toxicity: There were no data on reproductive toxicity of methacrylic acid. On the basis of data for
the methyl ester of methacrylic acid (methyl methacrylate), it was concluded that there is no concern in relation to
reproductive toxicity.
Dibuthyl phthalate
Final RAR, Addendum to the environmental section, 2004
Adsorption: Koc calculated by the TGD QSAR was used.
Final RAR, 2003
Adsorption: Koc calculated by the TGD QSAR was consistent with the experimental values and was used in the
assessment.
Aquatic compartment: Acute toxicity values were predicted by using QSARs for chemicals that act by narcosis.
The predicted values were found to fit closely the range of measured values, providing evidence that naphthalene
exerts its toxic effect by narcosis.
Final RAR, 2001
Aquatic compartment: The QSAR estimated chronic fish toxicity (NOEC) was used in the assessment. The
validity of the QSAR was checked by comparing the QSAR estimate of chronic toxicity for Daphnia magna with
the measured chronic toxicity.
Final RAR, 2002
Aquatic toxicity: Styrene is considered to exert toxic effects by a non-specific mode of action. The effect
concentrations were calculated by using respective QSAR equations for non-polar narcosis included in the TGD.
The short-term toxicity predictions for fish, Daphnia and algae were found to be in good agreement with the valid
measured values available.
Koc: Estimated from hydrophobics equation from Section 4.3 of Chapter on QSAR in the TGD.
Final RAR, 2002
Aquatic toxicity: No aquatic toxicity test data were available due to the physical nature of the substance (volatile,
carcinogenic and flammable). Thus the toxicity was estimated by using QSAR and read across from structurally
similar substances. In the QSAR estimations, the equations for non-polar narcotic chemicals were used. The
following endpoints were calculated: 72h EC50 for algae, 48h EC50 for Daphnia magna, 96h LC50 for fathead
minnow, 16 day NOEC for Daphnia magna, 28-day NOEC for zebra fish and fathead
minnow.
In the read across approach, experimental data for isoprene (2-methyl-1,3-butadiene) and 1,3-pentadiene were used.
The read across data suggested that 1,3-butadiene may be slightly less toxic than might be expected from QSAR
predictions. Therefore the PNEC was based on both acute L(E)C50 QSAR predictions with an assessment factor of
1,000 and the long-term NOEC QSAR predictions with an assessment factor of 100. The PNECwater derived from
the acute L(E)C50 was lower than the PNECwater derived from the long-term NOEC QSAR and was therefore
proposed as a conservative approach to be used in the assessment.
Biodegradation: The results of two different QSAR models (BIODEG and OECD-model-75) were used to support
the test data and point to the ready biodegradability of the substance.
Vinyl acetate (VAM)
Draft RAR, 2006 (R059_0605_env.doc)
Accumulation: Bioaccumulation test data were not available. However, due to the high vapour pressure, ready
degradability and low lipophilicity of the substance, it was argued that VAM is neither expected to bioconcentrate in terrestrial or aquatic organisms, nor to biomagnify in food chains. This appraisal was supported by results from QSAR calculations (BCFWIN v.2.14).
Final RAR, 2006
Aquatic toxicity: The QSAR estimates (according to the TGD models) for fish (96h) LC50 and Daphnia (48h) EC50
for polar narcotics were found to be in reasonable agreement with the experimental values.
Final RAR, 2004
Aquatic toxicity: Using the TGD QSARs for the base-line toxicity, estimates for fish, Daphnia magna and algae
were derived. These estimates were consistent with the measured concentrations, confirming that cyclohexane acts
by a non-specific mechanism.
Ethyl acetoacetate
Final RAR, 2002
Hydrolysis: Experimental data were not available for ethyl acetoacetate hydrolysis Half-lives for hydrolysis
amounting to between approx. 15 days (pH = 8) and 149 days (pH = 7) were calculated with QSARs. This
permitted the conclusion that hydrolysis is not a significant elimination process for ethyl acetoacetate in the
environment.
Aquatic toxicity: QSAR equations for non-polar narcotics are used to predict the toxicity for different species. The
QSAR estimations support the experimental data in the conclusion that fish is slightly more sensitive to ethyl
acetoacetate than daphnia or algae.
4-chloro-o-cresol
Final RAR, 2002
Adsorption: Various QSAR estimations give a Koc range of 14 to 700. Due to the lack of an experimental Koc
value, the calculated value of Koc = 400 l/kg was used in the risk assessment.1
Aquatic toxicity: TGD QSARs for acute fish and daphnid effects compared well with experimental data,
suggesting a polar narcotic mechanism of toxic action. This was supported by an evaluation of the acute mode of toxic action towards fathead minnow of the structurally related substance 4-chloro-3-methylphenol.
TM I,'03, TC NES IV'04 (mi_071_tc0404_hh_dr1.doc)
Repeated dose toxicity: NL noticed shortcomings in the oral and inhalation data for this end-point. UK suggested
read-across from ethanol or butanol. However, the use of read-across approach from other low molecular weight
alcohols was not accepted.
Final RAR, 2002
Adsorption: No measured values for Koc were available, so the TGD QSAR equation was used for its calculation.
Draft RAR, 2006 (R035_0602_env.doc)
Aquatic toxicity: The use of ECOSAR assigns CBS to the group of neutral organics whereas the estimation
sulphen-amide (CBS)
approach of Verhaar et al (1992) considers CBS to belong to the class 3 (reactive chemicals).
The acute toxicity tests indicated the algae is the most sensitive species followed by fish and then daphnids. QSAR
predictions also indicate that algae is the most sensitive species. The ECOSAR estimates for algae and fish are
slightly lower than the test results, but still above the water solubility. For daphnids, ECOSAR gives a significantly
lower estimation than the test available. The ECOSAR predictions were included as supporting information in
the risk assessment.
Breakdown products: Using the QSARs in EPI Suite v3.12, estimates of the following physicochemical properties
were obtained for benzothiazolsulphonic acid (BTSO3H): water solubility, logKow, volatility. According to the
ECOSAR v0.99 it is not toxic to aquatic organisms (acute effect values 473 g/l). As a conclusion, BTSO3H is not
expected to elicit any effects in the concentration range detected and expected in the aquatic environment.
1 These units are given in the RAR, but this is thought to be a mistake, because this is a coefficient and should therefore be without units
Draft RAR, 2004
Physicochemical properties: Kow was calculated with a QSAR equation (Leo and Hansch, 1995).
Final RAR, 2003
Aquatic toxicity: The ECOSAR program was used to predict the following aquatic endpoints: fish (96h LC50,
Daphnid (48h) LC50, Daphnid (16 day) EC50 and algae green (96h) EC50. The QSAR data were found to be in accordance with the measured data and therefore provided supporting information.
ethyl)phosphate (TCEP)
TM IV '03 discussion (mi_068_tc0403_hh_dr1.doc): IRL noticed that it had sensitisation test results available on
analogous compounds indicating that these substances were not skin sensitizers.
TC NES II '04 discussion (mi_068_tc0403_hh_dr1.doc): UK asked the rapporteur to give more justification in the
report on the adequacy of the read across from the structurally-related phosphates used (TCPP, V6 and TDCP). UK
was of the opinion that TCEP was more related to TCPP than to the other two chlorophosphates. D noted that the
main structure was similar but differences in chain length may influence the sensitization properties. IRL
considered read across from TCPP and TDCP to be more appropriate than from V6.
Draft RAR, 2006: Human data on sensitizing properties of TCEP were not available. An animal skin sensitisation
study showed no skin sensitizing potential. No further testing was recommended based on read across from
sensitisation data of the two structurally related chloroalkyl phosphate esters TCPP and TDCP (substances of the 4th
Priority list). These analogues were considered not to possess significant skin sensitisation potential, based on
guinea pig and local lymph node assay data.
1,2,4-trichlorobenzene
Final RAR, 2003
Aquatic toxicity: The QSAR estimates for acute fish toxicity, short-term toxicity to crustaceans and toxicity to
algae were found to fit well with the experimental data.
Endocrine disrupting activity: According to a HQSAR for estrogen receptor binding activty (Mekenyan, 1998),
1,2,4-TCB was not expected to bind to the estrogenic receptor and therefore not to show estrogenic activity. Test
studies confirmed that 1,2,4-TCB does not have any specific estrogenic activity.
Systemic toxicity: Large parts of the hazard section are identical in the risk assessment reports for the six zinc
compounds. The grouping approach is based on the assumption that the zinc cation (for dissolved zinc species) is
the determining factor for systemic toxicity.
1314-13-2, Zinc chloride 7646-85-7 Zinc sulphate, 7733-02-0 Trizinc bis(ortho-phospate) 7779-90-0
Final RAR, 2002
Toxicokinetics: Read across data from actylphenol (an alkyl phenol with a close structural relationship to
nonylphenol) was used to support the limited toxicokinetic data for nonylphenol obtained from rat and human
studies.
2,4,4-trime-thylpentene
Draft RAR, 2005
Toxicokinetics, metabolism and distribution: No data were available on 2,4,4-trimethylpentene. Therefore, the
results from studies on structurally similar 2,3,4- trimethylpentane and short chain olefins (n-1-octene, n-4-octene,
3-ethyl-2-pentene) were used in the risk assessment.
Di-' isodecyl' phthalate
Final RAR, 2003
Henry's law constant: Calculated by a QSAR described in the TGD.
Chronic fish toxicity: No data were available on chronic effects on fish. Data for closely related phthalates
(including C6 to C11 dialkyl phthalate esters) were read across to DIDP, indicating no chronic toxicity.
Skin sensitisation: Based on human data there is some evidence that DIDP may cause sensitization. Read across
from other phthalates, particularly DEHP and DBP, provided supplementary information for a low sensitizing
effect (if any). The weight of evidence was deemed insufficient to justify a classification.
Carcinogenicity: No carcinogenicity data on DIDP were available. On the basis of read across from other
phthalates (specifically DINP), it was concluded that there is no concern for carcinogenicity.
Di-' isononyl' phthalate
Chronic fish toxicity: On the basis of one study available and the large body of existing literature for closely
related phthalates, it was concluded that DINP has no adverse effects on fish.
Final RAR, 2001
pentabromo derivative
Toxicokinetics: There were no data on inhalation or dermal absorption. However, comparison with structurally
similar substances, such as penta and hexa brominated biphenyls (PBBs) and penta and hexa chlorinated biphenyls
(PCBs), suggested that the substance may be well absorbed by all routes of exposure. On the basis of all findings related to toxicokinetics, it was concluded that information is required concerning this substance with respect to breast milk, including uptake from breast milk to the infant.
Aquatic toxicity: Aquatic toxicity predictions obtained using the equations in TGD (for fish, Daphnia magna and
algae) were generally in good agreement with the experimental results.
1-vinyl-2-pyrrolidone
Final RAR, 2003
Toxicokinetics: Since it was not possible to estimate percentage bioavailability by the dermal route, comparison
with the structurally similar compound N-methyl-2-pyrrolidone, were used as in addition to the physicochemical characteristics to predict good dermal absorption.
Tert-butyl hydroperoxide
Draft RAR, 2006 (R319_0604_hh.doc)
Bioaccumulation: The BCF for fish was estimated with the QSAR in EUSES.
Monochloroacetic acid
Final RAR, 2005
Photodegradation: The photo-oxidation rate of MCAA with OH-radicals was estimated with a QSAR (Atkinson,
TGD 1996) and this value was used in the risk assessment.
Soil-water distribution: Koc was calculated using a QSAR for organic acids (the RAR does not indicate which
QSAR).
4,4'-isopropylidene
Final RAR, 2003
Bioaccumulation: An earthworm BCF of 7.9 kg/kg was estimated by using a QSAR in EUSES. Measured data for
fish BCF were used in preference to QSAR estimates.
Physicochemical properties: Water solubility was calculated with a QSAR based on logKow, melting point and
molecular weight. The measured and calculated water solubility values were in the same range. However, the
dinitroacetophenone
measured value was used in preference.
Bioaccumulation: No experimental data on accumulation in earthworms were available. The BCF was estimated
according to the TGD QSAR.
Aquatic compartment: The toxicity using QSAR for non-polar narcosis in TGD was calculated for 96 hour LC50
for Pimephales promelas; 28-32 day NOEC (ELS) for Brachydanio rerio/Pimephales promelas; 48 hour EC50 and
16 day NOEC for Daphnia magna, 72-96 hour EC50 for Selenastrum capricornutu). The QSAR estimates were in
reasonable agreement with the experimental result, indicating that musk ketone acts by non-polar narcosis.
Final RAR 2005
Carcinogenicity: No data on carcinogenicity were avaiable for musk ketone. Data on carcinogenicity was available
for a structurally related compound musk xylene. This was read across to musk ketone and used for risk
characterisation.
QSARs were used in a similar way as for musk ketone.
trinitro-m-xylene (musk xylene) 81-15-2 Tert-butyl methyl ether
Final RAR, 2002
Aquatic toxicity: QSAR calculations according to the TGD were performed for acute toxicity to fish, Daphnia and
algae. They are of the same magnitude as measured values for fish and Daphnia and higher than the measured for algae. ECOSAR calculations were also performed and they were in good agreement with the experimental values for fish, Daphnia and algae. It is not clear from the RAR whether these QSAR estimates were used in the riask assessment.
Developmental toxicity:
TM III'03, In depth discussion (mi_311+312+419+420+424_tm303_hh_dr2.doc):
Industry did not agree with the rapporteur's recommendation that metallic nickel should be classified as reprotoxic
Cat 3. The argument was that no metals have been classified for this endpoint so far. DK pointed out that the main
problem was the absence of relevant data for the metal. However there is evidence for such effect for soluble nickel
substances.
Draft RAR, 2005: No relevant studies regarding nickel metal were available. However, read-across from nickel
compounds was considered. The basic assumption is that after intake, nickel compounds (and metallic nickel) are
changed and the nickel ion is the determining factor for the reproductive toxicity. Based on consistent evidence of
developmental toxicity, the soluble (nickel sulphate, nickel chloride, nickel nitrate) and sparingly soluble (nickel carbonate) compounds and should be classified for developmental toxicity in category 2 with R61. However, since the potential release and absorption of nickel from metallic nickel is substantially lower than from the soluble compounds via all routes, it was agreed that metallic nickel should not be classified for this effect.
Draft RAR, 2006 (R308_0605_hh.doc) and comments from BfR (these comments will be included in the next
draft for the Final written procedure):
Human health effects: For some toxicological endpoints only few data are available. Therefore, information on
hydroxylamine hydrochloride is also considered. The read-across is justified due to the following reasons: BHAS
is a solid salt of hydroxylamine and sulfuric acid. Hydroxylamine hydrochloride is a crystalline salt of
hydroxylamine and hydrochloric acid. Selected physicochemical properties of both compounds are used as
reasoning for the read-across, namely molecular weight, water solubility, relative density. Both compounds are
completely dissociated in aqueous media in hydroxylammonium cations and the respective anions. The
hydroxylamine moiety represents the toxic species of both compounds. Therefore, both compounds are classified
with the same R phrases for human health effects.
Draft RAR, 2005
Atmospheric degradation: A QSAR in EUSES was used to provide a worst case estimate (atmospheric half-life of
29 days).
Accumulation in earthworms: No experimental data available. The BCF was estimated with a QSAR equation
given in the TGD.
Aquatic compartment: The ECOSAR program was used to obtain QSAR estimates for toxicity to fish,
invertebrates, and algae. These were found to be of the same order of magnitude (slightly higher) than the test data.
The good match between experimental results and QSAR results was used to rebut the suggestion that toxic effects
may have been caused by certain HCCP metabolites.
Final RAR, 2006
Physicochemical properties: Due to inadequacies in the existing data a QSAR estimate of the vapour pressure
pylidenediphenol
using the Syracuse Research Corporation MPBPWIN software was used to support the available measured data.
79-94-7 p-tert-butylphenol (ptBP) 4
Draft RAR, 2006 (R404_env_hh_0606.doc)
Endocrine disruption potential: Read across from similar alkyl phenol compounds, including p-tert-pentylphenol,
which have shown endocrine disrupting properties in vivo, was used to support in vitro data and to conclude that
ptBP has endocrine disrupting potential and should therefore be further tested.
4-tert-butylbenzoic acid
Draft RAR, 2006 (R405_0602_env.doc)
Adsorption: No experimental data were available. The Koc was calculated with the TGD QSAR for organic acids.
Draft RAR, 2004
methylbutane (TAME)
Aquatic toxicity: Comparison of QSAR calculations for toxicity to aquatic species according to the TGD and
ECOSAR (acute toxicity to freshwater fish, marine fish, daphnia and algae) indicated that TAME acts by a non-
specific mode of action. It is not clear from the RAR how this information was used (e.g. if any QSAR estimates
were used in the risk assessment)
Terrestrial environment: Effects on the terrestrial environment were estimated using EUSES-modelling based on
aquatic toxicity data
Hydrolysis: Read across from MTBE, a structurally related aliphatic ether, supported the conclusion that TAME is
not expected to significantly hydrolyze in natural waters under environmentally relevant pH conditions.
Exposure: Read-across from MTBE was used to estimate the exposure to TAME by inhalation and from tap water
in assessing the indirect exposure via the environment.
Ozone creation: A study by Derwent et al. (1998), reporting the photochemical ozone creation potential of MTBE,
was used to indicate that TAME is considered a low or negligible contributor.
Risk reduction strategy: In TC NES IV '05 (mi_ETBE_tc0504_env_ hh_dr1), there was agreement that the risk
reduction strategy might be considered as valid for all three structurally related ethers (MTBE, TAME and ETBE ),
irrespective of the actual compound. This reflects a read-across for the conclusions of a risk assessment, rather than
a read-across of any form of data.
Draft RAR, 2006 (R418_0602_env_rev1.doc)
Persistence: Read across from HHCB was used in combination with available data to support the conclusion that
2-naph-thyl)ethan-1-one
AHTN does not meet the persistence criterion.
(AHTN) 1506-02-1 Nickel chloride
(nickel dichloride)
Inhalation: There were no available animal data of systemic effects following repeated inhalation of nickel
chloride. An in-depth review by the DK EPA (2005) reported evidence that the long-term inhalation of insoluble as well as soluble nickel compounds results in adverse effects on the lungs. A LOAEC for repeated dose toxicity via inhalation, based on the data nickel sulphate, was used in the risk characterization of nickel chloride. Based on data from other nickel compounds, supported by the nickel chloride-specific data on macrophage effects in rabbits, the C&L Health Effects Group has agreed to classify nickel chloride as T; R48/23
Skin and eye irritation: No skin or eye irritation data on nickel chloride have been found. Instead a read across
from nickel sulphate and nickel nitrate is used. Nickel sulphate was not eye irritating in an animal study, whilst
nickel nitrate was a severe eye irritant (Xi; R41). Considering that nickel chloride has no oxidising potential as
nickel nitrate and that the solubility of the substance is comparable to that of nickel sulphate, it was concluded that
the eye irritating potential of nickel chloride will not exceed that of nickel sulphate. The C&L Health effects group
has agreed not to classify nickel chloride as an eye irritant. Similarly, for skin irritation, the information available
shows that nickel sulphate was not irritant to animals. However, human data do show skin irritation, and
classification is considered relevant. Animal data for nickel nitrate show that this soluble nickel compound is also a
skin irritant. Based on the data for these two soluble nickel compounds, the C&L Health Effects Group has agreed
to classify nickel chloride as Xi; R38 with a specific concentration limit of 20%.
Sensitization: Nickel metal is a skin sensitiser in humans and should be classified as R43. Nickel sulphate is
already classified as a skin and respiratory sensitiser (R43/42). It has been agreed that nickel chloride, nickel nitrate
and the nickel carbonates should also be classified as skin and respiratory sensitisers (R43/42).
Nickel carbonate
Carcinogenicity: An Industry derogation statement (Laine, 2003d) indicated that the carcinogenicity should be
evaluated by using the worst-case read-across from soluble and insoluble nickel compounds. Since both water-
soluble nickel compounds and insoluble inorganic nickel compounds are considered as human carcinogens, nickel
carbonate was also considered to be a human carcinogen.
Toxicity for reproduction: No relevant studies regarding nickel carbonate had been found. From review
documents prepared by the DK EPA, relevant data were identified for other nickel compounds. These data were
used to classify soluble nickel compounds as Repr. Cat. 2; R61. In addition, the TC C&L also agreed to classify the
sparingly soluble nickel carbonate as Repr. Cat. 2; R61. The assumption was made that after intake nickel
compounds (including nickel carbonate) are changed and that it is the nickel ion that is the determining factor for
the reproductive toxicity.
Irritation /corrosivity:
Eye irritation: No skin or eye irritation data on nickel carbonate have been found. Data on eye irritation is
available for nickel sulphate and nickel nitrate. Nickel sulphate was not eye irritating in an animal study, whilst
nickel nitrate was a severe eye irritant (Xi; R41). Considering that nickel carbonate has no oxidising potential as
nickel nitrate and considering that the solubility of the substance is limited compared to nickel sulphate, it was
supposed the eye irritating potential of nickel hydroxy carbonate doesn't exceed that of nickel sulphate. The C&L
Health effects group has agreed not to classify nickel hydroxycarbonate as an eye irritant.
Skin irritation: Information on nickel sulphate is available, which shows that nickel sulphate was not irritant to
animals. However, human data do show skin irritation, and classification is considered relevant. Animal data for
nickel nitrate show that this soluble nickel compound is also a skin irritant. Based on the data for these two soluble
nickel compounds, the C&L Health Effects Group has agreed to classify nickel carbonate as Xi; R38 with a specific
concentration limit of 20%.
Sensitisation: No data regarding skin sensitisation or elicitation in humans have been located. Based on data from
other nickel compounds nickel carbonate may cause sensitisation, under conditions where the nickel ion is released.
The C&L Health Effects Group has agreed to confirm the current classification of nickel carbonate with R43.
In the same way, based on data for other related nickel compounds it is concluded that nickel carbonate is a
respiratory sensitiser. The C&L Health Effects Group has agreed to classify nickel carbonate as R42.
Repeated dose toxicity:
Inhalation: No data on repeated dose toxicity of nickel carbonate via inhalation have been found. It appears that
long-term inhalation of nickel compounds insoluble as well as soluble nickel compounds results in adverse effects
on the lungs including chronic inflammation and fibrosis. A LOAEC identified in the 2-year rat study of nickel
sulphate is used. Based on data from other nickel compounds, the C&L Health Effects Group has agreed to classify
nickel nitrate as T; R48/23.
Oral: Sufficient oral repeated dose toxicity data are available for nickel sulphate (oral LOAEL of 6.7 mg Ni/kg
bw/day based on reduced body weight and increased mortality and a NOAEL of 2.2 mg Ni/kg bw/day). These data
are not in conflict with the database on nickel carbonate, and are considered relevant for the risk assessment of
nickel carbonate. The effects following repeated oral administration of nickel compounds in general do not lead to
a need for classification.
Nickel dinitrate
Acute inhalation toxicity: Based on the oral toxicity, and knowing that the absorption via inhalation is
considerably greater than via the oral route, and further considering the observed lethality in a 16-days inhalational
study with nickel sulphate, the C&L Health Effects Group has agreed to classify nickel nitrate as Harmful with Xn; R20.
Repeated dose toxicity:
See the section for nickel carbonate.
Sensitisation: Nickel nitrate can elicit an allergic reaction in nickel sensitive humans. Based on data from other
nickel compound it may also cause sensitisation. The C&L Health Effects Group has agreed to classify nickel
nitrate as R43 with a specific concentration limit of 0.01%.
Draft RAR, 2005 (R425_0508_env.doc)
methylethyl) phosphate
Aquatic toxicity: QSAR predictions of aquatic toxicity are included in the risk assessment as supporting
information. The reasonable agreement between the measured acute fish LC50 and the QSAR predictions (SRC
ECOSAR predictions with measured physicochemical data entered) leads to the conclusion that the substance appears to be behaving in a predictable way. This was used as an argument to build the case not to classify the substance as dangerous to the environment R52-53.
Draft RAR, 2006 (R425_0610_ENV)
Terrestrial microorganisms: Due to the structural similarity between TCPP and TDCP, a read-across of data from
soil micro-organism studies (NOEC for effects on soil nitrogen transformation effects) from TDCP was performed.
This resulted in a lower assessment factor for the derivation of PNECsoil for TCPP.
Absorption: The Koc value for TCPP was calculated using an empirical relationship that was derived from the
measured log Kow and log Koc.for TDCP and read-across to TCPP. The log Koc for TCPP was calculated by
applying this same relationship and the log Kow for TCPP.
Draft RAR, 2006 (R425_0701_HH.doc)
Carcinogenicity: The acceptability of a read-across approach (qualitatively and/or quantatively) to other
chlorinated alkyl phosphates (TCEP and TDCP) to address the carcinogenicity of TCPP was discussed. Further
justification on the acceptability or not of this approach should be provided in the risk assessment report. Therefore,
as yet no agreement on the read-across for carcinogenicity for TCPP has been reached by TC NES.
Draft RAR, 2006 (R426_0508_env.doc)
methyl)ethyl]phosphate
Aquatic toxicity: QSAR predictions of aquatic toxicity are included in the risk assessment as supporting
Draft RAR, 2006 (R426_0601_HH.doc)
Flash point (closed cup): No closed cup result was available. Read-across from TCPP (EA 2004a) suggested that
the result is likely to be above 245°C
2,2-bis(chloromethyl)
Draft RAR, (R428_0701_HH)
trimethylene bis(bis(2-
Carcinogenicity: It is accepted that when compared with the other chlorinated alkyl phosphates (TCEP, TCPP and
chloroethyl)phosphate)
TDCP), V6 is a much bulkier molecule. Thus, so far V6 has been treated as a stand alone risk assessment. As the
repeated dose toxicity data on V6 are limited it was agreed at TC NES I ‘07 to await the outcome of ongoing 2-
generation reproductive toxicity study on V6 (and TCPP) before drawing a conclusion on carcinogenicity. In
addition, as with TCPP, further justification on the acceptability or not of a read-across to other chloroalkyl
phosphates should be included in the report. Therefore, as yet no agreement on the read-across or not for
carcinogenicity for V6 has been reached by TC NES.
R428_0508_env (R428_0508_env.doc)
Aquatic toxicity: QSAR predictions of aquatic toxicity are included in the risk assessment as supporting
information. The reasonable agreement between the measured acute fish LC50 and the QSAR predictions
(ECOSAR predictions with measured physicochemical data entered) led to the conclusion that the substance
appears to be behaving in a predictable way. This was used as an argument to build the case not to classify the
substance as dangerous to the environment R52-53.
Draft RAR, 2006 (R428_0610_ENV)
Absorption: The Koc value for V6 was calculated using an empirical relationship that was derived from the
measured log Kow and log Koc.for TDCP and read-across to V6. The log Koc for V6 was calculated by applying this
same relationship and the log Kow for V6.
Higher plants: No data are available on the toxicity of V6 to higher plants. Data are however available for the
structurally related substances TCEP, TCPP and TDCP.
Terrestrial compartment:Terrestrial micro-organism: No test data are available on the toxicity of V6 to terrestrial
microorganisms. These data are however available for TDCP and a 28-day NOEC (NOEC for effects on soil
nitrogen transformation effects) has been determined. PNECsoil: in risk characterization for agricultural soil,
PEC/PNECsoil ratio was calculated also by using the PNECsoil derived for the structurally similar compound
TCPP. This was used for comparison with the PEC/PNECsoil derived by equilibrium partitioning. The later was
more conservative and considered to be the most sound.
Physico-chemical properties: A QSAR estimation of vapour pressure was performed using EPIWIN Version 3.05.
Measured data for TCPP and TDCP were used to validate the estimation. There was sufficiently close agreement between the measured vapour pressures for these two substances and the value calculated by the QSAR equation that the calculated value was considered to be acceptable.
Draft RAR, 2004
methylbutane (TAME)
Aquatic toxicity: QSAR calculations compared with the aquatic test results indicater that TAME acts by a non-
polar mode of actionin awuatic species thus justifying the use of lower assessment factor for PNEc derivation.
Draft RAR, 2005 (R413_0602_hh_final.doc)
Carcinogenicity: Results are available only rom a single oral carcinogenicity study. However, lack of alert from
molecule structure and results of carcinogenicity testing for the related substance MTBE, suggest that
carcinogenicity is not an endpoint of concern.
Footnotes:
1 PL = Priority List
2 Rap = rapporteur
3 Information sources: ECB website: http://ecb.jrc.it/esis/ or ECB internal record (from shared drive)
Abbreviations:
AHTN:
Bioconcentration Factor
Bis(hydroxylammonium) sulphate
Benzothiazolsulphonic acid
Dibutyl phthalate
Bis(2-ethylhexyl) phthalate
Di (isodecyl) phthalate
Di (isononyl) phthalate
European Union System for the Evaluation of Substances
Chlorodifluoromethane
Carbon-water partition coefficient
Methyl tert-butyl ether
Penta and hexa brominated biphenyls
Penta and hexa chlorinated biphenyls
Diphenyl ether, pentabromo derivative
Quantitative Structure – Activity Relationship
Risk Assessment Report
Tris (2-chloro-1-methylethyl) phosphate
Technical Guidance Document
Technical meeting
Summary of Discussions on the Use of QSARs, Read-Across and Grouping
in the Technical Committee for Classification and Labelling (TC C&L)
Ana Gallegos, Ingrid Langezaal & Andrew Worth
European Chemicals Bureau
28 February 2007
This document gives an overview of examples of substances for which non-testing methods (QSARs, read across and grouping) have been mentioned or used for classification and labelling of chemical substances by the Technical Committee for Classification and Labelling. Read-across has been used extensively in the discussions in the Technical Committee for Classification and Labelling and its predecessor, the Commission Working Group on the Classification of Dangerous Substances and the use of this approach has been summarised in the TAPIR report available on the ECB website. In addition, other descriptions of the use of a group approach to the classification of substances have been published by Comber & Simpson (2007)1, Hart (2007)2 and Hart & Veith (2007)3. The Technical Committee for Classification and Labelling (TC C&L) gives recommendations for EU harmonised classification and labelling of dangerous substances. For health effects such recommendations are usually based on weight of evidence. Read across is mostly used as a part of the weight of evidence. All substances for which QSARs, read across or grouping were mentioned in the documentation relevant to the substance are listed in the tables below. The tables include substances and endpoints for which the use of non-testing approaches was agreed, as well as substances/endpoints for which the non-testing approaches were not accepted.
1 Comber M & Simpson B. (2007). Grouping of Petroleum substances. p. 118 of this publication. 2 Hart J (2007). Nickel compounds – a category approach for metals in EU Legislation. A report to the Danish Environmental Protection Agency. Available at www.mst.dk/ 3 Hart J & Veith GD (2007): Applying Chemical Categories to Classification & Labelling: A Case Study of Volatile Aliphatic
SUMMARY OF DISCUSSIONS ON THE USE OF QSARs, READ-ACROSS AND GROUPING IN THE TECHNICAL COMMITTEE
FOR CLASSIFICATION AND LABELLING (TC C&L)
1) Human health effects (examples identified by screening electronic archives in ECB)
Substance
References
Approach
Discussions on use of non-testing approaches
discussed
ECBI/69/03, Add. 1, Add. 2(I and II),
Proposal based on ESR review.
A case study (Hart, 2007) describes in detail the discussions that led to
the agreement on the classification of nickel compounds.
ECBI/80/04 and Adds.
Conclusion:
The classification for nickel sulfate is included in the 30th ATP of
ECBI/52/04 Revision 3 (web
Directive 67/548/EEC.
ECBI/102/04 Revision 6 (web
Comment: The classification proposal for nickel sulfate is based on data
ECBI/147/04 Rev. 3
for all classified endpoints (see agreed RA report for nickel sulfate).
(summary record, ECB archive)
Nickel sulfate is therefore a "source chemical" according to RIP 3.3
draft guidance terminology
Proposal based on ESR review.
(a) Nickel dinitrate
ECBI/80/04 and Adds.
A case study (Hart, 2007) describes in detail the discussions that led to
the agreement on the classification of nickel compounds.
(b) Nitric acid, nickel salt
ECBI/102/04 Revision 6 (web
Conclusion:
The classification for nickel nitrate is included in the 30th ATP of
ECBI/109/04 Rev. 3 (summary
Directive 67/548/EEC.
record, ECB archive)
Comment: The classification proposal for nickel nitrate is based on a
mixture of data for some endpoints and read-across for others. Nickel
nitrate is therefore both a "source chemical" and a "target chemical"
according to RIP 3.3 draft guidance terminology, depending on
endpoint.
Nickel dichloride
Proposal based on ESR review.
ECBI/80/04 and Adds.
A case study (Hart, 2007) describes in detail the discussions that led to
the agreement on the classification of nickel compounds.
Substance
References
Approach
Discussions on use of non-testing approaches
discussed
ECBI/102/04 Revision 6 (web
Conclusion:
The classification for nickel dichloride is included in the 30th ATP of
ECBI/109/04 Rev. 3 (summary
Directive 67/548/EEC.
record, ECB archive)
Comment:
The classification proposal for nickel chloride is based on a mixture of
data for some endpoints and read-across for others. Nickel chloride is
therefore both a "source chemical" and a "target chemical" according to
RIP 3.3 draft guidance terminology, depending on endpoint.
Nickel carbonate
Proposal based on ESR review.
(a) Carbonic acid, nickel (2+) salt
ECBI/80/04 and Adds.
A case study (Hart, 2007) describes in detail the discussions that led to
(b) Carbonic acid, nickel salt
the agreement on the classification of nickel compounds.
(c) [µ-[carbonato(2-)-O:O']] dihydroxy
ECBI/102/04 Revision 6 (web
Conclusion:
The classification for nickel carbonate is included in the 30th ATP of
ECBI/109/04 Rev. 3 (summary
Directive 67/548/EEC.
(d) [carbonato(2-)] tetrahydroxytrinickel
record, ECB archive)
Comment: There is quite limited data for nickel carbonate, with test
data for acute oral toxicity, limited data for mutagenicity, and very
limited animal carcinogenicity studies. The producers sent a derogation
statement that was subsequently accepted by the TC NES that they
would accept a "worst-case" classification. Nickel nitrate is therefore a
"target chemical" according to RIP 3.3 draft guidance terminology, for
all endpoints except acute oral toxicity.
ECBI/70/04 ECBI/147/04 Revision 3
Proposal based on ESR review.
(web summary record)
A case study (Hart 2007) describes in detail the discussions that led to
the agreement on the classification of nickel compounds.
Conclusion:
The classification for nickel is included in the 30th ATP of Directive
67/548/EEC. The classification proposal for nickel is based on data for
all classified endpoints. There is only limited data on the carcinogenicity
of nickel metal, and read-across was not used for this endpoint. Further
testing for this endpoint is being carried out.
Substance
References
Approach
Discussions on use of non-testing approaches
discussed
Category approach for nickel compounds
ECBI/96/04 Adds. 2 , 2rev1, 4,
Proposal:
6, 7, 8, 9, 10, 11, 12, 13, 14(I, II),15,
A case study (Hart 2007) describes in detail the discussions that led to
16, 17, 18 and 19 and 25
the agreement on the classification of nickel compounds.
ECBI/29/05, Revision 5 (web
Conclusion: The category approach was agreed for a large number of
nickel compounds. Classification proposals for individual category
members were agreed for inclusion in 31st ATP of Directive
See Schoeters I & Veroustraete V
(2007). Case studies: metals & inorganic metal compounds. (later in this document)
n-propyl bromide / 1-bromopropane (NPB)
Documents ECBI/89/01 + addenda.
Reproductive toxicity:
Documents under ECBI/67/02 +
Read across from 2-bromopropane to 1-bromopropane was mentioned
but thought to be inappropriate. Data submitted by industry showed that
ECBI/60/05 Rev. 3 (summary record,
n-propyl bromide on its own merited classification in Repr. Cat. 2. The
SAR with 2-bromopropane was not decisive for C&L.
ECBI/90/06 Rev. 1 (draft summary
record, ECB archive)
Perfluorooctane sulfonate (PFOS) and its
ECBI/24/05 Add. 1 and 2, Rev. 1
Acute, repeat dose and developmental toxicity: The plausibility of
ECBI/29/05, Revision 5 (web
read across between PFOS and its salts was discussed for acute toxicity
(Xn; R20/22), repeated-dose toxicity (T; R48/25) and reproductive
ECBI/60/05 Rev. 3 (summary record,
(developmental) toxicity (R61).
ECB archive) ECBI/90/06 Rev. 1 (draft summary record, ECB archive)
2-methoxy-1-methylethyl acetate (PGMA)
ECBI/125/04, Rev. 1
Respiratory irritation and narcosis:
ECBI/146/04 Rev. 2
Read across of R37 (respiratory irritation) and R67 (vapour may cause
(web summary record)
drowsiness) from 1-methoxy-2-propanol (PGME) was proposed but not
ECBI/55/05 (summary record, ECB
agreed on the basis that there were data indicating the absence of these
Hydroxylammonium salts
ECBI/14/03 Add. 7 , 8 and 9
(a) Hydroxylammonium hydrogensulfate
ECBI/16/03 and Add. 1 (I,II)
Explosivity, carcinogenicity, irritation, acute toxicity, chronic
ECBI/146/04 Rev. 2
toxicity, aquatic toxicity:
(b) Hydroxylamine phosphate
(web summary record)
The group agreed to classify the hydroxylammonium salts (except the
nitrate, the chloride and Bis(hydroxylammonium)sulfate) for explosivity
(c) Hydroxylamine dihydrogenphosphate
(summary record, ECB archive)
(R2), carcinogenicity (R40), irritation (R36/38), acute toxicity (R21/22),
chronic toxicity (R48/20) and aquatic toxicity (R50).
Substance
References
Approach
Discussions on use of non-testing approaches
discussed
(d) Hydroxylamine 4-methylbenzenesulfonate CAS: 53933-48-5 Perboric acid, sodium salts
ECBI/38/03 Add.1 and Add. 2
Reproductive toxicity:
Summary Record from the Specialised support to data.
It was proposed to classify sodium perborate for reproductive toxicity
Perboric acid, sodium salt monohydrate
Experts meeting ECBI/132/04 Rev. 2
(Repr. Cat. 2 R61 for development and Repr. Cat. 3 R62 for fertility).
ECBI/146/04 Rev. 2
Perboric acid, sodium salt Tetrahydrate
(web summary record)
For developmental effects the Specialised Experts (SE) agreed that
based on 1 rat study sodium perborate should be classified with Repr.
(summary record, ECB archive)
Cat. 2; R61. This was supported by the evidence that sodium perborate is
converted to boric acid, which is a developmental toxicant with a
recommended classification as Repr. Cat. 2; R61.
Upon the SE advice, the member states expressed differing views on the
developmental effects since there was a limited amount of data and the
read across to boric acid was not accepted by all Member States.
The animal data on fertility were very limited, and alone were
insufficient for classification. However, evidence that sodium perborate
is converted to boric acid, which has a recommended classification as
Repr. Cat. 2; R60, suggested that sodium perborate may affect fertility
and on this basis the Specialised Experts recommended classification as
Repr. Cat. 3; R62.
Some Member States argued, upon the SE advice, that the comparison
with boric acid was inappropriate and that there was actually very
limited direct evidence of an effect, particularly in terms of fertility. The
SE advice was accepted with strong reservation from some Member
States.
Alkanes, C10-13, Chloro (short-chained
ECBI/36/02, Adds. 1, 2, 3, 4 and 5
Toxicity via lactation:
chlorinated paraffins; SCCPs)
Extract of summary record
The possibility of reading across toxicity via lactation (R64) from
Specialised Experts meeting:
MCCPs was raised, but the discussion was postponed pending
ECBI/08/04 Rev. 2
discussions in the TCNES.
ECBI/102/04 Revision 6 (web
ECBI/147/04 Rev. 3 (web summary record)
Substance
References
Approach
Discussions on use of non-testing approaches
discussed
Alkanes, C14-17, Chloro (medium-chained
ECBI/35/02, Adds. 1, 2, 3 and 4
Carcinogenicity: Specialised experts recommended not to read across
chlorinated paraffins; MCCPs)
Extract of summary record
carcinogenicity (R40) from SCCPs. This conclusion was questioned by
Specialised Experts meeting:
S, but the group agreed to follow the recommendation. It was therefore
ECBI/08/04 Rev. 2
agreed not to classify for carcinogenicity.
ECBI/102/04 Revision 6 (web summary record) ECBI/147/04 Rev. 3 (web summary record)
1,2-Diethoxyethane
ECBI/15/03 and Add. 2, 3 and 4
Reproductive toxicity: In discussing the classification for reproductive
ECBI/102/04 Revision 6 (web
(developmental) toxicity (R63), there were different views as to whether
the classification should be with Cat. 2 or Cat. 3. The majority view was
ECBI/109/04 Rev. 3 (summary
to assign Cat. 3, due to uncertainty arising from use of read-across.
record, shared drive)
Phthalate esters:
ECBI/15/02 Rev. 3
Reproductive toxicity: In discussing the classification for reproductive
(web summary record)
toxicity (R63), it was noted that these phthalate esters follow a trend in
(a) 1,2-benzenedicarboxylic acid, di-C7-11-
which the severity of the effects depend on the degree of branching and
branched and linear alkylesters
the chain length (the lower the chain length and the less the branching,
the greater the effect).
(b) 1,2-benzenedicarboxylic acid, di-C7-9-
It was agreed to classify (a) with Repr. Cat.2; R61 and with Repr. Cat. 3;
branched and linear alkylesters
R62. It was also agreed to exclude (b) and (c).
CAS: 68515-41-3 (c) 1,2-benzenedicarboxylic acid, di-C9-11-branched and linear alkylesters CAS: 68515-43-5 2-Ethylhexyl-2-ethylhexanoate
Proposal: No classification
ECBI/19/05 Rev.2
Discussion: QSAR results promised by S in follow-up
(web summary record)
ECBI/48/05 Rev.1
R63 classification was based on data. SAR to 2-ethylhexanoic acid was
(summary record, ECB archive)
used as an additional argument
Conclusion: No classification (provisional)
2) Environmental effects (examples identified by screening electronic archives in ECB)
Substance
References
Approach
Classification proposal, discussion and conclusion
Tris(2-chloro-1-methylethyl) phosphate
ECBI/128/04 Add. 1
Discussion: No data for acute toxicity in fish, but read-across (from
ECBI/141/04 Add. 2
algae) and QSAR for fish toxicity supported
ECBI/19/05 Rev.2
no classification. It was agreed not to classify, but some MS expressed
(web summary record)
a preference for R52-53
ECBI/48/05 Rev.1
(summary record, ECB archive)
Conclusion: No classification
ECBI/74/02 Add. 1
Proposal: No classification based on lack of data
ECBI/19/05 Rev.2
Discussion: DK sought QSAR estimates, but considered the models to
(web summary record)
be unreliable. E reported an estimate of not biodegradable according to
ECBI/48/05 Rev.1
EPIWIN QSAR model.
(summary record, ECB archive)
Conclusion: Decision postponed, awaiting revised proposal
Perfluorooctane acetate (PFOA)
Proposal: No classification
Discussion: TC C&L considered use of QSAR to be unclear.
ECBI/19/05 Rev.2
(web summary record)
Conclusion: Decision postponed, awaiting additional information.
ECBI/48/05 Rev.1 (summary record, ECB archive)
Proposal: N; R50-53
Classification proposal based on the application of QSAR models
ECBI/42/05 Rev.1
(Episuite 3.1) to predict toxicity and non-biodegradability (ECBI/42/05
ECBI/19/05 Rev.2
(web summary record)
ECBI/48/05 Rev.1
Conclusion: N; R50-53
(summary record, shared drive)
ECBI/113/04 Add. 1
Proposal: No environmental classification
ECBI/19/05 Rev.2
(web summary record)
Discussion: DK reported QSAR results, but they considered them
ECBI/48/05 Rev.1
unreliable because complex substance.
(summary record, ECB archive)
Conclusion: No classification based on lack of data
ECBI/72/04 Add. 1
Proposal: No environmental classification
tetrayltetraamine
ECBI/19/05 Rev.2
(web summary record)
Discussion: UK considered that read across from other benzidines was
ECBI/48/05 Rev.1
not applicable. DK reported QSAR results for fish toxicity but
(summary record, shared drive)
considered them unreliable
Conclusion: No classification based on lack of data
Proposal: N; R51-53
ECBI/11/04 Rev. 4
(web summary record)
Discussion: IND disagreed with R53 (possible long-term effects) on
ECBI/64/04 Rev. 2 (summary record,
grounds of data showing ready biodegradability, supported by QSAR
data.
Conclusion: N; R51-53
Tert-butyl hydroperoxide (TBHP)
Discussion: Not readily biodegradable based on the standard test, but
ECBI/40/03 Add.1
inherently degradable based on QSAR data. Test data took precedence
R319_0302_env26.02.03
(Draft Risk Assessment Report)
ECBI/26/03 Rev.5
Conclusion: N; R51-53 and not readily degradable.
(web summary record) ECBI/50/03 (summary record, shared drive)
5-tert-butyl-2,4,6-trinitro-m-xylene (Musk
R322_0112_env_hh 20.12.01
Proposal: N; R50-53
(Draft Risk Assessment Report)
ECBI/16/02 Add.1
Discussion: Classification based on data. R50 was supported by QSAR
Follow-up Documents after June
meeting: Add.1 Rev.1 , Add.5
ECBI/61/02 Rev.3
Conclusion: N; R50-53
(web summary record)
ECBI/71/02 Rev.4 (summary record, ECB archive)
4,4'-bis(dimethylamino) benzophenone
ECBI/56/00 Rev.1 and Add.3, 4
Proposal: N; R51-53
(Michler's ketone)
(confidential), 5, 6, 7
ECBI/32/01 Rev.4 (web summary
Discussion: The validity of solubility and fish test data were discussed.
A disagreement was noted between QSAR and experimental data for
ECBI/47/01 Rev.2
fish toxicity. In addition, QSAR data indicated higher toxicity data for
(summary record, ECB archive)
daphnia and algae. This led to a discussion over whether to assign no
classification due to lack of data or to classify based on QSAR data.
Subsequently, the validity of solubility data was confirmed and
daphnid data was provided. It was agreed not to classify.
Conclusion: No classification based on data
Tallow alkyl amine
ECBI/04/05 Add. 7
Proposal: R50/53
(C14-C18 alkylamines)
ECBI/04/05 Add. 14
R53 based on calculated log Kow= 7.1
ECBI/19/05 Rev.2 (web summary
BCF was estimated by read-across from ionic surfactants and by
applying QSARs (Bintein and Syracuse)
ECBI/48/05 Rev.1
(summary record, ECB archive)
Conclusion: Provisionally classified N; R50-53
ECBI/37/05 Add. 1
Discussion: Due to lack of data, UK proposed to read-across aquatic
benzylidenedianiline (Leucomalachite
ECBI/19/05 Rev.2 (web summary
toxicity and (non-ready) biodegradability from malachite green to
leucomalachite green. Since leucomalachite green is ionised in water,
ECBI/48/05 Rev.1
its ionised form is similar to malachite green.
(summary record, ECB archive)
Conclusion: Classification N, R50-53, S60-61
Tris(2-chloro-1-methylethyl) phosphate
Draft RAR: R425_0508_env
Discussion:
Data presented in the RAR report are consistent with no classification
for the environment being necessary. The fish, Daphnia and algae
acute E(L)C50 values all fall in the range 10 to 100 mg/l, and there is
no evidence of ready degradability in standard tests. However, R52-53
is not applicable for TCPP for the reasons outlined below:
Reliable chronic NOECs are now available for invertebrates and
Commission Working Group on the
algae and both are well above 1 mg/l (32 and 23 mg/l
Classification and Labelling of
respectively). The acute-to-chronic ratios are 4 and 3.6
Dangerous Substances Meeting on
Environmental Effects of Existing
The acute effect concentrations range from 51 to 131 mg/l (fish and
Chemicals, Pesticides & New
Daphnia respectively). The difference in acute susceptibility across
Chemicals September 28-30, 2005
the taxa is therefore actually quite small (approximately 3-fold)
The tests have been conducted well below the water solubility
ECBI/128/04 Add. 1 (web summary
limit (1080 mg/l), and the low measured BCF values do not
record) and ECBI/141/04 Add. 2 (web
suggest that the substance will accumulate over long periods. The
summary record);
acute toxicity therefore probably reflects the effect of uptake at
ECBI/48/05 Rev.1 (summary
steady state (i.e. not just partial uptake)
record, ECB archive) and ECBI/55/05
There is reasonable agreement between the measured acute fish
(summary record, ECB archive)
LC50 (51 mg/l) and QSAR predictions (11-21 mg/l, using SRC
ECOSAR with measured physicochemical data entered). The substance therefore appears to be behaving in a predictable way
There is no indication of neurotoxicity in this chemical class from mammalian and avian studies
There is therefore no reason to suppose that there will be a significant difference in chronic effects in fish compared to the other taxa
Therefore, applying the Daphnia acute-to-chronic ratio to the acute fish result would give a NOEC of approximately 4.5 mg/l. This is
very similar to the QSAR estimate of 5.2 mg/l (using SRC ECOSAR with measured physicochemical data entered)
The acute-to-chronic ratio would be above 50 if the fish NOEC were below 1 mg/l, which is clearly out of line with the observations for Daphnia and algae.
Conclusion: Not classified as dangerous to the environment R52-53.
Draft RAR: R428_0508_env
Discussion:
chloroethyl)phosphate) (V6)
Commission Working Group on the
Data presented in this report are consistent with no classification for
Classification and Labelling of
the environment. The fish, Daphnia and algae acute E(L)C50 values all
Dangerous Substances Meeting on
fall in the range 10 to 100 mg/l, and there is no evidence of ready
Environmental Effects of Existing Chemicals, Pesticides & New
degradability in standard tests. However, R52-53 is not applicable for
Chemicals September 28-30, 2005
V6 for the reasons outlined below:
ECBI/128/04 Add. 1 (web summary
1. Reliable chronic NOECs are available for invertebrates and algae
and both are above 1 mg/l (>3.7 and 10 mg/l respectively). The
ECBI/141/04 Add. 2 (web summary
acute-to-chronic ratios are ≤11.4 and 3.5 respectively.
2. The measured acute data show a similar level of sensitivity across
ECBI/48/05 Rev.1 (summary record,
the three taxonomic groups.
ECB archive) and ECBI/55/05 (summary record, ECB
3. The tests have been conducted well below the water solubility
limit (232 mg/l), and the low log Kow (2.83) does not suggest that the substance will accumulate over long periods (in line with measured BCF data for analogous substances). The acute toxicity therefore probably reflects the effect of uptake at steady state (i.e. not just partial uptake).
4. There is reasonable agreement between the measured acute fish
LC50 (52 mg/l) and QSAR predictions (17-32 mg/l, using SRC ECOSAR with measured physicochemical data entered). The substance therefore appears to be behaving in a predictable way.
5. There is no indication of neurotoxicity in this chemical class from
mammalian and avian studies.
6. There is therefore no reason to suppose that there will be a
significant difference in chronic effects in fish compared to the other taxa.
7. Therefore, applying the worst-case acute-to-chronic ratio for
Daphnia to fish would give a NOEC of approximately 4.5 mg/l. This is very similar to the QSAR estimate of 7.0 mg/l (using SRC
ECOSAR with measured physicochemical data entered).
8. The acute-to-chronic ratio would be above 50 if the fish NOEC
were below 1 mg/l, which is clearly out of line with the observations for Daphnia and algae.
Given these considerations it is unlikely that V6 would be chronically toxic to fish at <1 mg/l and testing to confirm this assertion could not be justified on animal welfare grounds. V6 should not therefore be classified.
Conclusion: Not classified as dangerous to the environment R52-53
Nickel and nickel compounds
ECBI/50/03 (web summary record)
Proposal:
ECBI/48/05 (draft summary record)
A case study (Hart 2007) describes in detail the discussions that led to
ECBI/92/06 Revision 1 (web summary
the agreement on a category approach for nickel compounds.
ECBI/96/04 Add. 2 Rev. 1 and addenda
Conclusion: The category approach was agreed for a large number of
4, 6, 7, 8, 9, 10, 11, 12, 13, 14 Parts I
nickel compounds. Classification proposals for individual category
and II , 15, 16, 21 Parts I and II, 22, 23,
members were agreed for inclusion in 31st ATP of Directive
3) Environmental effects (examples identified by screening the N-CLASS Database on Environmental Hazard Classification; http://apps.kemi.se/nclass/)
Substance
References
Approach
Classification proposal, discussion and conclusion
Propane, 2,2-dimethyl-
ECB1/81/95 Rev. 2
Conclusion: N; R51-53
2-Propanone, 1,1,1,3,3,3-hexachloro-
ECB1/17/97 Rev. 2
Conclusion: N; R51-53
1,3-Isobenzofurandione, 4,5,6,7-tetrachloro-
ECB1/50/96 Rev. 1
Conclusion: N; R50-53
CAS: 117-08-8 1,1'-Biphenyl, 4-nitro-
ECBI/36/97 Add.7
Conclusion: N; R51-53
ECB1/56/97 Rev. 2 ECB1/24/98 Rev. 1
Stannane, chlorotrioctyl-
ECB1/50/96 Rev. 1
Read-across from other organotin compounds
ECB1/56/97 Rev. 2
ECB1/24/98 Rev. 1
Conclusion: N; R53
ECB1/49/98 Rev. 2 ECB1/23/99 Rev. 2
ECB1/81/95 Rev. 2
Conclusion: NC
ECB1/17/97 Rev. 2
Conclusion: NC
2-Pentanone, 4-methoxy-4-methyl-
ECB1/17/97 Rev. 2
Conclusion: NC
3-Pentanone, 2,4-dimethyl
ECB1/17/97 Rev. 2
Conclusion: NC
Salts of oxalic acid.
ECB1/23/96 Rev. 1
Conclusion: N; R51-53
Annex I Index: 607-007-00-3
Phosphoric acid, tris(2-methylphenyl) ester
ECB1/29/97 Rev. 2
Toxicity data and read-across from tricresyl phosphate
ECB1/48/97 Rev. 1
ECB1/56/97 Rev. 2
Conclusion: N; R51-53
ECBI/07/97 Add.11
Phenol, 4-chloro-2-methyl-
ECB1/31/96 Rev. 1
Toxicity data and read-across from 4-chloro-3-methylphenol
ECB1/50/96 Rev. 1
Conclusion: N; R50
Acetaldehyde, chloro-
ECB1/56/97 Rev. 2
Toxicity data and read-across from chloroacetic acid
ECBI/46/95 Add.37
Conclusion: N; R50
Peracetic acid (ethaneperoxoic acid)
ECB1/23/96 Rev. 1
Toxicity data and read-across from acetic acid, the degradation product
ECB1/31/96 Rev. 1
of peracetic acid
ECB1/50/96 Rev. 1
ECB1/17/97 Rev. 2
Conclusion: N; R50
ECBI/90/95 Add.36
ECBI/10/96 Rev. 1
R53 due to lack of evidence of ready biodegradability and read-across
ECBI/89/95 Add.3
(read across compound not given in ECBI/10/96 Rev. 1)
Conclusion: N; R50-53
479 substances were identified, 384 of
which belong to group entries
ANNEX 1: LIST OF R-PHRASES
R1: Explosive when dry. R2: Risk of explosion by shock, friction, fire or other sources of ignition. R3: Extreme risk of explosion by shock, friction, fire or other sources of ignition. R4: Forms very sensitive explosive metallic compounds. R5: Heating may cause an explosion. R6: Explosive with or without contact with air. R7: May cause fire. R8: Contact with combustible material may cause fire. R9: Explosive when mixed with combustible material. R10: Flammable R11: Highly flammable R12: Extremely flammable R13 Extremely flammable liquid gas (obsolete) R14: Reacts violently with water. R15: Contact with water liberates extremely flammable gases. R16: Explosive when mixed with oxidizing substances. R17: Spontaneously flammable in air. R18: In use, may form flammable/explosive vapour-air mixture. R19: May form explosive peroxides. R20: Harmful by inhalation. R21: Harmful in contact with skin. R22: Harmful if swallowed. R23: Toxic by inhalation. R24: Toxic in contact with skin. R25: Toxic if swallowed. R26: Very toxic by inhalation. R27: Very toxic in contact with skin R28: Very toxic if swallowed. R29: Contact with water liberates toxic gas. R30: Can become highly flammable in use. R31: Contact with acids liberates toxic gas. R32: Contact with acids liberates very toxic gas. R33: Danger of cumulative effects. R34: Causes burns. R35: Causes severe burns. R36: Irritating to eyes. R37: Irritating to respiratory system. R38: Irritating to skin. R39: Danger of very serious irreversible effects. R40: Possible risk of cancer. R41: Risk of serious damage to eyes. R42: May cause sensitization by inhalation. R43: May cause sensitization by skin contact. R44: Risk of explosion if heated under confinement. R45: May cause cancer. R46: May cause heritable genetic damage. R47: May cause deformities (obsolete). R48: Danger of serious damage to health by prolonged exposure. R49: May cause cancer by inhalation. R50: Very toxic to aquatic organisms. R51: Toxic to aquatic organisms. R52: Harmful to aquatic organisms. R53: May cause long-term adverse effects in the aquatic environment. R54: Toxic to flora. R55: Toxic to fauna. R56: Toxic to soil organisms. R57: Toxic to bees. R58: May cause long-term adverse effects in the environment. R59: Dangerous for the ozone layer.
R60: May impair fertility.
R61: May cause harm to the unborn child.
R62: Possible risk of impaired fertility.
R63: Possible risk of harm to the unborn child.
R64: May cause harm to breastfed babies.
R65: Harmful: may cause lung damage if swallowed.
R66: Repeated exposure may cause skin dryness or cracking.
R67: Vapours may cause drowsiness and dizziness.
R68: Possible risks of irreversible effects.
ANNEX 2: COMBINATIONS OF R-PHRASES
R14/15: Reacts violently with water, liberating extremely flammable gases. R15/29: Contact with water liberates toxic, extremely flammable gas. R20/21: Harmful by inhalation and in contact with skin. R21/22: Harmful in contact with skin and if swallowed. R20/22: Harmful by inhalation and if swallowed. R20/21/22: Harmful by inhalation, in contact with skin and if swallowed. R21/22: Harmful in contact with skin and if swallowed. R23/24: Toxic by inhalation and in contact with skin. R24/25: Toxic in contact with skin and if swallowed. R23/25: Toxic by inhalation and if swallowed. R23/24/25: Toxic by inhalation, in contact with skin and if swallowed. R24/25: Toxic in contact with skin and if swallowed. R26/27: Very toxic by inhalation and in contact with skin. R27/28: Very toxic in contact with skin and if swallowed. R26/28: Very toxic by inhalation and if swallowed. R26/27/28: Very toxic by inhalation, in contact with skin and if swallowed. R36/37: Irritating to eyes and respiratory system. R37/38: Irritating to respiratory system and skin. R36/38: Irritating to eyes and skin. R36/37/38: Irritating to eyes, respiratory system and skin. R39/23: Toxic: danger of very serious irreversible effects through inhalation. R39/24: Toxic: danger of very serious irreversible effects in contact with skin. R39/25: Toxic: danger of very serious irreversible effects if swallowed. R39/23/24: Toxic: danger of very serious irreversible effects through inhalation and in contact with skin. R39/23/25: Toxic: danger of very serious irreversible effects through inhalation and if swallowed. R39/24/25: Toxic: danger of very serious irreversible effects in contact with skin and if swallowed. R39/23/24/25: Toxic: danger of very serious irreversible effects through inhalation, in contact with skin and if swallowed. R39/26: Very toxic: danger of very serious irreversible effects through inhalation. R39/27: Very toxic: danger of very serious irreversible effects in contact with skin. R39/28: Very toxic: danger of very serious irreversible effects if swallowed. R39/26/27: Very toxic: danger of very serious irreversible effects through inhalation and in contact with skin. R39/26/28: Very toxic: danger of very serious irreversible effects through inhalation and if swallowed. R39/27/28: Very toxic: danger of very serious irreversible effects in contact with skin and if swallowed. R39/26/27/28: Very toxic: danger of very serious irreversible effects through inhalation, in contact with skin and if swallowed. R42/43: May cause sensitization by inhalation and skin contact. R48/20: Harmful: danger of serious damage to health by prolonged exposure through inhalation. R48/21: Harmful: danger of serious damage to health by prolonged exposure in contact with skin. R48/22: Harmful: danger of serious damage to health by prolonged exposure if swallowed. R48/20/21: Harmful: danger of serious damage to health by prolonged exposure through inhalation and in contact with skin. R48/20/22: Harmful: danger of serious damage to health by prolonged exposure through inhalation and if swallowed. R48/21/22: Harmful: danger of serious damage to health by prolonged exposure in contact with skin and if swallowed. R48/20/21/22: Harmful: danger of serious damage to health by prolonged exposure through inhalation, in contact with skin and if swallowed. R48/23: Toxic: danger of serious damage to health by prolonged exposure through inhalation. R48/24: Toxic: danger of serious damage to health by prolonged exposure in contact with skin.
R48/25: Toxic: danger of serious damage to health by prolonged exposure if swallowed. R48/23/24: Toxic: danger of serious damage to health by prolonged exposure through inhalation and in contact with skin. R48/23/25: Toxic: danger of serious damage to health by prolonged exposure through inhalation and if swallowed. R48/24/25: Toxic: danger of serious damage to health by prolonged exposure in contact with skin and if swallowed. R48/23/24/25: Toxic: danger of serious damage to health by prolonged exposure through inhalation, in contact with skin and if swallowed. R50/53: Very toxic to aquatic organisms, may cause long-term adverse effects in the aquatic environment. R51/53: Toxic to aquatic organisms, may cause long-term adverse effects in the aquatic environment. R52/53: Harmful to aquatic organisms, may cause long-term adverse effects in the aquatic environment. R68/20: Harmful: possible risk of irreversible effects through inhalation. R68/21: Harmful: possible risk of irreversible effects in contact with skin. R68/22: Harmful: possible risk of irreversible effects if swallowed. R68/20/21: Harmful: possible risk of irreversible effects through inhalation and in contact with skin. R68/20/22: Harmful: possible risk of irreversible effects through inhalation and if swallowed. R68/21/22: Harmful: possible risk of irreversible effects in contact with skin and if swallowed. R68/20/21/22: Harmful: possible risk of irreversible effects through inhalation, in contact with skin and if swallowed.
Read-across Example: Cd & Cd Compounds Human Health
Ilse Schoeters and Violaine Verougstraete
Eurométaux
7 July 2006
Read-across example Cd & Cd compounds human health
The ESR Cd-RA (September 2004) assessed risks associated with exposure to cadmium metal and cadmium oxide. Data with other Cadmium compounds were used as supporting data (‘data on cadmium compounds are included when no (not enough) information on the effects of CdO/Cd metal was available and when the studies using cadmium compounds were mechanistically relevant'). Available data:
Compound
oxide fumes
oxide(dust)
chloride
sulphide
compounds
Solubility (mg/L)
Spherical diameter
Dermal Absorption
Inhalation absorption
Acute inhalation
toxicity (mg/m³)
28.4 (Cd iron
Acute oral toxicity
Acute dermal toxicity
(mg/kg BW)
Irritation respiratory
Irritation: eye, skin
Repro: fertility
Repro: development
RDT bone and kidney
9: data available of good quality 9/-: data available but of limited to poor quality -: no data available
Read-across was applied to derive classification proposals and assess risks for the substances/endpoints shaded in
-for oral absorption of CdO, Cd metal
-for inhalation absorption of Cd metal
-for dermal absorption of Cd metal, CdO
-for acute inhalation toxicity of CdO dust, Cd metal
-for mutagenicity of Cd metal, CdO
-for carcinogenicity of Cd metal
-for reproductive toxicity (fertility) of Cd metal, CdO
-for reproductive toxicity (development) of Cd metal
-for repeated dose toxicity of Cd metal
Digestive absorption rates varying between 0.5 and 12% (on the average 2%) have been reported
according to the animal species and the chemical form of cadmium. The higher values have been
reported for monkeys and large animals compared to rodents. In small animals, absorption in the
range of 1 to 2% is commonly reported (CEC, 1978; Nordberg, 1985).
Little is known about the mechanism of uptake of the various forms of Cd and the transport across
the epithelial cells in the intestine.
It seemed reasonable to assume that gastrointestinal absorption of CdO is not significantly different
from that of other Cd compounds, mainly because of the high solubility of CdO (and probably Cd
metal) in gastric juice (94 %). Therefore, data from studies conducted with other Cd compounds
were judged relevant for assessing the gastro-intestinal absorption of CdO/Cd metal in this RAR.
Overall, it was considered that a large proportion of ingested Cd (including from CdO) is eliminated
in the faeces and that only a few percent (maximum 5%) is absorbed via the gastrointestinal tract.
After inhalation, the alveolar absorption rate of Cd from CdO varies depending on the type of
exposure (fumes>dust; intra-tracheal>inhalation). It is a slow process that continues for many
weeks after a single inhalation exposure (Nordberg et al, 1985). Absorption rates after inhalation of
CdO derived from animal studies range from 50 % (fumes) to 30 % (dust, depending on particle
size).In humans, figures of 10-30 % of absorption rate according to particle size are derived for
CdO dust (Task Group 1973).
Although specific data for Cd metal dust are not available, it appeared reasonable to assume that it
does not differ greatly from CdO.
Acute inhalation toxicity
The acute pulmonary toxicity of cadmium seems to depend on the chemical and physical form of
the administered compound, and therefore the question of the validity of an extrapolation to CdO of
results obtained with other compounds had to be considered in the Cd RA.
Different Cd compounds tested in rats were not equivalent with respect to toxicity: CdO fumes and
Cd carbonate appeared to be more toxic than two cadmium pigments, "cadmium red" (Cd: 69.9%,
Se: 16.4%, S: 13.2%) and "cadmium yellow" (Cd: 77.4%, S: 21.7%, Zn: 0.28%, Se: 0.27%) (Rusch
et al, 1986). Difference in toxicity was attributed to an increased retention and greater absorption
for the more soluble compounds (carbonate and oxide fumes) compared to the highly insoluble
cadmium pigments which have a greater mucociliary clearance.
The CT50 for cadmium oxide dust would be about three to four times the values for cadmium oxide
fumes (Friberg, 1950), and this would be explained by a much longer retention of CdO dust in the
lung (Oberdörster et al.,1992). The retardation of the clearance of the CdO oxide particles may be
due to a slower solubilisation of the particles, compared to that of the fine and very porous fume
particles (Oberdörster, 1992).
On the other hand, some authors warned of predicting the behaviour of compounds in complex
biological systems by their chemical solubility alone (Glaser et al., 1986). For example, based on
the water solubility of CdO and CdS that are very low compared to the highly soluble CdCl2, one
might predict a higher bioavailability of CdCl2 in vivo.
The pulmonary effects of water insoluble cadmium oxide (dust, fumes) were compared with those
induced by water-soluble cadmium chloride in groups of rats exposed by inhalation by Oberdörster
et al. (1987). Small CdO particles and CdCl2 were equally toxic. Authors concluded that acute
effects of Cd compounds in the lung cannot only be predicted from their water solubility
(Oberdörster et al, 1987: the in vivo solubility in the lung after inhalation exposure is very high for
CdO (Oberdörster and Cox, 1990).
Inhaled CdO appeared to be even more damaging to the lung than CdCl2 in the experiment
conducted by Grose et al. (1987) who compared the effects of aerosols of both compounds on the
pulmonary biochemistry and histology in rats and rabbits. Authors concluded that because of the
more acute response of the lung to CdO compared to CdCl2, extrapolation of CdCl2 effects to
potential CdO effects could be scientifically vulnerable (Grose et al, 1987).
For Cadmium metal, no data were available and read-across was done from the CdO data as
exposure is mainly to CdO.
Sensitisation
No test results with cadmium (oxide) as test substance were submitted. A single test with a soluble
cadmium salt (CdCl2) was located in animals (with negative result but insufficient information to
document the test conditions). No read-across was done, no proposal for classification was made.
No study specifically using cadmium metal was located. Since inhalation exposure to cadmium
metal dust is very unlikely in occupational settings, this absence of information is not deemed
critical.
Repeated dose toxicity
Lung: Long-term inhalation exposure of experimental animals to CdO results in similar effects as
seen upon acute exposures, i.e. pneumonia accompanied by histopathologic alterations and changes
in the cellular and enzymatic composition of the broncho-alveolar fluid. Identified NOAELs are:
0.025 mg CdO/m3 in F344/N rats exposed for 13 weeks and 0.01 mg Cd/m3 in hamsters exposed for
16 months.
From the available human studies, a LOAEL of 3.1 µg Cd/l (Cd-U) was derived taking into
consideration that this value is for CdO fumes and may not necessarily apply to CdO or Cd metal
dust.
Bone: Because most of the experimental studies were designed to explore the pathogenesis of Itai-
Itai disease and because animals were generally exposed during a relatively short period with
relatively high doses of Cd water soluble compounds, they do not allow to derive a robust NOAEL
relevant for humans exposed chronically to low doses via the diet or by inhalation.
The kidney is another target organ for cadmium (not specifically Cd metal) toxicity following
repeated exposure by the inhalation and oral routes. Numerous studies in rats, mice, rhesus
monkeys and rabbits have indicated that exposure to cadmium compounds administered orally or by
inhalation causes kidney damage including increase or decrease of relative kidney weight,
histological (necrosis of the proximal tubules, interstitial renal fibrosis) and functional changes
(reduced glomerular filtration rate, proteinuria). On the basis of the most recent human studies
conducted in Europe, a LOAEL of 2 µg/g creatinine is considered for the risk characterisation.
Genotoxicity
No in vitro study using cadmium metal was identified. Bacterial tests with cadmium oxide yielded
negative results. Most of the located in vitro studies used water-soluble cadmium chloride. While,
water solubility does not necessarily reflect in vivo solubility, it was assumed that Cd/CdO will to
some extent be solubilised in vivo, especially in the lung, and data obtained with soluble Cd
compounds were considered relevant to assess the possible genotoxic potential (hazard) of cadmium
oxide.
Although a clear and consistent pattern of action remains to be determined (direct damage by
interacting with the chromatin to generate strand breaks, cross-links or structural alterations in
DNA, and/or indirectly, by depleting antioxidant levels and thereby increasing intracellular
hydrogen peroxide and other oxidants, and/or by interacting at metal-binding sites of proteins
involved in transcription), it was concluded that cadmium metal and oxide can exert a genotoxic
potential in vitro in consideration of several genotoxic effects reported with water soluble cadmium
compounds.
Carcinogenicity
An unequivocal relationship between Cd exposure and lung cancer incidence was demonstrated in chronic inhalation studies in Wistar rats exposed to CdCl2, CdO fumes and CdO dust. In two inhalation studies in rats, malignant lung tumours were produced by cadmium oxide dust and fumes at low levels of exposure for short duration. The lowest dose to produce carcinogenic effects was 30 µg Cd/m³ as cadmium oxide dust as well as cadmium oxide fumes. Groups of rats exposed to cadmium oxide fumes (10 µg CdO/m3, 30 µg CdO/m3) had significantly lower lung tumour incidences than those seen in groups exposed to cadmium oxide dust using the same the exposures modalities. However, these animals had only about half the cadmium content in their lungs compared to animals exposed to the same concentration of CdO dust over the same period, attributed to a lower pulmonary deposition of the chain-like electric arc-generated fume particles (IARC 1993 reporting Oberdörster & Cox, 1989). Mice exposed to equivalent levels of cadmium oxide, had only marginally significant elevations in lung cancer, but the rate of lung cancers in control mice was variable and elevated. No evidence for lung carcinogenicity was found in hamsters, possibly due to lung damage and subsequent decreased survival at high doses. Interspecies but also interstrain differences seem to play a role in the sensitivity to Cd-induced carcinogenesis. Intratracheal instillation of cadmium oxide caused no increase in lung tumours in rats, but did increase the incidence of mammary fibroadenomas. Cadmium oxide is a carcinogen in rats when injected locally at the site of injection. Cadmium metal powder is a carcinogen in rats when injected intramuscularly forming malignant tumours.
Only one study reported an increase in cancer upon oral exposure to soluble cadmium compounds; no data were located for cadmium metal or cadmium oxide.
Toxicity for reproduction
By the oral route, no animal study specifically using cadmium oxide or metal has been identified. The lowest concentration of cadmium reported to affect fertility in rats was 10 mg Cd/kg/day (number of copulations and pregnancies, number of implants and fetuses were decreased) when males and females rats were both treated with Cd water soluble compounds. Inhalation exposure to cadmium oxide at a concentration of 1 mg/m3 (for more than 10 weeks) has been associated with an increase in oestrous cycle length and reduction of the number of spermatids in testis in rats.
The overall NOAEL is 0.1 mg CdO/m³ (about 0.09 mg Cd/m³). No study specifically using cadmium metal by inhalation was located.
Developmental toxicity
No study specifically using cadmium oxide or cadmium metal by the oral route was located.
Neurobehavioural effects or changes in electrophysiological parameters were reported to occur at
doses that did not induce maternal toxicity. Lowest dose reported to generate behavioural changes
in pups is 0.04 mg Cd/kg/day (LOAEL) (Baranski et al., 1983). Significance of these changes and
underlying mechanisms for the observed effects on behavioural endpoints are not completely
elucidated yet; some authors suggested that the toxic effects might be mediated by placental toxicity
or by interference with the normal fœtal metabolism of Zn and/or Cu. Because the oral
bioavailability of CdO and Cd metal is not fundamentally different from the compounds tested, it
can reasonably be considered that these observations can be extended to Cd metal and CdO by the
oral route.
Inhalation route: No study using cadmium metal was located. Some studies using cadmium oxide and investigating foetal body weight, malformations or neurobehavioural effects of inhaled cadmium have been identified (Baranski, 1984; Baranski, 1985; NTP Report 1995). Neurobehavioural changes were reported in young rats from dams exposed to CdO (0.02 mg Cd/m3 or about) in an apparently single experiment but these observations should be confirmed in an independent study.
Read-across Example: Cu & Cu Compounds Human Health
Ilse Schoeters and Violaine Verougstraete
Eurométaux
7 July 2006
Read-across example Cu & Cu compounds human health
Read across has been used under a select set of circumstances in the ongoing Voluntary Copper Risk Assessment. For purposes of testing derogation and acute toxicity classification, read across has been based upon evaluation of physical properties (e.g. particle size in dustiness testing), water solubility and oral absorption. Cu-VRA substances: copper (metal), copper (I)oxide, copper(II)oxide, copper sulphate pentahydrate, copper oxychloride Data available
Compound
Cuprous (I)
Copper (II)
sulphate
oxychloride
Solubility (mg/L)
D50 (µm) MMAD of
marketed material
D50 (µm) and dustiness
of airborne fraction
(mg/g) Oral absorption
Dermal Absorption
Inhalation absorption
Acute inhalation
toxicity (mg/L)
Acute oral toxicity
Acute dermal toxicity
Skin sensitisation
9: data available of good quality 9/-: data available but of limited to poor quality -: no data available
Read-across was applied to derive classification proposals and assess risks for the substances/endpoints shaded in
-for acute inhalation toxicity for Cu(II)O, Cu-powder and CuS04.5H2O
-for acute oral toxicity for Cu-powder
-for mutagenicity
-for carcinogenicity
-for reproductive toxicity
-for repeated dose toxicity
Properties used to read-across from compounds with data to compounds without data or with no good quality data:
-solubility in water
-oral absorption
-dustiness information and particle size distribution
Acute oral toxicity for Cu-powder
Copper powder
Read-across was based on solubility and available acute oral toxicity information for the other
LD50 of the least soluble compound Cu(II)O: >2500 mg/kgBW; no classification.
The more soluble compounds all classify as harmful.
In view of the poor solubility of copper powder, it is considered appropriate to read-across from
Conclusion: copper powder no classified
Acute inhalation toxicity for Cu(II)O, Cu-powder and CuS04.5H2O (still under discussion;
based on proposal prepared by EBRC)
Copper (II) oxide
Read-across was based on the following information:
-Copper (II) oxide has a low oral toxicity (LD50 > 2000 mg/kg bw), and -a low water solubility (< 0.39 mg/L). Although toxicokinetic data in laboratory animals are not
available for this compound, investigations in feeding trials with cattle, pigs and chicken indicate a
very limited bioavailability compared to other copper compounds.
-Laboratory investigations on the propensity to become airborne suggest a moderate mobility (i.e.,
total dustiness measured at 364 mg/g). The particle size distribution of airborne matter shows a
mass median aerodynamic diameter of more than 60 microns. Using the MPPD model, the
deposition pattern in humans (extrathoracic 49%, tracheobronchial 0.9%, alveolar fraction 1.1%)
and rat was derived (total deposition of 34%, with 33% deposited in the extrathoracic region, 0.4 %
in the tracheobronchial region, and 0.4% in the alveolar fraction).
Summary:
- Copper (II) oxide has a moderate (but not negligible) tendency to become airborne,
-based on particle size considerations (MMAD > 60 µm), more than 95% of the material deposited
in the respiratory tract will be translocated to the GI tract shortly after inhalation, so that the acute
toxicity will be determined by that of the oral route,
- Copper (II) oxide is assumed to have a low bioavailability, based on water solubility and on
indicative data from animal feeding studies,
- it has an established low oral toxicity (LD50 > 2000 mg/kg bw).
Conclusion:
the inhalation hazard of copper (II) oxide is very low and it was therefore proposed not to classify
copper (II) oxide for acute inhalation toxicity.
Copper powder
-Copper powder has not been tested for acute oral toxicity, but by way of read-across from data on
copper (II) oxide and its extremely low water solubility (<< 1 mg/L) has been assigned a low oral
toxicity, not requiring classification.
-In analogy with copper (II) oxide, a very limited bioavailability compared to other copper
compounds is similarly assumed.
-Laboratory investigations on the propensity to become airborne suggest a very low mobility (i.e.,
total dustiness measured at 46 mg/g). The particle size distribution of airborne matter shows a mass
median aerodynamic diameter of more than 70 microns, based upon which the following deposition
pattern in humans was derived using the MPPD model: extrathoracic 49%, tracheobronchial 0.9%,
alveolar fraction 1.2%. The corresponding prediction for the rat is 34% total deposition, with 33%
deposited in the extrathoracic region, 0.4 % in the tracheobronchial region, and 0.4% in the alveolar
Summary:
- Copper powder has a negligible tendency to become airborne (i.e., less than 5%),
- based on particle size considerations (MMAD > 70 µm), more than 95% of the material deposited
in the respiratory tract will be translocated to the GI tract shortly after inhalation, so that the acute
toxicity will be determined by that of the oral route,
- Copper powder, in analogy to copper (II) oxide and based on the extremely low water solubility, is
assumed to have a low bioavailability,
- Copper powder is assumed to have a low oral toxicity (LD50 > 2000 mg/kg bw) by read-across from copper (II) oxide.
Conclusion:
the inhalation hazard of copper powder is very low, and it was therefore proposed not to classify
copper powder for acute inhalation toxicity.
Copper sulphate pentahydrate
-Copper sulphate pentahydrate has been tested several times for acute oral toxicity, with resulting
LD50 values in the rage of 480 – 960 mg/kg bw, resulting in a classification as "harmful via ingestion". Similarly, the anhydrous form has yielded an LD50 of 300 mg/kg bw, in line with the slightly elevated relative copper content compared to the hydrate. In contrast, no data is available on
acute inhalation toxicity of copper sulphate pentahydrate.
- Copper sulphate pentahydrate has a low relative copper content (25%, compared for example to
copper powder (100%), copper (I) oxide (89%) and copper (II) oxide (80%)
- the oral bioavailability of copper sulphate in comparison to other copper compounds at dose levels
relevant for acute toxicity testing has been assessed as being similar, i.e. 11-13%
- laboratory investigations on the propensity to become airborne suggest a negligible mobility (i.e.,
total dustiness measured at 49 mg/g). The particle size distribution of airborne matter shows a mass
median aerodynamic diameter of more than 90 microns, based upon which the following deposition
pattern in humans was derived using the MPPD model: extrathoracic 49%, tracheobronchial 1.0%,
alveolar fraction 1.2%. The corresponding inhalation deposition prediction for the rat is 34% total
deposition, with 33% deposited in the extrathoracic region, 0.4 % in the tracheobronchial region,
and 0.4% in the alveolar fraction
- technical considerations render inhalation toxicity testing of copper sulphate pentahydrate
technically unfeasible: the d50 physical particle size has been determined at 220 µm.
- without manipulation of particle size (i.e. by micronisation), more than 95% of inhalable copper
sulphate pentahydrate would be deposited in the extrathoracic region, and subsequently and rapidly
translocated to the GI tract; however, only approx. 5% of the material present in commercial grades
of this substance can at all become airborne
- even if a mechanical micronisation procedure down to particle sizes in the region of those tested
for other copper compounds (i.e., copper (I) oxide and copper oxychloride) would be employed, the
thus generated material would render a similar deposition pattern in the respiratory tract, again with
predominant translocation to the GI tract; however, based on the rel. copper content of only 25%,
the inhalation of particles of the same size range as those already tested would deliver only one
third to one quarter of the amount of copper by comparison
- given that copper (I) oxide and copper oxychloride have been tested in multiple assays, and have
yielded "borderline" results (i.e, some LC50 value above 5 mg/L, some below), then it can not be expected that copper sulphate pentahydrate can cause any toxicity beyond that observed for these
two compounds; instead, less copper is expected to become systemically available
- toxicity other than systemically available copper cations is not to be expected: any copper sulphate
will deposit almost exclusively in the ET fraction, from where systemic absorption is unlikely to
occur; absorption from this region is commonly extrapolated from dermal absorption data, which is
known to be low (0.3%).
- given that copper sulphate is only moderately irritant and not corrosive, any direct local action is
also not anticipated
- the residual very low amounts of copper sulphate that may reach the tracheobronchial based on the
predictions set forth below may at first glance be subject to dissolution; however, any dissolution is
effectively counteracted by the high carbonate content (50 mM) of airway fluids, so that any
material that becomes available would be rendered insoluble as copper carbonate
Conclusion:
the inhalation hazard of copper sulphate pentahydrate is very low; and it was therefore proposed not
to classify copper sulphate pentahydrate for acute inhalation toxicity
Mutagenicity, Carcinogenicity, Reproductive toxicity, RDT
Toxicity data are available for CuSO4.5H2O and no data is available for the other compounds.
Classification proposals and risk analysis for the non tested compounds are based on the
conclusions for CuSO4.5H2O assuming that copper in the GIT will occur, at least in part, in the
ionic form and will therefore be available for absorption. Whilst bioavailability data exist for
several copper substances in addition to copper sulphate (copper (I) oxide, copper (II) oxide and
copper oxychloride), the database is limited in terms of quality and/or relevance to human risk
assessment. Further, no bioavailability data are currently available for copper powder. In the
absence of reliable bioavailability data for all copper substances, as a worst case situation, it was
considered appropriate to read-across from copper sulphate, on the basis that this is the most soluble
and apparently bioavailable copper substance covered here, and the most well-characterised with
respect to toxicokinetics and repeated dose toxicity.
Read-across Example: Ni & Ni Compounds
Ilse Schoeters and Violaine Verougstraete
Eurométaux
7 July 2006
READ-ACROSS: Example Ni and Ni-compounds
Basis: Data collected for the EU-RA and EU classification and labeling of Ni and
The document provides principles on how to apply read-across and gives examples for certain
endpoints.
I. Background: In its simplest form, read-across is an extrapolation of known data from one
substance to another substance on the basis of assumptions leading to a conclusion that the two
substances will cause similar biological responses. This basic premise of science is used in the
regulatory setting to reduce the need for animal testing. The most detailed embodiment of the
concept of read-across has occurred in the production of Qualitative Structure Activity Relationship
(QSAR) models, developed for organic compounds and based on the presence of active groups
within the organic molecule that are capable of eliciting certain biological effects. For metal
substances, it is very important to understand that the simple presence of a metal in a substance does
not necessarily impart to that substance the biological properties of the metal ion. It is the
bioavailability of the metal ion (or a redox form of this ion) at target sites that needs to be assessed
for the read-across of metal substances to be accurate.
In general, regulatory read-across seeks to extrapolate the biological response data for required
toxicity testing endpoints. These endpoints can be summarized as follows:
• Acute Toxicity – Inhalation, Oral, Dermal • Irritation – Eye, Dermal • Sensitization – Skin, Respiratory • Repeated Dose Toxicity – Inhalation, Oral, Dermal • Mutagenicity • Carcinogenicity – Inhalation, Oral, Dermal • Reproductive – Developmental Toxicity, Gonadal Toxicity (Fertility)
For the purposes of regulatory read-across, toxicity categories can be defined that will aid in reducing testing requirements for a group of similar substances. Specifically, for inorganic substances and many organic substances four categories can be developed:
1. Systemic threshold toxicity 2. Systemic non-threshold toxicity 3. Contact threshold toxicity 4. Contact non-threshold toxicity
The major difference between the contact and systemic toxicity categories lies in the manner in
which the substance is made bioavailable to the target tissue.
• In the case of dermal, eye, or inhalation toxicity, bioavailability is dictated by a series of
local events that will enable the metal ion in the substance to interact with target cells and organelles in the tissue of contact. These events could include phagocytosis, passive and active membrane transport, and redox changes.
• In the case of systemic toxicity, all of these local events are equally important. However,
prior to consideration of whether a substance will have similar biological activity at a systemic site of action, one needs to consider whether the substance will be absorbed from the respiratory tract by inhalation, from the skin, or from the gastrointestinal tract after oral exposure and transported to the site of action. Therefore, in the case of systemic toxicity,
oral exposure nearly always results in the highest systemic exposure of a substance and hence may often be the route of exposure used to determine if the criteria for grouping and extrapolation is appropriate.
The same issues hold for systemic and contact non-threshold toxicities (e.g., most commonly
mutagenicity and carcinogenicity) with the exception that there is no safe level of exposure
associated with zero risk of causing the toxicity in question.
While most of the issues surrounding the concept of bioavailability at target sites is self evident, it is
still essential to validate the grouping criteria, by demonstrating an equivalency of bioavailability
between the substances of a proposed grouping. Doing so constitutes the validation of the grouping
criteria stipulated by the OECD and TAPIR Annex 9 as essential components of the read-across
process. In fact, there are a number of common validation principles across all of these approaches
that need to be addressed to a greater or lesser extent in order for the output to be accepted (TAPIR
Annex 9). These include:
• transparency - both of method and data; • definition of applicability domain. This domain refers to those chemicals that the approach
can make reliable predictions for;
• assumption, or recognition of a common mode of action or mechanistic understanding; • validation of model/output.
II. Extrapolating Threshold Based Toxicities
For regulatory purposes, threshold based toxicities include all the endpoints mentioned in section
I, except mutagenicity and carcinogenicity (and in some cases respiratory sensitization).
• In the case of most systemic threshold-based toxicities, oral exposure provides the highest
potential for systemic exposure levels.
• In the case of threshold-based contact toxicities dermal, eye, and inhalation routes of
exposure must be evaluated.
In this framework, a scientifically valid grouping and read-across (from a "reference substance" to the "substance under evaluation") for threshold based systemic toxicities is possible.
1. No read across is needed
There is data on systemic effects seen with that specific substance under evaluation.
2. Best read across
- Obtain toxicokinetics data on NOAEL blood levels of a reference substance from the group with a known toxicity profile - Compare those levels to the blood levels of the substance under evaluation exposed at its Maximum Tolerated Dose (MTD).
If the blood levels of the substance under evaluation are below those corresponding
to the NOAEL value for the reference substance, do not classify.
If values are higher, classify and use the NOAEL of the reference substance for risk
characterization.
3. Limited read across No toxicokinetic data are available.
- Collect data on oral absorption and oral toxicity (MTD) for the reference and the substance
under evaluation. The balance between oral toxicity and absorption will determine what is the highest absorbed level possible for the substance under evaluation. - Compare these data. - Based on expert judgment, decide whether to classify or not.
4. Minimal read across No data on toxicokinetics or oral absorption are available.
- Generate data on bioaccessibility (dissolution of the substance under evaluation in biologically relevant solutions (e.g., lung lavage fluid, gastric juice/intestinal juice, synthetic sweat). - Conduct side by side experiments with the substance under evaluation and if possible with more than one reference substance(s). These assays can be used to generate quick and relatively inexpensive data on a large number of substances. However, this approach needs to be validated by using several reference substances for which some correlation between bioaccessibility in gastric fluid and oral absorption exists.
5. Not acceptable read across - No data on toxicokinetics, oral absorption, or bioaccessibility
are available. Only physical/chemical data exist. - This can ONLY be used for read across if the physical/chemical nature of the substance under evaluation is so similar to that of the reference substance, that bioaccessibility and oral absorption are predicted to be almost identical.
III. Test waiving
If for a given substance under evaluation, no data are available for steps 1-4, and the producer does
not want to undertake the minimal testing (step 4) or more thorough testing (step 2-3), then the
default classification should follow the worst case substance in the proposed grouping category.
Such a default classification would represent therefore the most conservative option for default
classification.
IV. EXAMPLE: REPRODUCTIVE EFFECTS AND NICKEL SUBSTANCES: CLASSIFICATION AND RISK
CHARACTERIZATION.
Data available for classification and risk characterization.
Data were available from one- and two-generation reproductive studies in rats exposed orally to
nickel chloride and nickel sulphate studies. The NOAEL for developmental effects seen in rats
exposed orally to nickel sulphate equates to 1.1 mg Ni/kg/day. Based on these data, nickel sulphate
and chloride were classified as category 2 developmental toxicants.
How to "read across" to other nickel substances?
The determinant of a developmental reproductive effect is the bioavailability of the nickel ion to
systemic circulation and ultimately the delivery to the conceptus of pregnant rats. Bioavailability of
nickel ion will be influenced by oral absorption.
Read across from nickel sulphate and chloride data to another nickel substance.
1. No read across is needed – There is data on reproductive effects seen with that specific
nickel substance. Examples: nickel chloride hexahydrate and nickel sulphate hexahydrate.
2. Best read across
- Obtain toxicokinetics data on blood nickel levels after oral exposure to 1.1. mg Ni/kg of
nickel sulphate hexahydrate (reference substance). - Measure the same parameters for the other nickel substance under evaluation at the MTD and compare values.
If the blood levels of the nickel substance under evaluation are below those
corresponding to the NOAEL value for nickel sulphate ∈ do not classify.
If values are higher ∈ classify and use 1.1. mg Ni/kg for risk characterization.
3. Limited read across – No toxicokinetic data are available.
- Collect data on oral absorption of the nickel substance under evaluation as well as oral toxicity - Compare to the values for the reference substance (nickel sulphate hexahydrate). Example: read across by the TC C&L for nickel metal as regards to reproductive toxicity, given the absence of toxicokinetic data. Nickel metal had 100-fold lower oral absorption than nickel sulphate, while the oral LD50 was over 30-fold higher. The high equivalent oral exposure levels in humans that would be needed to absorb sufficient nickel ion to cause an adverse effect were not considered to be realistic. Expert judgment deemed that nickel metal should not be classified as a reproductive toxicant.
4. Minimal read across – This approach was not used for nickel compounds. However,
validation of such an approach could be done by: - looking at the oral absorption for some substances that have bioaccessibility values in the middle of the range between sulphate (category 2) and metal (no classification) and - making sure that the bioaccessibility value corresponding to the threshold between classification and no classification is well defined (Minimal validation could include comparison of oral absorption and bioaccessibility)
5. Not acceptable read across – No data on toxicokinetics, oral absorption, or bioaccessibility
are available. Only data on water solubility exist. This can ONLY be used for read across if the water solubility and nature of the nickel substances is so similar to that of the reference substance, that bioaccessibility and oral absorption are predicted to be almost identical. For example, read across from nickel sulphate to nickel chloride and nitrate. Both are strong salts that will be expected to be ionized and dissolved completely in the gastric environment.
V. Extrapolating Non-Threshold Based Toxicities
For regulatory purposes, non-threshold based toxicities typically refer to mutagenicity and
carcinogenicity. As in the case of systemic threshold based toxicities, oral exposure provides the
highest potential for systemic exposure levels. In the particular case of non-threshold based contact
toxicities, the inhalation route of exposure must be evaluated and expert judgment should be applied
to the need for testing the dermal and eye routes of exposure (i.e., these would be expected to be
done infrequently).
VI. How to read across for carcinogenicity?
The most important issue to be able to read across for carcinogenicity classification and risk
characterization is to understand the mode of action of the carcinogenic substance. Some important
components of mode of action are:
1. local or systemic nature of the cancer effects, 2. mutagenic (genotoxic) or non genotoxic mechanisms for cancer induction, 3. factors that influence bioavailability of metal ion at critical cellular sites.
Complete information on mode of action is not likely to be available for any metal substance and, in fact, for few compounds at all. Therefore, this is one of the most difficult endpoints to consider read across for. Some examples of differences in components of the mode of action for cancer associated with metal/metalloid substances are as follows:
• systemic effects for arsenic via inhalation but only local (respiratory) effects for nickel
• difficulty to draw a universal relation between water solubility and bioavailability
o water solubility of substances is directly related to bioavailability of cadmium (II)
(for tumour induction) from cadmium compounds,
o water solubility is inversely related to bioavailability of Cr (VI) from chromium
compounds (for tumor induction) after inhalation.
o The relationship between water solubility and bioavailability of nickel (II) at target
sites may be even more complex for nickel, with compounds of intermediate solubility having the highest bioavailability.
• Both mutagenic and non genotoxic effects have been postulated to be part of mode of action
In the process of assessing weight of evidence for carcinogenicity classification, the following approach is typically used:
• causal association in human epidemiological studies indicates a category 1 carcinogen
• causal association in animal studies (with no data or weak evidence from human studies)
indicates a Category 2 carcinogen classification
• weak data from animal studies (e.g., positive data by non relevant routes or in a poor study)
and/or human studies, with some supporting evidence of mutagenicity indicates a Category 3 carcinogen classification
In reading across from one substance classified as a carcinogen to another, the relevance of the read across data has to be considered as well as the starting Category classification of the reference substance. A possible approach is shown below with examples from the read across for nickel substances.
VII. EXAMPLE: CARCINOGENICITY EFFECTS AND NICKEL SUBSTANCES: CLASSIFICATION AND RISK
CHARACTERIZATION.
Nickel data availability for classification and risk characterization.
Data were available from several epidemiological studies of nickel refinery workers exposed to
mixtures of nickel-containing substances and other confounders. Data were also available from
three well-conducted cancer inhalation studies in rats with three different nickel compounds
1. Based solely on the epidemiologic data, the EU Specialized Experts recommended nickel
sulphate and chloride be classified as Category 1 carcinogens.
Read across for a Category 1 carcinogen
Presumably, the reference substance has a causal association in epidemiologic studies and no data
or positive animal data in animal studies exist. The epidemiological studies should be strong
enough to indicate that the reference substance being assessed2 is responsible for excess cancer
risks. For this substance, there may be quite a bit of information on the components of the mode of
action, particularly if animal studies have been undertaken3.
To read across from the reference substance to another substance under evaluation, a weight of
evidence approach considering the various components of the mode of action could be undertaken.
- Evaluate data on any existing epidemiologic studies that included the substance under
- Consider any animal evidence for carcinogenicity of the substance under evaluation, - Assess presence of systemic versus local effects for substance under evaluation, - Get data on factors that influence bioavailability at target cellular sites for cancer. - If weight of evidence is strong of similar responses for reference and substance under
Consider read across with a category 1 carcinogen classification.
[For nickel substances, this could be exemplified by reading across from nickel sulphate and chloride to nickel nitrate (category 1 carcinogen), or for reading across from crystalline nickel subsulfide to nickel sulfide (category 1 carcinogen).]
1 E.g., nickel sulphate hexahydrate, nickel monoxide calcined at high temperatures, and crystalline nickel subsulfide 2 And not a mixture 3 Note that this scenario did not exactly apply to nickel sulphate or nickel chloride since there were no epidemiological studies with single exposures to these substances and the animal cancer inhalation study with nickel sulfate was negative.
Step 2. • Data on epidemiological studies does not exist for other substance or is very weak, but some
animal data are available, or
• no human or animal data are available for substance under evaluation but data on mode of
action is robust to indicate same effects as reference substance.
In this scenario, because there is a justifiable concern for the reference substance, but not too much supporting data for the other substance:
A read across to a lesser carcinogen classification (category 2 or 3) may be
appropriate.
[For nickel substances, one example could be the read across from nickel monoxide to complex nickel-copper oxide. There are not enough data for the classification of nickel copper oxide as a category 1 carcinogen in its own but there are some animal injection studies that could provide supporting evidence for a category 2 carcinogen. Note. The EU TC C&L recommended a category 2 read across for many nickel containing substances in the absence of any supporting data, except water solubility.
Step 3. No cancer/tumor data available for other substance, but some data on components of mode of action exist that suggest similarities (e.g., data from bioaccessibility studies or even water solubility studies). In this scenario:
Read across to a lesser carcinogen classification (category 3) may be appropriate4.
Read across from a Category 2 or 3 carcinogen.
In the case of substances classified as Category 2 and 3 carcinogens, the weight of evidence for
carcinogenicity of the reference substance is weaker. The requirements for data needed to read
across to the same carcinogenicity classification should be the same as mentioned above in steps 2
and 3. Otherwise, there is a risk of perpetuating the uncertainties, by directly reading across from a
substance that has weak data to another with even less certain data.
Example, nickel metal has a category 3 carcinogen classification at the moment based on a couple
of injection animal studies. To read across a Category 3 carcinogen classification from nickel metal
to a NiTi alloy would be inappropriate unless animal supporting data would be available. Consistent
with this idea, the EU TC CL & L did not read across from nickel metal to nickel alloys.
Test waiving
If for a given metal substance under evaluation, no data are available for steps 1-3. REACH may
require the producer to undertake the minimal testing requirement (step 2-3) but the producer may
potentially waive more thorough testing (step 1). In such a case the classification should be based
on a realistic scenario using the minimal information available (step 2-3). While it is recognised that
such cancer classification may be more conservative than when all test info would be available, it is
4 a Category 3 may have been more appropriate read across based on existing data for hundreds of nickel substances, but instead the EU CL&L recommended a category 2 read across in the absence of any supporting data, except water solubility
not necessarily equal to a default worst case classification. Indeed the minimal testing requirement forms here the basis on which the decision based on the most plausible assumption should be made. Example: For the case of nickel substances, read across from reference substances could result in category 2 or 3 along the criteria stated above. The default classification in the absence of read across data would be category 1 as applied to nickel carbonate hydroxide through a test derogation document. It should be made clear in this case that the classification given to nickel hydroxy carbonate is the result of test waiving derogation and therefore the weight of evidence implied in a Category 1 classification was not fulfilled in this case.
Read-across Example: Pb & Pb Compounds
Ilse Schoeters and Violaine Verougstraete
Eurométaux
7 July 2006
Acute Toxicity
Read across has been used under a select set of circumstances in the ongoing Voluntary Lead Risk
Assessment. For purposes of testing derogation and acute toxicity classification, read across has
been based upon evaluation of physical properties (e.g. particle size in dustiness testing) and water
solubility. This strategic approach has been made possible by a set of circumstances that include:
- all compounds under evaluation were sparingly soluble in water - acute oral toxicity data were available for a number of compounds under evaluation and
indicated absence of toxicity up to the upper limit of ranges tested
- direct effects upon the skin or the lung (e.g. sensitization) were absent - the particle size distribution of compounds was such that upper airway deposition would be
predicted, to be followed by translocation to the gastrointestinal tract.- systemic effects following acute inhalation exposure would thus largely be dictated by the oral exposure route
3ΥΡΣΗΥΩΛΗς ∆ΘΓ ∆ΦΞΩΗ ΡΥ∆Ο ΩΡ[ΛΦΛΩ∴ ΟΗ∆Γ ∆ΘΓ ΟΗ∆Γ ΦΡΠΣΡΞΘΓς
Dustiness
D50 [µm]
Solubility
Rat Oral LD50
D50 [µm]
Substance
(mg/kg/bw)
diameter(1)
Under this set of circumstances, read across was facilitated by a lack of acute effects for multiple compounds for either systemic toxicity resulting from ingestion routes of exposure or direct contact
toxicity. Water solubility was presumed to be an adequate, albeit potentially imperfect, indicator of compound dissolution on the skin (in sweat) or in the mucous of the pulmonary tract that suggested there was no reason to believe that compound specific differences existed that would modify this lack of toxicity. Read across for acute oral toxicity was made possible by the absence of acute oral systemic effects – even though it was known that the oral bioavailability of the compounds being tested was in fact quite different and not reflected by differences in water solubility. The existing scientific literature has documented that the oral bioavailability of lead compounds varies by several orders of magnitude and as a function of the behavior of a given lead salt in the acidic condition of the gastrointestinal tract. The following table presents the conclusions regarding acute toxicity based upon read across following these principles. Measure values or conclusions are indicated in bold faced type. Conclusions based upon read across in accordance with the preceding principles are in italics.
3ΥΡΣΗΥΩΛΗς ∆ΘΓ ∆ΦΞΩΗ ΡΥ∆Ο ΩΡ[ΛΦΛΩ∴ ΟΗ∆Γ ∆ΘΓ ΟΗ∆Γ ΦΡΠΣΡΞΘΓς
Rat Oral LD50
Inhalation
Dermal LD50
Skin or lung
Substance
(mg/kg/bw)
(mg/kg/bw)
> 2000
>2000
> 2000
>10,000
> 2000
> 2000
> 2000
> 2000
> 5000
> 10,000
None
> 2000
> 2000
> 2000
> 2000
> 2000
> 5000
> 2000
phosphite / sulphite
> 2000
> 2000
> 2000
> 2000
>2000
Repeated Dose Toxicity
Most toxicity studies of lead compounds in experimental animals have employed extremely soluble
compounds such as lead acetate. Toxicity has further been observed in studies of exposed human
populations, but the external dosimetry (administered dose) required to produce effects in humans is
usually unknown. However, determination of lead levels in blood has proven to be a reliable index
of systemic exposure and can serve as the basis for determining whether or not a level of exposure
sufficient to produce systemic toxicity has been attained.
Prediction of the toxic potential of different lead compounds based solely upon physical or chemical
properties of individual lead compounds has proven difficult due to discrepancies between
parameters such as water solubility and the relative bioavailability of different lead compounds
following the more common routes of exposure (e.g. ingestion). The following table presents the
aqueous solubility of a number of lead compounds and contrasts their typical relative bioavailability
to that of lead acetate, one of the most soluble and bioavailable compounds known. While
extremely water insoluble lead compounds can have low bioavailability, some can exhibit
bioavailability that approaches that exhibited by lead acetate. The failure of the water solubility of
compounds to correlate with relative bioavailability is due to the complex acidic conditions within
the stomach and variability of bioavailability of as a function of decreasing particle size. As
particle size decreases, the resistance of otherwise stable lead compounds in the gastrointestinal
tract will be diminished. Relatively oral bioavailability will further me modulated by "matrix"
effects that can be exerted by lead-containing foods or soils that may be ingested.
Comparison of water solubility and bioavailability (oral) relative
to that of lead acetate (in %)
Compound
Water Solubility (mg/L)
% Bioavailability
(relative to lead acetate)
Lead Metal Powder
The relative bioavailability of different lead compounds can be determined by animal feeding
studies. In vitro test systems have also been validated that can predict the bioavailability of lead
contained in matrices such as soil but cannot yet be applied to predict the bioavailability of pure
compounds.
The costs of conducting animal feeding studies, and the multitude of modifying factors that can
alter bioavailability, are such that estimates of the presence or absence of risk of repeated dose
toxicity is generally assessed from studies of exposed human populations and the level of lead that
is observed in blood. If the level of lead in blood is above that associated with systemic toxicity,
then risk of repeated dose toxicity is presumed. If it is lower, the lack of risk is assumed.
CMR Endpoints
Evaluation of CMR endpoints, and read across issues associated with them, has proven to be
challenging within the context of the existing voluntary lead risk assessment.
Highly soluble lead compounds have been shown to be mutagenic and genotoxic in vitro, but responses observed are generally weak and appear to be mediated by indirect mechanisms (e.g. inhibition of DNA repair, alteration of oxygen radical metabolism), presumably through the action of the lead cation. Concentrations required to elicit these effects are usually high (mM concentration range) and beyond the solubility of most lead compounds being evaluated to for risk assessment purposes. On the basis that mechanism(s) of mutagenic action are presumed to be mediated by the lead cation, it has yet to be determined if insoluble compounds can realistically be expected to exhibit activity in vitro. Testing in vivo has similarly been conducted almost exclusively with highly water soluble compounds, yielding weak and inconsistent results that have been both difficult to interpret and to extrapolate to insoluble compounds.
Soluble lead compounds produce kidney cancer in rodents when administered at high doses and, as with mutagenicity, appears to be mediated by indirect mechanisms. Testing of insoluble compounds (oral gavage studies with lead metal powder, inhalation studies with lead oxide) have produced negative results. It thus remains to be determined how high dose effects obtained in animals through the administration of soluble/bioavailable compounds can be extrapolated to insoluble or nonbioavailable compounds. Extrapolation between compounds based upon the blood lead levels that can be produced is being attempted, but is complicated by a lack of blood lead measurements in most cancer bioassays.
Reproductive Toxicity
Lead compounds are known human and animal reproductive toxicants, most notably inducing developmental deficits at relatively low levels of exposure. While it is conceivable that some lead compounds may have bioavailability that is sufficiently limited so as to restrict their reproductive toxicity, the doses required to produce developmental effects are sufficiently low to support a presumption that most, if not all, lead compounds should be classified as known human developmental toxicants.
Grouping of Petroleum Substances
Mike Comber & Barry Simpson
September 2006
Grouping of Petroleum Substances
The purpose of this paper is to describe the historical approach for the grouping of petroleum
substances which has been applied in the context of the Existing Substances Regulation 793/93 and
for classification under the Dangerous Substances Directive 67/548.
Background
There was a need to arrange approximately 660 petroleum substances listed on EINECS, into
smaller groups to assist in the collection, summary and evaluation of hazard data for the purpose of
satisfying the requirements of the Existing Substances Regulation (EU Council regulation 793/93 of
23 March 1993, published in the Official Journal L84 on 5 April 1993). The grouping approach
developed for that purpose was also used by the EC C&L working group in their assessment for
classification for carcinogenicity of petroleum substances (21st adaptation to technical progress of
the Dangerous Substances Directive, published in the Official Journal L 381 on 31.12.1994). In
addition, the oil industry association CONCAWE has employed this grouping approach in the
voluntary risk assessment of gasoline, which has been presented to and discussed by TCNES at its
meeting in December 2004, and in the drafting of industry guidance on the classification and
labelling of petroleum substances.
Petroleum substances are of unknown and variable composition and are regarded as typical UVCBs
(substances of Unknown or Variable compositions, Complex reaction products or Biological
materials). They can therefore only be described in general terms and hence the assigned CAS
numbers do not describe them precisely in terms of their exact chemical composition. However, the
CAS definitions for petroleum substances identify the starting material, the last processing step that
the substance underwent during its manufacture and, in many cases, an indication of key physico-
chemical properties such as boiling range, and carbon number range.
For these reasons a different approach was required for grouping the petroleum substances
compared to the classical grouping approach used for well defined chemical substances.
Rationale and grouping of petroleum substances
Since it was not possible to characterise the petroleum substances in terms of their exact chemical
composition it was decided to group them according to the process by which they are being
manufactured. It was reasoned that similar starting materials that underwent similar modification
and/or separation processes would result in streams of broadly similar composition.
The resulting groups and sub-groups of petroleum substances were published in the Official Journal
L84 on 5 April 1993. Descriptions for each of the groups or sub-groups are described in Table 1.
Use of the grouping methodology
The
determination/prediction of their intrinsic hazard properties through application of a variety of techniques, such as use of test data, read-across, QSAR modelling, and marker substances (i.e., 1,3-butadiene, benzene, polycyclic aromatics). It should be noted that when read-across is applied to a group/sub-group, all substances included in the groups/sub-groups are presumed to exhibit similar intrinsic hazard properties based on similarities in their physico-chemical properties. This grouping methodology was also developed with the desire to avoid further animal testing and was confirmed by the Commission in the Official Journal L84 on 5 April 1993. As illustrated by the following example, a combination of the use of hazard data, read-across and markers was applied for the classification of petroleum substances for carcinogenicity. It should be
noted that markers have been developed to facilitate the classification for carcinogenicity;
classifications for other endpoints are largely based on read-across from data on related petroleum
substances.
Example of the approach used for the classification for carcinogenicity
For some of the individual substances in the petroleum substance groups/sub-groups listed in Table
1, results of carcinogenicity studies were available. However, it was also recognized that some
refining processes to which the distillation fractions of crude oil were subjected could result in the
occurrence of varying levels of individual constituents, which themselves were known to be
carcinogenic. Therefore, it was decided to use a combination of available carcinogenicity data and
knowledge of the occurrence of known carcinogenic substances in the streams during the
assessment of classification for carcinogenicity.
The carcinogens that were known to occur in petroleum substances and the concentrations used as
indicators for classification were 1,3-butadiene (0.1%), benzene (0.1%) and IP-346 DMSO
extractables (3%); the latter is a surrogate for polycyclic aromatic hydrocarbons (PAHs), which
was correlated with results from experimental studies. Since the groups of petroleum substances
originate from different processes, this determined which carcinogenic constituents were likely to
be present in substances from the respective groups.
The following information was used by the EU C&L working group to assess the carcinogenicity
classification of each of the following groups of petroleum substances. The classifications represent
the harmonised EU Classifications which appear in Annex 1 to the Dangerous Substances Directive
(67/548 EC). They are examples illustrating how the techniques were applied:
Crude Oil (group 1) contains a number of carcinogens, notably benzene and 4 to 6 fused ring
polycyclic aromatic hydrocarbons (PAHs).
Classified as a Category 2 carcinogen on the basis of available animal studies.
Petroleum Gases (group 2) derive from crude oil distillation and cracking processes. The latter can
give rise to the presence of 1, 3-butadiene. For those gases which are condensed to give LPG, either
butadiene extraction or hydrofinishing is normally practiced reducing the concentration of
butadiene to negligible levels.
All Group 2 substances are classified as Category 1 carcinogens unless the concentration of 1,3-
butadiene is <0.1%.
Gasoline Streams (groups 3A to 3G) consist of petroleum substances composed of aliphatic and
aromatic hydrocarbons including benzene.
All Group 3A to 3G substances are classified as Category 2 carcinogens unless the concentration of
benzene is <0.1%.
Kerosine Streams (groups 3H to 3J) all contain promoters. Do not contain significant amounts of
benzene or PAHs
On the basis of animal studies, Group 3H to 3J substances are not classified for carcinogenicity.
Gas Oil Streams (groups 4 and 5) all contain promoters. Straight run gas oils (group 4A) do not
contain significant concentrations of PAHs. Cracked gas oils (group 4B) contain PAHs. Other gas
oils (group 5B), with the exception of distillate fuels – see below, are assumed to be carcinogenic
unless information is available to contrary on the refining history.
On the basis of animal studies, Group 4A substances are not classified for carcinogenicity.
On the basis of animal studies, Group 4B and 5B substances are classified as Category 2
carcinogens.
Based on available information the EU C&L working group classified four distillate fuel oils (fuels
diesel, fuel oil number 2, and fuel oil number 4 and fuels diesel number 2) as Category 3
carcinogens.
Heavy Fuel Oil Streams (group 6A) consist of heavy distillates or residual fractions from
distillation or cracking processes and contain saturated, aromatic and olefinic hydrocarbons. Some
of these substances contain significant concentrations of PAHs.
All Group 6A substances are classified as Category 2 carcinogens on the basis of animal studies.
Lubricating Greases (group 6B) consist of lubricant base oils and thickeners. The PAH content of
greases is dependent on the nature and severity of the treatment processes to which the base oils
have been subjected.
Classification of Group 6B substances is dependent on the classification of the base oil.
Lubricant Base Oils (groups 7A to 7C) consist of vacuum distillates and oils obtained from
vacuum residues. Their PAH content is dependent on the nature and severity of the treatment
process to which they have been subjected.
On the basis of human data, all Group 7A substances are classified as Category 1 carcinogens.
On the basis of animal studies, all Group 7B substances are not classified for carcinogenicity.
All Group 7C substances are classified as Category 2 carcinogens unless the IP-346 DMSO
extractables (surrogate for PAH levels) are < 3%.
Lessons Learned
The classical OECD guidance for the formation of chemical categories is appropriate for the
application on chemicals with well-defined chemical structure. As discussed it is not considered
suitable however for addressing categories of UVCB substances, which by their very definition are
of unknown or varying composition. The grouping approach developed by CONCAWE, based on
similarities in refinery manufacturing process and physico-chemical properties, provides a
pragmatic solution, which has been applied to the evaluation of toxicological, ecotoxicological and
physico-chemical hazards of petroleum substances, whilst minimizing unnecessary animal testing.
Table 1
Description of groups of petroleum substances
Name of the group (or subgroup)
sub-group) number* 1
Raw petroleum oil obtained in its natural state from the ground (excluding hydrocarbons from shale and coal) and containing aliphatic, alicyclic, and aromatic hydrocarbons, with small quantities of nitrogen, oxygen and sulphur compounds.
Streams obtained from crude oil distillation, cracking
processes and tail gases, containing saturated and/or olefinic hydrocarbons mainly in the range C2 to C5
Low boiling point
Streams obtained from the atmospheric distillation of
crude oil and containing saturated and aromatic
hydrocarbons, mainly in the range C4 to C12 and boiling in the range ca. -20 to 230°C.
Low boiling point
Streams obtained by alkylation (catalytic reaction),
modified naphtha
isomerisation (catalytic conversion) and solvent
extraction, and containing saturated hydrocarbons, mainly in the range C5 to C12 and boiling in the range ca. 35 to 230°C.
Low boiling point cat-
Streams obtained from the catalytic cracking of heavy
distillates into lighter fractions, and containing saturated,
olefinic and aromatic hydrocarbons, mainly in the range C4 to C12 and boiling in the range ca. -20 to 230°C.
Low boiling point cat-
Streams obtained from the catalytic reforming of mainly
reformed naphtha
n-alkane and cycloparaffinic feedstocks into aromatic
and branched chain hydrocarbons, mainly in the range C5 to C12 and boiling in the range ca. 35 to 230°C.
Low boiling point
Streams obtained by the high temperature splitting of
thermally cracked
heavy distillates into lighter fractions, and containing
saturated, olefinic and aromatic hydrocarbons, mainly in the range C4 to C12 and boiling in the range ca. -20 to 230°C.
Low boiling point
Streams obtained by the catalytic reaction of feedstocks
hydrogen treated naphtha
with hydrogen to remove unsaturated and organic
sulphur compounds, and containing mainly saturated hydrocarbons, mainly in the range C4 to C12 and boiling in the range ca. -20 to 230°C
Low boiling point
Streams obtained by processes such as steam and hydro
naphtha – unspecified
cracking and sweetening, and containing saturated,
aromatic and olefinic hydrocarbons, mainly in the range C4 to C12 and boiling in the range ca -20 to 230°C.
Straight run kerosine
Streams obtained from the atmospheric distillation of
crude oil, and containing saturated and aromatic hydrocarbons, mainly in the range C9 to C16 and boiling in the ca. range 145 to 300°C.
Cracked kerosine
Streams obtained from processes involving the cracking
of hydrocarbon feedstocks, and containing saturated, olefinic and aromatic hydrocarbons, mainly in the range C8 to C16 and boiling in the ca. range 90 to 290°C.
Kerosine – unspecified
Streams obtained from processes not sufficiently defined
to enable them to be placed in sub-groups 3I or 3J and containing saturated, aromatic and olefinic hydrocarbons, mainly in the range C7 to C16 and boiling in the range ca. 90 to 290°C.
Straight run gas oil
Streams obtained from the atmospheric distillation of
crude oil, and containing saturated and aromatic hydrocarbons, mainly in the range C9 to C25 and boiling in the range ca. 150 to 400°C.
Streams obtained from processes involving the cracking
of hydrocarbon feedstocks, and containing saturated, olefinic and aromatic hydrocarbons, mainly in the range C9 to C25 and boiling in the range ca. 150 to 400°C.
Streams obtained from the vacuum distillation of
atmospheric residues, and containing saturated and aromatic hydrocarbons, mainly in the range C11 to C25 and boiling in the range ca. 200 to 450°C.
Gas oil – unspecified
Streams obtained from processes not sufficiently defined to enable them to be placed in groups 4A, 4B or 5A and containing saturated, aromatic and olefinic hydrocarbons mainly in the range C9 to C25 and boiling in the range ca.
Name of the group (or subgroup)
sub-group) number*
Streams obtained as either distillates or residues from
distillation and cracking processes, and containing saturated, aromatic and olefinic hydrocarbons mainly in the range C9 to C50 and boiling in the range ca. 160 to 600°C.
A complex combination of hydrocarbons mainly in the
range C12 to C50 and containing organic compounds of alkali metals, alkaline earth metals and/or aluminium.
Unrefined or mildly
Untreated and acid treated streams obtained from the
refined base oil
vacuum distillation of atmospheric residues, and containing saturated and aromatic hydrocarbons, mainly in the range C15 to C50.
Highly refined base oil
Streams obtained by (a) severe refining of vacuum
distillates to remove aromatic hydrocarbons or (b) the treatment of vacuum residues, and containing saturated and aromatic hydrocarbons, mainly in the range C12 to C50.
Base oil – unspecified
Streams obtained from vacuum distillates, vacuum
residues and atmospheric distillation residues by
processes such as solvent extraction or hydrogenation, and containing saturated and aromatic hydrocarbons, mainly in the range C10 to C50.
Residual aromatic extract
Streams obtained from the solvent extraction of vacuum
residues, and containing saturated and aromatic
hydrocarbons, mainly in the range > C25.
Distillate aromatic
Streams obtained from the solvent extraction of vacuum
distillates, and containing mainly aromatic
hydrocarbons, mainly in the range C15 to C50.
Distillate aromatic
Streams obtained by subjecting untreated aromatic
extract (treated)
extracts from vacuum distillates to processes such as
hydrogenation, and containing predominantly saturated and aromatic hydrocarbons, mainly in the range C
Aromatic extract –
Stream obtained by the solvent extraction of straight run
gas oils, vacuum gas oils and distillation residues etc.,
and containing saturated and aromatic hydrocarbons, mainly in the range C9 to C30 and boiling in the range ca. 150 to 450°C.
Streams obtained as the insoluble phase from the solvent
treatment of atmospheric and vacuum distillates or
vacuum residues, and containing saturated straight and branched chain hydrocarbons, mainly in the range C20 to C50.
Streams obtained as the liquid phase in the separation of paraffin wax from slack wax, and containing mainly branched chain saturated hydrocarbons, mainly in the range C20 to C50.
Streams obtained by the solvent dewaxing of vacuum distillates, and containing straight and branched chain saturated hydrocarbons, mainly in the range >C20.
Streams obtained by the solvent dewaxing of vacuum residues, and containing mainly branched chain saturated hydrocarbons, mainly in the range >C20.
Used or re-refined oil
Spent formulated oils derived from various uses, most of
which are treated by processes such as clay percolation, hydrogenation and distillation, and mainly in the range C15 to C50.
Bitumen or vacuum
Streams obtained as residues from vacuum distillation
and cracking processes, some of which are subjected to further processing, and containing saturated and aromatic hydrocarbons mainly in the range >C25
Granular or needle like substances, basically carbon,
contained by the high temperature decomposition of heavy oils. May contain some high molecular weight hydrocarbons.
*According to the Official Journal L84 of 5 April 1993
Case Study on Polyols
Mike Jeffs
ISOPA INPUT RIP3.3-2 TASK 3
CASE STUDY: POLYOLS
ISOPA has an interest in a range of polyols that have been defined by the European Union as ‘no longer polymers'. The polyols are composed of an initiator, together with a chain or chains of propylene oxide (PO) or mixtures of ethylene oxide (EO) and PO with a range of molecular weights. They contain a common functional group - the hydroxyl group of which large numbers are present in each chemical entity. Because of their polymeric nature and apparent lack of hazard these substances have hitherto, not been extensively tested for toxicity and ecotoxicity. ISOPA is now addressing this problem in anticipation of the implementation of the EU REACH (Registration, Evaluation and Authorisation of CHemicals) legislation [1] by developing a case for read across in respect of health effects for members of a set of polyols. The read across case for the ISOPA polyols essentially follows steps 1-5 of the process proposed by the OECD [2].
Step 1: Identification of the compounds of interest
ISOPA has grouped this product range into 10 categories, based on 9 initiators. The first nine
categories are propoxylated derivatives of sucrose, propylidine trimethanol, D-glucitol,
pentaerythritol, nitrilotriethanol, ethylenediamine, o-toluenediamine, glycerol and propylene glycol.
The tenth category is a mixed ethoxylated/propoxylated derivative of ethylenediamine. Their CAS
and EINECS numbers are shown in Table 1.
Step 2: Collection of data for each category member
Available test reports on individual polyols were collected from various ISOPA members. Literature searches were conducted and data collected concerning the toxicity of each initiator and of the repeating units (poly-PO and poly-EO). The data collection included undertaking a limited number of basic studies o fill obvious gaps.
Steps 3 and 4: Evaluation of available data for adequacy and construction of a matrix of data
availability
Only studies of at least a minimal acceptable quality for classification and labelling (i.e. Klimisch categories 1 and 2, as determined by ISOPA) were included. The information for each of the ten categories was set out in individual Microsoft Excel spreadsheets in a single file. The individual end points were given on the x-axis and the average molecular weight on the y-axis, beginning with information on the initiator as the first line and with each successive line being for the next higher molecular weight average. In general, no entry means that no study was available and the entry
gives the key toxicity information. In this sense the approach used was more detailed than that set out at step 4 of the OECD proposed process. The polyol chain units (propoxy and ethoxy) were also examined; they were found to be non-toxic. In a second approach, the relative bioavailabilities of each component within each of the 10 groups were calculated using QSAR techniques [3, 4]. In all cases, the calculated bioavailability of the individual molecular species decreased with increasing molecular weight.
Step 5: Assessment of the validity of the category
The polyol chain units were found not to be intrinsically toxic. It was therefore felt to be reasonable to consider the polyol as a common functional group (group 1.4.1 of Annex IX), and to consider the 10 polyols simply on the basis of their different initiators. A review of the toxicology of the 9 initiators showed that 7 of them were essentially non-toxic, whilst two - ethylenediamine and o-toluenediamine - exhibited some distinct and separate toxic properties, principally based on their free amino functions. These latter two initiators have been termed "reactive" initiators and the three polyols derived from them have been placed in two separate sub-categories reflecting the different toxic properties of their initiators. In both cases the toxicity rapidly decreased with increasing molecular weight of the polyol and they represent ‘a constant pattern in the changing of the potency of the properties across the category'. The toxicity data from polyols derived from the 7 "non-reactive" initiators was set against their calculated bioavailability profiles. Generally, the bioavailablility decreased with increasing molecular weight, thus, if the strength of any toxic effect depended on bioavailability it would be manifest at the lowest molecular weight and would decrease rapidly with increasing molecular weight. This would be in line with the assumption, normally made, that, for the majority of foreign compounds absorption depends on passive diffusion, although it is accepted that other mechanisms (e.g. facilitated diffusion, active transport) may be relevant when considering substances mimicking dietary constituents. The polyols derived from glycerol and propylene glycol were clear exceptions. They showed a systemic toxicity to rats over certain MW ranges. It was concluded that they are probably absorbed by a mechanism based on the absorption of dietary fats and fatty acids [5, 6]. It is a qualitative structure-activity relationship based on knowledge of mechanisms of absorption. The polyols from glycerol and propylene glycol were therefore placed in a separate sub-category from the other 5 "non-reactive" polyols. The four sub-categories can be summarised as: • Propoxylated derivatives from five essentially non-toxic initiators (sucrose, propylidine
trimethanol, D-glucitol, pentaerythritol and nitrilotriethanol). The polyols derived from these initiators are also essentially non-toxic
• Propoxylated and propoxylated/ethoxylated derivatives obtained from the ‘reactive' initiator
ethylenediamine, o-toluenediamine. These polyols showed decreased toxicity with increasing molecular weight as the effect of the initiator toxicity was ‘diluted out'.
• Propoxylated derivatives obtained from the ‘reactive' initiator o-toluene diamine. These polyols
showed decreased toxicity with increasing molecular weight as the effect of the initiator toxicity was ‘diluted out'.
• Propoxylated substances derived from the initiators glycerol and propylene glycol over certain
MW range. These polyols are probably absorbed by a mechanism other than passive diffusion.
Step 6: Preparation of a test plan for the four sub-categories
Following our review of the toxicology of the polyols within the four sub-categories defined above, we are now in a position to identify appropriate test substances to deliver the maximum level of useful information suitable for classification and labelling, with minimum cost and use of experimental animals. For some toxicological endpoints, it may be possible to combine some of the sub-categories for the purposes of read-across, thereby further reducing cost and use of animals.
Conclusion
We have demonstrated that, in the case of the polyols, the grouping principle based on (a) essentially non-toxic initiators and (b) common functional groups, meets the OECD categorisation process criteria and can be used in the "read across" for Annex IX of the proposed REACH regulation.
References
1. Draft REACH Regulation agreed by Competitiveness Council at its meeting on 13
December 2005. Council Document 15921/05. (available on the www at http://register.consilium.eu.int/pdf/en/05/st15/st15921.en05.pdf or via the ECB website)
2. Manual for investigation of HPV chemicals, Chapter 3: data evaluation, OECD Draft on
Chemical Categories 1947509.pdf
3. Suzuki T and Kudo Y. (1990) Automatic logP estimation based on combined additive
modelling methods. Journal of Computer-Aided Molecular Design, 4, 155-198.
4. Potts R.O. and Guy R. (1992) Predicting skin permeability. Pharmaceutical Research, 9,
5. JECFA (2001). Safety evaluation of certain food additives and contaminants. Aliphatic
acyclic diols, triols and related substances. WHO Food Additives Series 48
6. Tyler, T R (1999). Peroral toxicity. In ‘General and Applied Toxicology', Second edition
(edited by B Ballantyne, T Marrs and T Syversen), 1, 543-559.
Table 1. CAS and EINECS numbers of initiators and their respective polyols
Initiator
EINECS Polyol chain
CAS No of Polyol
107-15-3 203-468-6 propoxylated
ethoxylated and propoxylated
D-Glucitol
200-061-5 propoxylated
Glycerol
200-289-5 propoxylated
102-71-6 203-449-8 propoxylated
115-77-5 204-104-9 propoxylated
9051-49-4(25214-63-5)* 500-030-9
200-338-0 propoxylated
201-074-9 propoxylated
200-354-9 propoxylated
2687-25-4 220-248-5 propoxylated
* two CAS numbers given for this substance
Possible Application of QSAR Methods to Organic Chemicals
Andrew Worth, Arianna Bassan, Ana Gallegos, Tatiana Netzeva, Grace
Patlewicz, Manuela Pavan & Ivanka Tsakovska
European Chemicals Bureau
30 September 2006
Possible Application of QSAR Methods to Organic Chemicals
This appendix aims to summarise and illustrate some of the different ways in which QSAR methods can be used in developing chemical categories. In the context of this document, the term QSAR method is interpreted in the broadest sense to incorporate analogue-searching methods, statistical methods for exploratory data analysis and model development, as well as existing computational prediction methods based on SARs and/or QSARs. A more detailed description of one aspect of this ECB investigation (including methods, materials and detailed results) has been published separately (see 1). There are a number of steps in the development and assessment of a chemical category as described below. In principle, QSAR methods can be used in any one or more of these stages.
Assessing membership of existing categories
Developing a category hypothesis and category definition
Gathering data for the analogues
Evaluation of data adequacy
Assessing the adequacy of the read-across or category
Guiding further testing
The findings from this limited investigation demonstrate that QSAR methods can provide a useful
supplement to non-formalised approaches, especially when developing the category hypothesis and
defining the category in terms of its members and endpoints (stage 2).
1.2
Background information on regulatory assessments
We emphasise that the main aim of this investigation was to explore and illustrate how different QSAR methods could be used in the formation of chemical categories, using a dataset of phthalate esters as an example of a category of organic chemicals. The purpose was not to re-evaluate any substance-specific data nor the conclusions made in the above-mentioned regulatory assessments of specific phthalate esters. Solely for completeness and as background information, it is noted that various regulatory assessments have been conducted on phthalate esters:
a) an OECD SIAM category on a set of seven high-molecular weight phthalate ester
(HMWPE) mixtures has been developed (2)
b) EU risk assessments have been completed for two higher molecular weight esters (3,4) c) EU harmonised classifications have been agreed for seven phthalate esters (5) d) A total of 14 phthalate esters were considered during an initial screening exercise by the EU
PBT Working Group (6).
Assessing membership of existing categories
A question that is likely to arise under REACH is whether a new substance is sufficiently similar to
the existing members of a category to be reasonably regarded as a member of that category and
consequently to be evaluated in the same or similar way. This question can be addressed, to some
extent, by using computational approaches. However, in order to apply QSAR modelling approaches, it is first necessary to characterise the existing members of the category in terms of their chemical identities. In this investigation, the SIAM category of HMWPEs (2) was examined to determine membership and scope (Table 1). The seven members of this category are all multi-component substances, which makes it more complicated to characterise them for modelling purposes. In the case of complex multi-component substances, the computational toxicologist has several options for the representation of each substance:
a) to consider all possible components in the mixture (if they are known or suspected, and if
the composition indicates approximately equal proportions of the components);
b) to consider the dominant component in the mixture, i.e. to select a representative structure
on a basis of the relative (molecular) weight;
c) to select a representative structure that reflects the chain length and the branching of the
Furthermore, since the substances belong to a category, and the question being asked is whether a
chemical is a reasonable member of that category, an additional consideration might be to include in
the selection structures that represent the boundaries of the category (and possibly any
subcategories). The inclusion of such structures needs to be judged on the basis of the available
information on the applicability domain (and subdomains) of the category. This is not necessarily
straightforward because, for administrative reasons, chemical categories tend to be defined in terms
of the actual members (of commercial interest and subject to the requirements of a particular
regulatory programme), rather than all possible members that can be conceived by applying the
considerations of combinatorial chemistry, irrespective of whether such members are actually
produced or marketed. For example, in addition to listing its seven members, the HMWPE category
is defined as "esters with an alkyl carbon backbone with 7 carbon atoms or greater". This provides
no indication of the upper limit of the carbon atoms in the side chains nor for the degree of
branching that might result in similar physicochemical and toxicological profiles. This adds to the
arbitrary nature of selecting representative structures for the category.
For the purposes of this study, and in the absence of composition information, the selection of a
single representative structure for each mixture was carried out for simplicity. CAS numbers and
other information on chemical identity provided in the OECD report (2) were therefore used to
draw a representative 2D chemical structure for each ester (Table 2). These 2D structures were then
used as seeds to illustrate hypothesis development and analogue identification (section 1.4). The
QSAR predictions subsequently made for these chemicals apply to individual chemicals and do not
aim to re-evaluate the SIAM HMWPE category, which is based on mixtures of isomers.
1
1.4
Developing a category hypothesis and category definition
Our starting hypothesis was that phthalate esters as individual chemicals show common properties and trends on account of the phthalate moiety. Clearly, on the basis of existing knowledge, we
1If it were the intention to evaluate and further develop the existing SIAM category, it would be desirable to obtain data on the composition of the HMWPE mixtures, both in terms of the discrete chemical structures and their mass distribution.
know this generalisation to be flawed, but for the purposes of exploring how different computational (and other) methods can be used to define suitable applicability domains for different endpoints, it was a convenient starting point. It also should be emphasised that this investigation aimed to simulate a situation where no category already existed within any regulatory programme.
1.4.1 Strategy for analogue searching
An early step in performing read-across, in developing a new category, or extending an existing one, is to search for possible analogues for which experimental data might be available. In this investigation, we used seven analogues (Table 2) which were considered representative structures of the seven mixtures in the SIAM category2. These analogues were used to help search for both higher and lower molecular weight phthalate esters. The following publicly available and commercial tools were used to identify analogues:
a) US EPA Analog Identification Method (AIM): http://esc.syrres.com/analog b) AMBIT (IDEA Ltd): http://ambit.acad.bg c) Danish (Q)SAR Database: http://ecbqsar.jrc.it d) Chemfinder: http://www.chemfinder.com e) ChemID plus: http://chem.sis.nlm.nih.gov/chemidplus f) Leadscope: http://www.leadscope.com
The main searching methods included substructural searches and the use of fingerprints or fragments in combination with the Tanimoto or modified Tanimoto coefficient as the similarity metric (Figure 1). A total of 558 analogues were retrieved, including replicates of the same chemicals. Removal of these replicates gave rise to a unique set of 341 chemicals. The set of 341 chemicals had the following characteristics:
a) The total number of carbons varied between 8 and 58 (the simplest molecular formula was
C8H6O6 and the most complex wasC58H84O12S3Sn);
b) Molecular weights varied between 164.2 and 1188.2; c) Log Kow values varied between -1.38 and 18.36.
Figure 2 gives the distributions of molecular weight and hydrophobicity within the compiled set of phthalate esters and within the set of representative HMWPE structures. The figure illustrates the potential to extend the original HMWPE category with additional chemicals, both in terms of MW and hydrophobicity. Chemicals with molecular weight higher than approximately 600 tend to break the normal distribution of this property, and are statistical outliers in the distribution. The inclusion of such chemicals in a new or extended category should be considered with caution, since they are atypical of the majority of chemicals in the distribution. The distribution of hydrophobicity (logKow) is further examined in Figure 3, which is suggestive of two overlapping distributions, with median values around logKow values of 3.5 and 9.0. Figure 2 also shows that the representative HMWPE structures occupy a physicochemical domain above the mean MW of the larger, compiled distribution (which is consistent with the name of the
2 The appropriate treatment of mixtures, for example by choosing representative structures, depends on the purpose of the investigation. Representative could mean most prevalent in the mixture or most typical in terms of physicochemical, environmental and/or toxicological properties. In this study, there was no attempt to analyse the structural variation within each mixture, because it was not the purpose to produce assessments for the SIAM chemicals.
SIAM category). A large number of phthalate structures with lower molecular weights were also identified. As a result of the analogue searching, it was found that:
a) analogue searching can be performed in multiple ways, depending on the specific search
engine used. The databases underpinning these search engines differ in size and scope. In addition the search options offered can vary e.g. use of more or less detailed substructures and different similarity measures;
b) due to the lack of a unique correspondence between chemical name and CAS number,
searching by chemical name can result in multiple CAS numbers being retrieved. Similarly, searching by CAS number can give rise to multiple names (and different structures). Thus, it is necessary to check the results obtained.
1.4.2 Exploratory analysis: identifying similarities, differences and outliers
In the absence of any experimental data, various exploratory data analysis methods were applied to the data set of 341 analogues. Such methods provide a convenient means of visualising relationships between chemicals to explore their similarities as well as to identify possible outliers. In addition to visualising possible outliers in a single descriptor space (as shown in Figures 2 and 3), a common statistical method for exploratory data analysis is principal components analysis (PCA). This can be used to visualise the chemical space of a dataset. PCA is a method that manipulates a multi-dimensional dataset to provide two-dimensional or three-dimensional cross-sections that capture as much of the variance in the data set as possible. Before applying PCA, a large number of descriptors were generated by using the following commercial programs: Accord for Excel (Accelrys Inc), TSAR (Version 3.3, Accelrys Inc) and DRAGON (Talete srl). Descriptors were computed for 323 of the 341 phthalates. For the remaining 18 chemicals either the SMILES code (which is the input to the software) was unavailable or could not be processed by one of above-mentioned software programs (e.g. in the case of salts or polymers). The following types of descriptors were calculated:
a) constitutional descriptors (40 descriptors), which are zero-dimensional counts of atomic
features within a molecule
b) topological descriptors (89 descriptors), which capture 2D information including structural
features such as shape, symmetry, branching and cyclicity
c) molecular connectivity indices and information indices (80 indices), which account for bond
accessibility within intermolecular interactions (connectivity indices) and total information content (descriptors based on the application of information theory to chemical graphs)
d) geometrical descriptors, which capture 3D information (295 descriptors) e) physicochemical properties (12 properties), i.e. descriptors that estimate experimental
properties such as logKow and boiling point.
PCA plots were generated for different combinations of these descriptors, as well as for all descriptors (to capture as much chemical information as possible). As an example, Figure 4 shows a PCA plot based on the combined use of connectivity and information indices. The plot captures 71.6% of the total variance in the data and reveals a number of statistical outliers (data points outside the ellipse). It can also be seen that the seven representative members of the SIAM category (red points) are within the ellipse and surrounded by many other analogues. In other words, the representative HMWPE chemicals are similar to the many of the analogues on the basis of the chosen descriptors.
The PCA plots are useful in two ways:
a) they help to identify statistical outliers, i.e. chemicals that are likely to behave differently,
and hence are candidates for exclusion from a category.
b) they illustrate how the analogues are clustered together, hence which analogues are similar
with respect to the chemical information computed (i.e. the connectivity and information indices).
Figure 4 indicates that there are four significant statistical outliers in the lower left corner, which are illustrated in Figure 5. These are chemicals that responded to some of the analogue search criteria but are in fact different from the others for some other reason. Therefore, if experimental data were available for these outliers, it would not be advisable to read-across these data to the other candidates and vice versa. The analysis of these outliers emphasises the point that when an automatic search of analogues is performed, the identified candidates might not all be suitable for the selection purpose (i.e. they might not be suitable candidates for the category) and should therefore be analysed critically. Figure 4 illustrates the other useful feature of the PCA plots, namely their utility in identifying chemicals that are grouped closely together with very similar structures. Read-across between such chemicals is more likely to be adequate (but this should still be analysed critically).
1.4.3 Exploratory analysis: building a matrix of estimated endpoints
In the absence (or paucity) of experimental data (as was the case with the data set here), existing QSAR models can be used to: a) identify chemicals with common hazards; b) predict trends in the potencies of such hazards; and c) identify possible breakpoints in trends, therefore possible subcategories.3 To illustrate this approach, the data set of analogues was extended to include predictions for a number of endpoints. The result was a large data matrix in which the phthalates were represented by different rows and the following human health and environmental endpoints were represented by different columns:
1) skin sensitisation, calculated with TOPKAT 2) skin irritation, calculated with TOPKAT 3) acute oral mammalian toxicity, calculated with TOPKAT 4) developmental toxicity, calculated with TOPKAT 5) persistence, calculated with BIOWIN3 (ultimate biodegradation model) 6) bioaccumulation, calculated with BCFWIN 7) acute fish toxicity (96h fathead minnow), calculated with ECOSAR and TOPKAT
These endpoints were chosen to cover a variety of endpoints that might normally be of interest in the development of a category. The choice was not intended to be comprehensive, nor were the endpoints chosen considered to be of any particular importance for the assessment of phthalate esters, since the exercise was based on the assumption of little or empirical knowledge.4
3 If sufficient reliable experimental data are available, it should also be possible to develop new QSARs that reflect the observed trends. In such a case, new QSAR model development is an integral part of the formation of the category. 4 In reality, evidence from regulatory assessments points to concerns for developmental toxicity and aquatic toxicity, at least for certain phthalate esters. However, this was not directly relevant to this exercise exploring the possible uses of QSAR methods.
The different software packages used were chosen simply for convenience (available in-house and capable of running in batch mode). BIOWIN3, BCFWIN and ECOSAR are available as free-ware from the US EPA (http://www.epa.gov/oppt/exposure/docs/episuitedl.htm). TOPKAT (Version 6.2) is developed and commercialised by Accelrys Inc (San Diego, CA, USA). The data matrix generated is the usual starting point for any (Q)SAR modelling or chemometric ranking exercises. In fact, the data for persistence (P), bioaccumulation (B), and fish toxicity (T) were used to explore the applicability of ranking methods as a means of evaluating chemicals according to their PBT properties (see Case study on ranking approaches). A major effort in constructing the data matrix was in obtaining the identities of the chemicals and, as far as possible, the structural representations, (e.g. SMILES codes). This process can involve some arbitrary decisions. For example, as mentioned above, in the case of the seven SIAM phthalates, each member is defined by a CAS number but this actually refers to a mixture of isomers, with varying lengths of the side chains and branching patterns. In such a case, it is either necessary to identify a "representative" structure for each isomeric mixture, or to analyse the structural variation within the mixture, depending on the purpose of the investigation. On the basis of such a matrix of estimated endpoints, it should be possible to gain an impression of whether the group of analogues is likely to form a robust category, or whether it needs to be redefined by removal and/or addition of analogues. It should also be possible to identify subgroups containing different trends that might form the basis for subcategories. In the matrix of 323 phthalate esters generated in this study, different trends were observed for different endpoints (which is not surprising given the size of the matrix). These trends are not discussed at length here. However, two examples are provided in an attempt to illustrate how phthalate esters could be grouped (in an endpoint-specific manner) for aquatic toxicity and skin sensitisation.
Exploratory analysis: identifying possible subcategories for aquatic toxicity
The availability of QSAR estimates for the members of a potential category enables trends to be identified in the absence of experimental data, as well as possible breakpoints in those trends. This allows a preliminary identification not only of possible category boundaries but also possible divisions into subcategories, some of which may be endpoint-specific. Under the current EU classification and labelling system, the following risk phrases are used for environmental hazard classification on the basis of aquatic toxicity:5
- R50 (Very toxic to aquatic organisms)
- R51 (Toxic to aquatic organisms)
> 1 and < 10 mg/L
- R52 (Harmful to aquatic organisms)
> 10 and < 100 mg/L
5 In addition, R53 may be assigned if there is potential for long-term adverse effects in the environment. The prediction of R53 was not considered in this investigation.
The same cut-off values also apply if 48h EC50 to Daphnia or other crustaceans, and 72 or 96h EC50 for algae or other aquatic plants, are used. If the LC50 (EC50) value is above 100 mg/L, it is considered that there is no concern (NC) for acute toxicity to aquatic organisms. For the purposes of the illustration in this section, it is assumed that a category is a chemical group in which a trend (e.g. increasing toxicity with increasing hydrophobicity) can be observed while a subcategory is a smaller group, in which the toxicity varies within a narrower range due to subtle structure modifications (but where the mechanism of action does not change). The assumption of a common mechanism of action is the "unifying feature" between the category members but the category as a whole does not provide a suitable basis for read-across unless subcategories are also defined. Thus, a read-across between members of the same category should be allowed because the result will not change the classification result (classification categories and cut-offs being preferably defined in formal way). However, read-across between members of different subcategories might produce predictions that are as unreliable as from members of different categories. To explore the trends in acute toxicity to fish, ECOSAR was used to predict the LC50 values for toxicity to fathead minnow6. The predicted LC50 values generated by ECOSAR were compared with those generated by TOPKAT (only predictions in the TOPKAT optimum prediction space were considered). Figure 6 shows how the two models correlate with each other (r2 = 0.8). Two additional observations can be made from Figure 6:
a) TOPKAT makes more conservative predictions than ECOSAR (i.e. predicts higher toxicity
for the same chemicals,) for a large number of chemicals. This is particularly evident for the higher predicted values. Systematic differences in the results obtained by different QSAR models should be taken into account if the model estimates on their own are to be relied upon for classification and labelling and/or risk assessment purposes.
b) A large number of phthalates are predicted as very toxic by both programs. The reasons for
this observation was analysed further and is further discussed below.
ECOSAR has multiple models, each of which is applicable to a specific chemical class. The model for esters (Equation 1) was used, unless one of the other models gave a more conservative value (lower LC50).
Log LC50 = -0.535 logKow + 0.25
ECOSAR produces warnings relating to the applicability of the estimates (e.g. when the water solubility is very low, or when the prediction is outside the range of logKow values in the model training set). If used alone, Equation 1 assumes that toxicity increases in a linear fashion as hydrophobicity increases (i.e. a monotonic increasing linear trend), yet numerous studies (e.g. Hermens et al, 7) have shown that a parabolic (i.e. non-monotonic) relationship between logLC50 and logKow is more realistic and better predicts toxicity at higher values of logKow (Figure 7). Therefore, to take this into account, a simple bilinear relationship was derived (Figure 8). This relationship was used to develop a decision rule approach, i.e. rules-of-thumb for classifying chemicals for acute fish toxicity on the basis of estimated logKow values (Table 3). The rules do not take into account possible metabolism of the esters the possible effects of metabolism on the toxicity.
6 The ECOSAR and EPIWIN programs were able to process 324 smiles codes and therefore make predictions for 324 phthalate esters.
In developing the decision rules, the relationship between the solubility limit and LC50 was also taken into account. If the solubility is less than the predicted LC50, then the solubility is limiting. On this basis, it was assumed that if the water solubility of a phthalate ester, calculated with WSKOWWIN, was less than 0.002 mg/L, then the chemical was not sufficiently soluble to be classified as toxic. This solubility value corresponds to a logKow of approximately 8 and places a large number of the category candidates in the NC group (Figure 8). However, this does not exclude the possibility of long-term chronic toxicity effects. At the border line of the solubility limit, more data should be compiled to define it better, especially for the toxicity of di-C6-C7 (and even C8) analogues, for which little or no data were found in this study. It is possible that around the limit of aqueous solubility, the branching of the side chains plays a significant role, but this hypothesis needs to be studied further. Application of these rules to the seven SIAM phthalate representative structures led to predictions of NC, which corresponds with available experimental data (Table 4). We emphasise that these decision rules are based on a preliminary investigation, and are presented to illustrate the application of a QSAR approach for defining possible subcategories based on structural and/or physicochemical rules. A larger set of experimental data would be needed to validate (and most likely refine) these decision rules. Validation of the decision rules would be especially important if they were used as the basis for (or to support) classification and labelling. Furthermore, it is anticipated that in regulatory practise, such rules would be used as "rules of thumb" rather than as prescriptive rules. In particular, expert judgement would be especially important for those chemical lying on or close to classification boundaries.
Exploratory analysis: identifying possible subcategories for skin sensitisation
A qualitative read-across can be regarded as equivalent to the use of a SAR based on the common
substructure. In developing a SAR, the greater the number of analogues identified, the greater the
possibility of understanding the consequences of structural variation, i.e. the greater the possibility
of defining an applicability domain (AD) for the SAR. Ideally, the AD should be defined by using
experimental data to identify the known consequences of structural variation. However, such data is
not always available, especially in the case of large homogeneous datasets (e.g. the dataset of 341
phthalate esters). However, in such cases, an exploratory analysis can be performed on the basis of
predicted data, to identify the possible consequences of structural variation.
As an example, a substructure-based clustering method within Leadscope (Leadscope Inc,
Columbus, OH, US) was applied to 79 predicted non-sensitising phthalates and 29 predicted
sensitising phthalates. This identified two main "substructural signatures" that were representative
of non-sensitisers and sensitisers, respectively (Figure 9). The signatures themselves do not
represent any single chemical in the group; instead they sum up the overall features of that group.
This analysis indicated that phthalate esters containing a single ester group, or containing an
additional functionality on the benzene ring (e.g. a nitro or chloro group), or containing branching
on the acyl chain, were more likely to form a different subcategory to the typical SIAM diesters (i.e.
these structural variants were more likely to be sensitising rather than non-sensitising).
1.5
Gathering data – filling data gaps with estimated values
Under REACH, in the absence of reliable experimental data, it will be possible to fill data gaps by using valid QSAR models. Models used should be validated in accordance with the OECD principles for QSAR validation. As far as possible, the predictions and trends established by QSAR
methods should be verified by comparison with experimental data. This should be feasible for
category approaches, since experimental data should be available for at least some of the category
members.
It may be the case that a QSAR predicts the trend correctly, but the numerical values of the
predictions are consistently high or low. In such cases, the QSAR could be used to support the
trend, but the experimental data should be relied upon when performing an interpolation (or even
extrapolation).
In this study, the predictions made by the above-mentioned models for the seven representative
structures were compared with experimental data for the seven SIAM phthalates. Table 4
summarises the experimental data for the seven endpoints, to the extent it was provided in the SIDS
Initial Assessment Report (SIAR; 3), and Table 5 provides the corresponding QSAR predictions.
Irrespective of the endpoint predicted, TOPKAT predictions are associated with an indication of
their reliability, according to whether the chemical of interest lies within the AD of the model. This
is an assessment of whether the chemical lies within the structural and physicochemical descriptor
space of the training set. However it is up to the end-user to decide how to interpret this
information. For the purposes of this study, a strict criterion was adopted – a prediction was only
considered to be reliable if the chemical was found to be within the structural and descriptor space.
For developmental toxicity and acute fish toxicity, none of the TOPKAT predictions was reliable.
For skin sensitisation, four phthalates were correctly predicted to be non-sensitisers, while the other
three were outside the AD. In the case of skin irritation, six predictions of non-irritancy were made,
of which four were correct and two could not be evaluated, due to lack of experimental data in the
SIAR. For eye irritation, TOPKAT made six predictions, including three correct predictions of
known non-irritants, a prediction of non-irritancy for a phthalate with no experimental data, a
prediction of mild irritancy for a phthalate with no experimental data, and a prediction of mild
irritancy for a non-irritant.
For bioconcentration, there was full concordance between the seven BCFWIN predictions of no
bioaccumulation potential and the experimental data reported in the SIAR.
For biodegradation, there was a good concordance between the BIOWIN predictions by
experimental data reported in the SIAR (where data were available). Experimentally it was
observed that lower molecular weight ranges are expected to biodegrade to a high extent (greater
than 60% after 28 days), while higher molecular weight members are expected to biodegrade to an
extent less than 60%. BIOWIN predictions are in agreement with these results, with Di-phC10 PE
(53306-54-0) and Di-C11 PE (3648-20-2) being predicted to biodegrade within weeks, and Di-C13
PE (68515-47-9) and Di-C13 PE (119-06-2) in months.
The TOPKAT model for acute oral toxicity (rat oral LD50) predicted all seven phthalates to be non-
toxic, but five of these predictions were out of the domain.
1.6
Assessing the adequacy of experimental data
Data adequacy can be evaluated with reference to Klimisch codes (8) or the OECD Guidance for Determining the Quality of Data for the SIDS Dossier (section 3.1 of the OECD Manual for Investigation of HPV Chemicals).
Reliable (Q)SAR predictions can contribute to the evaluation of data adequacy by providing
supplementary information. For example, the deviation of a chemical from an experimentally
observed trend could be attributable to a change in the mechanism of toxic action, as reflected by
the presence of a structural alert. Alternatively, the chemical could have a different toxicokinetic
behaviour, which might be predicted by a QSAR method. In the case of test data for aquatic
toxicity, comparison of predicted and experimental results can explain "unreliable" test results. For
example, a measured LC50 for acute fish toxicity that lies above the theoretical minimum LC50
predicted by a baseline QSAR model could indicate that adsorption or volatilisation has occurred in
the test system.
In other cases, the deviation of a chemical from an experimentally observed trend that cannot be
rationalised in mechanistic terms of mechanism or experimental artefact could simply be a
reflection of an unreliable measurement.
In other cases, a reliable QSAR estimate could be used to select an experimental value when a range
of test data are available and of uncertain quality. In such a case, the QSAR estimate can add to the
weight of evidence in making a decision based on the experimental data point.
1.7
Assessing the adequacy of read-across (impact of structural variation)
One of the difficulties in applying read-across is the uncertainty of whether a small structural difference between the source and target chemicals could invalidate the read-across. QSAR analysis can help to establish the adequacy of the read-across in cases where the (Q)SAR captures the factor(s) responsible for driving the (eco)toxicological effect. For example acute aquatic toxicity is typically modelled with logKow. For illustrative purposes, it can be assumed that data for this endpoint is missing for diethyl phthalate (Figure 10), but there are several choices of analogue for the read-across. Assuming the same mechanism of action holds, read-across from 1,2-benzenedicarboxylic acid, bis(2-hydroxyethyl) ester is likely to lead to an underestimation of the acute aquatic hazard, whereas read-across from diethyl 3,4,5,6-tetrachlorophthalate is likely to lead to overestimation. In other words, neither read-across would be adequate, even though the three example chemicals have two carbon atoms in the ester side chains. This is because introduction of hydrophilic groups (in the 1,2-benzenedicarboxylic acid, bis(2-hydroxyethyl) ester) reduces the logKow (hydrophobicity) to 0.12, whereas introduction of halogen atoms in the benzene ring increases the logKow to 5.22 (the latter chemical, however, might show a deviation from the predicted toxicity due to the possible effect of the halogen atoms on the hydrolysis rate). Conversely, another analogue (1,2-Benzenedicarboxylic acid, bis(2-ethoxyethyl) ester) has a similar logKow of 2.10. Thus, read-across from this analogue is therefore likely lead to the correct hazard classification (harmful), although looking at the side chain, it looks more dissimilar to diethyl phthalate than the other two analogues. Thus, the use of a valid QSAR can help to choose an appropriate analogue and support the adequacy of the read-across. The general assumption that all chemicals sharing a common substructural fragment show similar (eco)toxicological profiles fails when the structural analogues are able to act via different (or multiple) mechanisms of action (see Figure 10). For example, allyl 2,3-epoxypropyl phthalate can act as an alkylating agent towards proteins and DNA, dimethyl 3,6-dihydroxyphthalate can easily transform to a strong electrophile (quinine form), and 1,2-benzenedicarboxylic acid, 2-hydroxyethyl 2-[(1-oxo-2-propenyl)oxy]ethyl ester can be a strong electrophile itself due to the presence of an acrylate moiety in the molecule. Such chemicals should not normally be predicted by the same QSAR (unless the QSAR is developed specifically to capture more than one mechanism). Furthermore, such chemicals should either be excluded from a category based on a narcotic mode of action, or they should be included as a subcategory.
In other words, a category should be developed with mechanistic considerations in mind, to enable
adequate read-across to be carried out within the same category or subcategory. The scientific
challenge is to define appropriate structural rules and/or physicochemical cut-off values for defining
these subcategories.
1.8
Conclusions
The investigation described here, which used the existing SIAM category of phthalate esters as a starting point, aimed to explore and illustrate some of the ways in which QSAR methods can be applied in the development of chemical categories. It was not the aim of this investigation to further justify the SIAM category itself, to develop an alternative and extended category of phthalate esters, or to make proposals for the classification and labelling of specific chemicals. It was rather intended to simulate some of the questions that arise in de novo category development, and explore how computational toxicology can help.
In particular, the investigation resulted in the following learnings:
a) There are a number of search engines available for the identification of analogues for read-
across. These provide different analogues on account of the database covered and the similarity measure used. The different approaches vary in terms of their ease-of-use and their capacity for data-mining. Errors were discovered which propagated between different databases, which highlighted the importance of checking any results obtained.
b) Data exploration tools, such as PCA and clustering, are useful to enable visualisation (in 2D
or 3D) of the chemical domain of a set of compounds to look for obvious groups of "like" compounds. These approaches rely on a starting dataset of chemicals and computing different
physicochemical, electronic descriptors) for those chemicals or characterising them through the use of structural fingerprints.
c) The use of predictions from existing QSARs can be useful to explore trends within groups
of chemicals or help in the assessment of data adequacy. Structural fingerprints and cut-off values along descriptors (e.g. physicochemical properties) can be useful to gain insights about the scope and boundaries of a category (and subcategories).
d) QSARs that encode the descriptor(s) driving an endpoint can be helpful in assessing the
adequacy of a read-across (i.e. assessing how similar an analogue is to the chemical of interest with respect to a given endpoint).
Finally, QSAR methods should be seen as supplementary tools that are useful in the development of
categories of certain kinds of chemicals, rather than as an automated substitute for more
conventional approaches to category formation.
1.9
References
1. Netzeva TI & Worth AP (2007). Classification of phthalates according to their (Q)SAR
predicted acute toxicity to fish. A Case study. JRC report EUR 22623 EN. European Chemicals Bureau, Joint Research Centre, European Commission, Ispra, Italy. http://ecb.jrc.it.
2. OECD (2004). SIDS Initial Assessment Report for SIAM 19. Berlin, Germany, October
3. EC (2003). EU RAR on 1,2-Benzenedicarboxylic acid, di-C8-10-branched alkyl esters, C9-
rich (DINP), CAS. No. 68515-48-0 Volume 35. Available from the ECB website: http://ecb.jrc.it/esis/esis.php?PGM=ora
4. EC (2003). EU RAR on 1,2-Benzenedicarboxylic acid, di-C9-11-branched alkyl esters, C10-
rich (DIDP), CAS. No. 68515-49-1. Volume 36. Available from the ECB website: http://ecb.jrc.it/esis/esis.php?PGM=ora
5. The ECB CLASSLAB database (http://ecb.jrc.it/classification-labelling) identifies the
following entries:
i) Annex I entry 607-317-00-9: bis(2-ethylhexyl) phthalate; di-(2-ethylhexyl) phthalate; DEHP (CAS No. 117-81-7; EC No. 204-211-0): Repr. Cat. 2; R60; Repr. Cat. 2; R61.
ii) Annex I entry 607-318-00-4: dibutyl phthalate; DBP (CAS No. 84-74-2; EC No. 201-557-4): Repr. Cat. 2; R61; Repr. Cat. 3; R62; N; R50.
iii) Annex I entry 607-426-00-1: 1,2-benzenedicarboxylic acid, dipentylester, branched and linear (CAS No. 84777-06-0; EC No. 284-032-2): Repr. Cat. 2; R60; Repr. Cat. 2; R61; N; R50.
iv) Annex I entry 607-426-00-1: n-pentyl-isopentylphthalate (CAS No. -; EC No. -): Repr. Cat. 2; R60; Repr. Cat. 2; R61; N; R50.
v) Annex I entry 607-426-00-1: di-n-pentyl phthalate (CAS No. 131-18-0; EC No. 205-017-9): Repr. Cat. 2; R60; Repr. Cat. 2; R61; N; R50.
vi) Annex I entry 607-426-00-1: diisopentylphthalate (CAS No. 605-50-5; EC No. 210-088-4): Repr. Cat. 2; R60; Repr. Cat. 2; R61; N; R50.
vii) Annex I entry 607-480-00-6: 1,2-benzenedicarboxylic acid di-C7-11-branched and linear alkylesters (CAS No. 68515-42-4; EC No. 271-084-6): Repr. Cat. 2; R61; Repr. Cat. 3; R62.
6. ECB (2002). Identification of potential PBTs or vPvBs among the IUCLID High Production
Volume Chemicals. Doc ECB 4/14/02(pbt strategy – report)_rev1. Date 21 November 2002.
7. Hermens J., Leeuwangh P. & Musch A. (1984). Quantitative structure-activity relationships
and mixture toxicity studies of chloro- and alkylanilines at an acute lethal toxicity level to the guppy (Poecilia reticulate). Ecotox Environ Safety 8: 338-394.
8. Klimisch HJ, Andreae M & Tillmann U. (1997). A systematic approach for valuating the
quality of experimental toxicological and ecotoxicological data. Reg Tox Pharmacol 25: 1-5.
Table 1. Membership of the SIAM category of High Molecular Weight Phthalate Esters
1,2-benzenedicarboxilic acid, di-2-
R = C10H21 (propyl branched) [100% branched]
propylheptyl ester
1,2-benzenedicarboxilic acid, di-C7-9-
R = C7H15 to C9H19 (branched and linear)
branched and linear alkyl esters
[>80% linear]
1,2-benzenedicarboxilic acid, di-C11-
R = C11H23 (branched, essentially methyl, and
branched and linear alkyl esters
1,2-benzenedicarboxilic acid, di-C9-11-
R = C9H19 to C11H23 (branched and linear)
branched and linear alkyl esters
[>80% linear]
1,2-benzenedicarboxilic acid, di-C11-
R = C11H23 (branched)
1,2-benzenedicarboxilic acid, di-C11-
R = C11H23 (branched, essentially methyl)
14-branched alkyl esters, C13 rich
1,2-benzenedicarboxilic acid, di-C13-
R = C11H23 (branched)
Table 2. Representative structures for the seven SIAM phthalate esters
CAS number
Structure
CAS number Structure
1) 85507-79-5
5) 53306-54-0
2) 68515-47-9
6) 3648-20-2
7) 119-06-2
[1] 1,2-Benzenedicarboxylic acid, di-C11-branched and linear alkyl esters (Di-C11 PE) [2] 1,2-Benzenedicarboxylic acid, di-C11-14-branched alkyl esters, C13-rich (Di-C13 PE) [3] 1,2-Benzenedicarboxylic acid, di-C9-11-branched and linear alkyl esters (Di-C9-11 PE) [4] 1,2-Benzenedicarboxylic acid, di-C7-9-branched and linear alkyl esters (Di-C7-9 PE) [5] 1,2-Benzenedicarboxylic acid, di-2-propylheptyl ester (Di-phC10 PE) [6] 1,2-Benzenedicarboxylic acid, di-C11-alkyl ester (Di-C11 PE) [7] 1,2-Benzenedicarboxylic acid, di-C13-alkyl ester (Di-C13 PE)
Table 3. Rules-of-thumb for the classification of phthalates on the basis of estimated logKow
Log Kow range
Toxicity group
Acute fisha toxicity
value from
Chemical
log Kow < 1.5
1.5 < log Kow < 3.2
Diethyl phthalate
29.60; 17.00; 17.00; 16.80;
3.2 < log Kow < 5.0
Dibutyl phthalate
5.0 < log Kow < 7.0
Dipentyl phthalate
Dihexyl phthalate
(CAS 68515-50-4)
7.0 < log Kow < 8.0
log Kow > 8.0
Di-sec-octyl phthalate
R1 = R2 => C8H17
a Due to a large number of values available and for simplicity, only LC50 to fathead minnow is shown b N: Classified in Annex I of Directive 67/548/EEC as "Dangerous for the environment" c Classified in Annex I of Directive 67/548/EEC as "Very toxic to aquatic organisms" d Collected from http://www.epa.gov/opptintr/chemtest/pubs/alkpht.pdf e Not toxic below the limit of water solubility
Table 4. Experimental data for the seven SIAM phthalates
Abbreviation Developmental Acute fish
Toxicity
toxicity
Irritation
Irritation
Acclimated
LD50, g/kg
Inoculum
Inoculum
85507-79-5
68515-47-9
68515-43-5
68515-41-3
53306-54-0
3648-20-2
119-06-2
a No developmental toxicity signs are observed below maternotoxic doses
b Data were provided in the SIAR for several different species of fish (but not fathead minnow), invertebrates, and algae. All valid studies show that members of the HMWPE
Category do not produce acute toxicity to fish and invertebrates or toxicity to algae at their maximum solubility in the various media
Table 5. Predicted endpoints for the seven (representative structures of) SIAM phthalates
Acute fish
Acute fish
toxicity
Ultimate
al Toxicity
toxicity
(96h LC50 to P.
Irritation
Irritation
degradation
LD50, g/kg
(96h LC50 to P.
promelas)a
promelas)
85507-79-5
68515-47-9
68515-43-5
68515-41-3
53306-54-0
3648-20-2
119-06-2
aThe acute fish toxicity (96-h LC50 to P. promelas) in this column was judged on a basis of octanol-water partition coefficient (log Kow) and water solubility (WS, mg/L), calculated by WSKOWIN v. 1.41. All representative chemicals had calculated log Kow ≥ 8.5 and calculated water solubility below 0.001 mg/L. Therefore, they were considered not (acutely) toxic because they are below the limit of aqueous solubility (NTBLAS). This does not exclude possible concern for prolonged/chronic toxicity. NA = not applicable (out of domain) NT= non-toxic
ChemFinder
Leadscope
contained within
Figure 1. Multiple ways of analogue searching for seven phthalate esters
/Ρϑ .ΡΖ +0:3(
Figure 2. Plots of molecular weight (top) and hydrophobicity (bottom) for the compilation of
phthalates (first column) and representative structures for the HMWPE category (second column).
Mean symbol and median line are shown along with the min/max box and statistical outliers indicated with
an asterisk.
Figure 3. Distribution of logKow values for 324 phthalate esters
Figure 4. Plot of the first two principal components calculated from connectivity and information
indices.
Examples of outliers in the PCA plot (chemicals outside the ellipse in the lower left corner)
Examples of overlapping chemicals in the PCA plot (indicated with an arrow)
Figure 5. Examples of structures that have substantially different chemical features compared to the
rest of selected chemicals (outliers), and of very similar structures that are clustered together (very
close analogues).
Figure 6. Correlation between log toxicity values to fathead minnow predicted by ECOSAR and
TOPKAT. The lines are drawn according to regulatory cut-offs: 1 mg/L (solid line), 10 mg/L (dashed line),
and 100 mg/L (dotted line). The chemicals in the lower left corner are predicted to be very toxic by both
programs and the chemicals in the upper right corner are predicted to of no concern. Only chemicals
predicted within the TOPKAT optimum prediction space were used.
Figure 7. Parabolic relationship between logKow and toxicity. The function, observed by Hermens et al.
(8), was determined until log Kow of approximately 6. It was prolonged in this plot until 10 as a maximum
upper limit for measuring of log Kow.
Figure 8. Bilinear relationship between logKow and toxicity. The three reference lines indicate the
regions of the four toxicity categories: very toxic (VT) below 0; toxic (T) between 0 and 1; harmful (H)
between 1 and 2, and no concern (NC) above 2. The dashed line represents the logKow value, which
corresponds to the solubility limit of the phthalate esters. The circled chemicals are possible outliers due to
the possibility of acting by a more reactive mechanism.
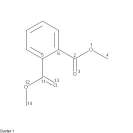
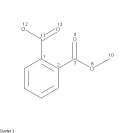
Signature
1) 79 predicted non-sensitisers
2) 27 predicted sensitisers
Figure 9. Substructural fingerprints for discriminating between sensitising and non-sensitising
phthalate esters
Log Kow (estimated): 0.12
Log Kow (estimated): 2.65
Log Kow (estimated): 5.22
1,2-benzenedicarboxylic acid, bis(2-
diethyl phthalate
diethyl 3,4,5,6-tetrachlorophthalate
hydroxyethyl) ester
1,2-Benzenedicarboxylic acid, bis(2-
ethoxyethyl) ester
Examples of possible trend breakers (should not be predicted by the ester model)
Allyl 2,3-epoxypropyl phthalate
Dimethyl 3,6-dihydroxyphthalate
1,2-Benzenedicarboxylic acid, 2-hydroxyethyl 2-[(1-oxo-2-propenyl)oxy]ethyl ester
Figure 10. Modifications in chemical structure can change the toxicity classification
Investigations of Read-across & Grouping, including mechanistic
Considerations and Rationales for Outliers
Rudolf Jäckh
Category approach: the weight of evidence and possible validation steps
Working with categories emerges from an iterative process of learning-by-doing, which could
identify remarkable similarities between structural elements and toxicological profiles on one hand,
whereas on the other hand it was also shown that a chemical category does not necessarily have to
be congruent with the category in a toxicological sense. Single compounds from the same chemical
category may in a number of cases exhibit different toxicological properties as well as
toxicologically very similar compounds may belong to quite various chemical categories.
The category approach presumes for the assessment of the toxicological properties of (a)
compound(s) not (fully) investigated a common mechanism of action which links the biological
activity to typical structural similarities and/or physiochemical properties. Assessments of
compounds via the category approach need a critical mass of empirical evidence and may, in
principle, be challenged by any meaningful experiment. If sufficiently established the category
approach allows interpolations and cross-reading of data, whereever the mechanism appears to be
understood. The derivation of a toxicological property purely on the basis of theory and similarity,
needs careful consideration and a sufficient amount of consensus. Any evidence or good reason that
militates against a proposed category or debates its size and limits should be welcomed and any
reasonable experimental engagement in single compound evaluation is regarded as a justifiable
option.
Chemical-toxicological categories often do not show the same structure-activity links for all
toxicological endpoints. As an example, branched chain carboxylic acids are very similar in many
aspects such as non-mutagenicity but may be highly variable in terms of prenatal toxicity.
Furthermore, not all structure activity postulates are substantiated by the same level of homogeneity
and validity, either on empirical or theoretical grounds; some may be more debatable than others
and in a number of cases additional experimental validation may be necessary.
Tentatively, it may be therefore differentiated between categories which are empirically and
mechanistically very firmly established and others which are in a more preliminary stage and might
profit from further collection of data or an (often limited) experimental challenge.
In the following, examples are proposed for such different levels:
1.)
Groups of compounds with typical molecule moieties or functional groups and a very monotone behaviour in a toxicological endpoint, with only minor or moderate quantitative shifts in activity according to common physicochemical descriptors such as chain length, molecular shape or physico-chemical parameters.
Such a pattern may be largely applied e.g. for many aliphatic hydrocarbons, aliphatic amines, nitriles, aldehydes, alcohols, side-chain-bearing non-heterocyclic aromates, at least for most endpoints. With an increased depth of knowledge and understanding of mechanisms, certain subcategories have emerged with specifities often in one endpoint such as the allergenicity of aliphatic amines with more than one amino function, the enhanced toxicity of --unsaturated aldehydes
-nitriles, the decreased toxicity of certain nitriles with specific -substitution patterns preventing metabolic activation (e.g. Proprionitrile vs. Methoxyproprionitrile or Tolunitriles) or the n-Hexane related neurotoxicity.
Groups of compounds which qualitatively and mechanistically form a common chemical-toxicological relation, however, with additional functional groups or structural elements that may cause them to act in a quantitatively variable manner. These quantitative aspects may be specifically considered and exploited in cross-readings, too, and either exempt compounds from further testing or require additional testing. (Examples are carboxylate or sulfate moieties in aromatic amines or nitro-compounds which practically inactivate the compound from Met-Hb formation. Also the non-carcinogenicity of Toluene vs. Benzene is based on such a carboxylate function resulting from the methyl substitution. Other substituents may increase lipophilicity and intracellular bioavailability. For non-polar compounds, such as benzene, a halogen may induce a dipole momentum and facilitate glutathione binding or hydrolysis, whereas halogenation may reverse such a trend).
In some cases a chemical category may typically show a very prominent potential in one single endpoint, such as extreme acute toxicity or corrosion. This may allow to neglect the consideration of other endpoints for practical reasons or to exclude the compound from further testing. In that case, a chemical category is deliberately not fully evaluated for all endpoints.
Chemical categories with a limited database or a doubtful degree of homogeneity in toxicological terms. Additional investigation may either corroborate or decline the category in the toxicological sense or create further subcategories for which theoretical/mechanistical explanations need to be worked out. This goal has been successfully achieved, e.g. for the category of glycol ethers which in their vast majority are innocious unless Methoxy-or Ethoxy acetic acid is formed in the course of metabolic transformation. In the case of glycol ethers, the categorical concept has, in fact, been confirmed via differentiation.
As a general caveat, no cross-reading should be done from ethyl to methyl, propyl- or methoxy substituents and vice versa for critical endpoints of systemic toxicity. Examples are Methanol vs. Ethanol, Ethylenglycol vs. Propylenglycol, e.g. Acetonitrile vs. Propionitrile, Methoxy-and Ethoxyacetic acid vs. Propoxyacetic acid; Butter yellow vs. its Ethyl-homologue, Ethylcarbamate vs. Methylcarbamate, Formaldehyde vs. Acetaldehyde.
Approaches to Chemical Categorization: An Illustrative Example of
Approaches Used by the Fragrance Industry
Tim Adams FEMA & Daniel Salvito RIFM
Approaches to Chemical Categorization: An Illustrative Example of Approaches Used by the
Fragrance Industry
Scope
This document is illustrative of the approaches used by the fragrance industry to categorize
chemicals for evaluation of human health and environmental endpoints. Specifically, it considers
an approach proposed by the fragrance industry for evaluating the systemic, reproductive,
developmental and genotoxic effects and environmental toxicity and fate of a group of 22
monoterpene primary alcohols and aldehydes and structurally related esters and acetals. Other
effects arising from topical exposure such as sensitisation or irritation or other routes (for example
via inhalation) are not considered, however similar criteria and considerations for chemical
categorization may also be applied to these and other toxicological endpoints.
Introduction
The safety evaluation of fragrance ingredients presents unique challenges principally due to the fact
that there are over 2,600 chemically defined fragrance ingredients and naturally occurring mixtures
(e.g., essential oils) in commerce, the vast majority of which are used at low levels. These
substances are used to produce a wide range of fragrances intended for use in cosmetics, fine
fragrances, household, personal care and other perfumed products. A majority of these substances
are used in food flavours and are present naturally in a traditional diet.
A large number of fragrance substances are found in plants as products of biosynthetic pathways
such as the isoprene and shikimic acid pathways and contain the same carbon skeleton backbone
and functionality, i.e. they are close structural relatives. Both aquatic and terrestrial animals have
evolved an array of biochemical processes to safely dispose of these naturally occurring substances
and structurally related analogs
Given the large number and commonality of fragrance substances, chemical grouping can
effectively be used to assess their hazards and risks.
For more than 40 years, the fragrance industry, through its human health and environmental safety
research institute (Research Institute for Fragrance Materials or RIFM) and its various trade associations,
has used chemical grouping and read across methods to objectively assess the safety of chemicals used as
fragrance ingredients in consumer products. These approaches have included grouping of chemicals in
order to assess their safety for consumer use in collaboration with the RIFM Expert Panel and compliance
with various international regulatory initiatives (e.g., US High Production Volume program and, in
preparation for REACH, the Strategic Partnership on REACH Testing). Flavours have also been assessed
using similar methodology to provide safety assessments for JECFA in support of, among other initiatives,
EU activities under FLAVIS and EFSA.
Despite encompassing more than 2,600 discrete chemicals, the majority of fragrance materials can be
classified into a relatively few basic structural groups which can be further subdivided based on structural
moiety most likely to be significant toxicologically and rendering the groups as similar as possible
between molecules by structural type (Bickers et al., 2003a). It is key to note that fragrance chemical
groups contain structurally related substances that participate in common metabolic pathways and exhibit
similar toxicologic potential. As an example, RIFM in consultation with the RIFM Expert Panel, has
recently published an assessment for the chemical group containing linalool and linalyl esters (Bickers et
al., 2003b) and three key cinnamyl fragrance ingredients (Bickers et al., 2005). Another example is the
US EPA High Production Volume Program, where essentially all fragrance chemicals encompassed by the
Program were organized by the fragrance industry into groups, which were accepted for evaluation by the
Criteria for Chemical Group Approach
A limited number of chemical groups exist for flavor/fragrance substances because approximately
90% of the chemicals are simple, low molecular weight substances derived from well-recognized
plant biosynthetic pathways consisting of only carbon, hydrogen and oxygen.
In order to apply the chemical group approach for fragrance ingredients, certain criteria can be
applied to identify members of a chemical group.
1) Members of the group should contain the same or homologous carbon skeletal backbone
2) Members of the group should contain common functional groups or functional groups
that participate in common biochemical pathways yielding metabolites that are of lower
toxic potential than the members of the chemical group. For example, a group may contain
esters, acetals, alcohols, aldehydes and carboxylic acids provided that data are available to
show that the esters and acetals rapidly hydrolyze in vivo to yield their corresponding
alcohols and aldehydes that subsequently the alcohols and aldehydes are efficiently oxidized
to the less toxic carboxylic acid derivatives. Systemic exposure levels should typically be
well below those required to saturate available detoxication pathways and thresholds of
toxicological concern.
3) Toxicity data among representative members of the group should show consistent effects
and similar toxic potential for the endpoint considered. For example, it is justifiable to
consider terpene hydrocarbons in a chemical group, since studies on representative members
of the group (limonene, myrcene, alpha-pinene, and camphene) show a common target
organ (kidney) and toxic levels within the same order of magnitude following systemic
Structural homologies allow hazard and risk issues to be considered for several materials within the
context of the information that exists for the representative members of the chemical group. In
many cases existing information for a structural group may obviate the need to submit a particular
individual substance to full toxicological testing and QSAR and expert judgment can be applied to
reduce uncertainty. In other cases, it may be necessary to test one or more particular members of a
structural class to obtain a more robust assessment of the class as a whole.
The application of these principles and criteria for the identification and organization of a chemical
group of fragrance materials is illustrated for a group of 22 monoterpene primary alcohols and
aldehydes and structurally related esters and acetals. The chemical group also includes one natural-
occurring complex mixture (lemongrass oil) whose main constituents are members of this chemical
group and account for the majority (>80%) of the mass of the natural but other natural substances
may also be relevant. The chemical grouping exercise is intended to demonstrate the type and extent
of data required to support the formation of a chemical group, and to provide the basis for assessing
the hazards and risks associated with use of members of the chemical group. An accompanying data
grid provides an overview of the available data for the monoterpene primary
alcohol/aldehyde/ester/acetal group (Attachment 1).
Chemical Group Approach
Monoterpene Primary Alcohols and Aldehydes and Related Esters and Acetals
Chemical substances
The twenty-two monoterpene alcohols, aldehydes esters and acetals are all derived from the same
branched-chain monoterpene C10 carbon skeleton (3,7-dimethyl-1-octyl) containing an oxygenated
function group on C1. The alcohols in this group are commonly recognized as geraniol, nerol and
citronellol. Structurally, they are either cis-trans isomers (nerol/geraniol) or a dihydro derivative
Although they contain different oxygenated functional groups at the terminal carbon, rapid
hydrolysis in animals converts the esters and acetals into the corresponding alcohols and aldehydes
that then participate in the common metabolic pathways of detoxication (see Figure 1). Differences
in structure among members of the group include: 1) the length of the aliphatic chain in the
carboxylic acid component of the ester or alcohol component of the acetal, 2) the length and
position of one of the alkyl substituents in the monoterpene, and 3) the presence or absence of
unsaturation within the monoterpene carbon skeleton. Although the latter difference may seem
important if the unsaturation involves an alpha,beta-unsaturated aldehyde, metabolic data exist to
show that these substances do not efficiently conjugate with glutathione and, therefore, are not
associated with the systemic toxic effects due to cellular glutathione depletion and oxidative stress
(Diliberto et al., 1990; Parke and Rahman, 1969; Boyer and Petersen, 1990; Chadha and
Madyastha, 1982; NTP, 991)
Naturally-occurring botanical mixture of variable composition
In addition to chemically-defined substances, essential oils (botanical UVCB's) derived from plants
are important fragrance materials. Although the composition is variable, the variability is limited by
the fact that key constituents must be present at sufficient concentrations to exert the required
fragrance effect. Hence, citronella oil and lemongrass oil are effective as fragrances only when they
contain a complex mixture containing mainly monoterpene primary alcohols, aldehydes and related
esters. Greater than 80% of commercial West or East Indian types of lemongrass oil is composed of
a mixture of monoterpene branched-chain primary alcohols (geraniol, nerol, citronellol), aldehydes
(citral as a mixture of geranial and neral), acids, and related esters (geranyl acetate). The majority
of the remaining substances are terpene hydrocarbons (e.g., limonene and myrcene) that exhibit a
toxic potential similar to that of the members of this chemical group. Therefore, it is not
unreasonable to conclude that metabolism and toxicity data representative of the chemical group is
also representative of a mixture of the chemical group, i.e., the essential oil.
Categorization Criteria
In order to qualify for membership in the chemical group, members must meet the aforementioned
criteria. Substances must not only show similar biochemical fate, but the toxic potential for
representative members of the group (esters, alcohols, acetals and aldehydes) should be consistent
thereby defining the applicability domain and boundary substances for the different endpoints
The discussion below attempts to evaluate the consistency and strength of the data within the
category in this context.
Hydrolysis of monoterpene esters and acetals and metabolism of monoterpene alcohols and
aldehydes
Monoterpene esters have been demonstrated to hydrolyse to the corresponding terpene alcohol in
both terrestrial and aquatic species such as fish and subsequently the alcohols are metabolized to the
corresponding aldehydes, which are then converted into polar excretable products (Diliberto et al.,
1990; Ishida et al., 1989). Acetals are also hydrolysed to aldehydes that enter the same pathway
(Morgareidge, 1962; Vicchio et al., 1989).
Hydrolysis data are available for a number of geranyl and citronellyl esters in vitro in gastric juice
and intestinal fluid (Grundschober, 1977; Hall, 1979), in intestinal and mucosal preparations, rat
liver, and in rat and human blood. Hydrolytic activity is greatest in the liver (half-lives normally in
the order of a few minutes) (Buck and Renwick, 1998). These data would support the rapid
conversion of the esters to the alcohols in vivo in terrestrial species including humans. The
presence of carboxylesterase isozymes in aquatic species (liver, whole body, etc.) and relative rates
of ester hydrolysis in a variety of fish and mammals (Barron et al., 1999; Li and Fan, 1997) support
the conclusion that hydrolysis of esters also occurs in aquatic species. These conclusions could be
further supported for example by collecting additional in vitro hydrolysis data with geranyl and
citronellyl esters in fish and mammal liver homogenates. Hydrolysis of acetals is even more rapid
than that of esters. In vitro experiments on simple aliphatic acetals could be supplemented with
hydrolysis data in rat and fish tissues.
Following hydrolysis of the esters, the resulting primary monoterpene alcohols such as geraniol,
nerol, rhodinol and citronellol undergo alcohol oxidation to yield the corresponding aldehyde
(Diliberto et al., 1990; Ishida et al., 1989). These reactions are catalyzed by alcohol dehydrogenase
and other high capacity oxidative enzymes. Also, these terpenes are subject to CYP450 -catalyzed
omega-oxidation of the alkyl substituents to yield 8-hydroxy derivatives that undergo subsequent
functional group oxidation to yield polar dicarboxylic acid derivatives (Hildebrandt's acid)( Parke
and Rahman, 1969; Boyer and Petersen, 1990; Chadha and Madyastha, 1982). To a minor extent,
hydration and selective hydrogenation also occur (Figure 2). These polar metabolites are rapidly
excreted primarily in the urine of animals. Alternately, the corresponding carboxylic acids formed
by oxidation of the alcohol function may enter the beta-oxidation pathway and eventually undergo
cleavage to yield shorter chain carboxylic acids that are completely metabolized to carbon dioxide
and water in the fatty acid pathway and tricarboxylic acid cycle. The enzyme systems available to
catalyze these reactions have also been characterized in fish and other aquatic species supporting
the conclusion that the esters and acetals are hydrolyzed to alcohols and aldehydes in aquatic
species and then oxidized to even more polar products that are not bioaccumulative.
Metabolism of Nerol, Geraniol, and Citral in Rats
Alcohol Dehydrogenase
Physiochemical Properties
Although all physiochemical properties are to be provided in REACH, selected key physical
properties discussed here include vapor pressure and log Kow. Values of vapor pressure are on the
same order of magnitude for the alcohols, aldehydes, acetals and for formate esters and an order of
magnitude greater than those for other higher MW esters.
Although key physiochemical properties of esters and acetals, such as vapor pressure and log Kow,
show consistent increases with increasing molecular weight of the non-terpene carboxylic acid or
alcohol component, respectively, data supporting ready hydrolysis argues that esters and acetals in
the group are present in vivo as the corresponding monoterpene alcohols and aldehydes. Therefore,
although differences in physiochemical properties exist between the esters and alcohols in this
group, metabolic fate and toxic potential of the esters and alcohols are similar.
Although model predictions of physiochemical properties can be used, the collection of
experimental log Kow and water solubility data for a representative aldehyde and saturated alcohol
(for example tetrahydrogeraniol) would provide a more comprehensive evaluation of log Kow and
solubility within the group and would allow better extrapolation of experimental ecotoxicity data to
physiochemical-based model data.
Categories for Environmental Endpoints
The application of grouping concepts to the formation of chemical categories for environmental
endpoints for fragrance materials can be performed by reviewing the physical-chemical properties,
metabolic pathways and ecotoxicological mode of action. For example, trends for fate parameters
can be established following consideration of the octanol-water partitioning coefficient and water
solubility as well as an understanding of the metabolism in organisms of interest (e.g., fish for
bioaccumulation). Mode of action (Verhaar et al. 1992; Russom et al. 1997) can be useful in
assigning sub-categories (e.g., polar versus non-polar narcotics) to better establish trends in the
hazard data. These concepts can be extended to the review of related UVCBs using their major
constituents as markers.
Environmental fate
Biodegradation data for unpublished reports using standardized OECD guideline protocols clearly
demonstrate that the esters, alcohols, acetals and aldehydes are readily and ultimately
biodegradable, although some reduced biodegradability was noted for the acetals. Static fugacity
model-based calculations for persistence in the environment show differences among members of
the group. The significance of these calculations must be evaluated in the context that the
substances in this chemical group are also products of plant biosynthesis and are, therefore,
ubiquitous in the environment. Experimentally, members of the group have been shown to be
readily and/or ultimately biodegradable, and the remainder would be expected to behave similarly
in the environment. These models do not account for the influence of biogenic production on
partitioning in the environment nor do they account for the hydrolysis of these substances under
environmental conditions. In light of the significant impact of natural production of the terpenes and
hydrolytic activity, relevance of fugacity calculations for this chemical group must be carefully
The existing data, supplemented with established QSAR approaches, should provide justification to
limit the need for further testing.
Aquatic toxicity endpoints
Experimental ecotoxicity data derived from OECD or EEC guideline studies show LC50 values in
the range from 5-15 mg/l for an ester, three alcohols and an aldehyde in the group (Attachment 1).
Given the volume of production, structure (alpha-beta-unsaturated aldehyde) and key metabolic role
of citral in the chemical group, an OECD guideline fish acute study could be performed to better
evaluate the range of acute toxicity for the different metabolic products formed from various
members of the group. For aquatic invertebrates, experimental EC50 data for Daphnia with an
ester, two alcohols and an acetal could be supplemented with an acute study for citral. Esters could
be placed in a subcategory and additional OECD studies could be performed for low and high MW
esters. Similar arguments can be made for aquatic plants.
Bioaccumulation potential from longer-term exposure of aquatic species to monoterpene substances
in this group needs to be addressed in a scientifically rigorous manner. First, data on the hydrolysis
of esters and acetals in aquatic species (fish liver, etc.) should be collected to show that more
lipophilic members of the group are readily converted into more polar metabolites (alcohols and
aldehydes) that can be further oxidized and excreted or completely metabolized. Although data
exists to show that invertebrates have the enzymatic capacity to hydrolyse and oxidize these
substances, additional experimental data would need to demonstrate that these biochemical changes
occur upon exposure to low levels of these substances. These types of biochemical data would focus
testing requirements for chronic ecotoxicity studies on those members of the group that are
indicated to bioaccumulate.
Human health endpoints
Acute Toxicity
Consistent acute mammalian toxic potential via both the oral and dermal route are demonstrated by
LD50 values in the range from 4300 -6300 mg/kg with a majority of values >5000 mg/kg. These
data are consistent with the conclusion that esters and acetals are rapidly hydrolysed to the
corresponding alcohols and aldehydes, and that there is sufficient data to support the category.
Sensitization
Some of the materials in this group, particularly the aldehydes, have the potential to cause dermal
sensitization. However, they can be formulated into consumer products at safe levels. Based on the
chemical, cellular and molecular understanding of dermal sensitization, it is possible to conduct an
exposure-based Quantitative Risk Assessment (QRA) to determine safe use levels of fragrance
ingredients in a variety of consumer product types.
Genotoxicity
In vitro genotoxicity data must always be evaluated in the context of existing in vivo data and the
results of two-year carcinogenicity bioassays. Only geranyl acetate shows consistent evidence of
mutagenicity in vitro. Geranyl acetate produces an increase in mutational frequency in the mouse
lymphoma forward mutation assay (MLA). Other in vitro assays are uniformly negative. MLA
results for these types of substances are now recognized as false positives that are caused by the
formation of organic acids in metabolically competent cell lines (Brusick, 1986). These acids alter
cellular buffering capacity and cellular osmolality. Based on the observation that other in vitro
assays and the two in vivo assays (UDS and mouse micronucleus) are negative and geranyl acetate
is not carcinogenic in either rats or mice in a 2-yr bioassay, the MLA data can be confidently
classified as a false positive. In a similar manner, isolated evidence of increased chromosomal
aberrations for citronellal and sister chromatid exchange for citral were obtained in non-standard
assays performed at longer incubation times and at near toxic concentrations (Kasamaki et al.,
1982; NTP, 2001). QSAR assessment could provide direction in defining boundary substances or
the need to confirm via further testing. In addition, if related esters and acetals such as geranyl
acetate and citral diethyl acetal can be demonstrated to hydrolyze under the in vivo conditions used
in the micronucleus test, additional support for the low genotoxic potential of the category could be
Short-term and long-term toxicity
Key repeat dose studies exist for representative esters, acetals, alcohols and aldehydes in this
chemical group. 90-day and 2-yr (NTP) studies in mice and rats for geranyl acetate/citronellyl
acetate, citral diethyl acetal, geraniol, citronellol, and the mixture of geranial and neral commonly
recognized as citral (see Attachment 1) are available. The slightly lower NOAELs (345 vs 1000
mg/kg in the 90-day and 210 vs 2000 mg/kg) for citral versus geranyl acetate/citronellyl acetate can
be understood in terms of the known palatability issue and bodyweight reduction related to
administering high dietary levels of aldehydes (citral). Disregarding the bodyweight effect, the
NOAELs for citral and the ester are surprising similar. Importantly, neither substance showed any
significant evidence of carcinogenicity in the 2-yr assay. Key 90-day and 28-day data for a
homologue of citronellal (melonal, 2,6-dimethyl-5-heptenal) provide additional data to assess the
hazard potential of the category. The results of 90- and 28-day studies for citronellol, geraniol and
citral diethyl acetal confirm the toxic endpoints and potential of the category. It would be important
that repeat dose histopathology data on sex organs be provided from all new studies in order to
properly evaluate the results of reproductive studies with members of the chemical group and
mitigate the need for additional reproductive studies (see below).
Reproductive and developmental toxicity endpoints
Current data to evaluate the reproductive toxicity potential of the chemical group consist of two
studies for citral that support NOAELs in the range of 50-60 mg/kg. Other screening
reproductive/developmental toxicity data for citral diethyl acetal and melonal (2,6-dimethyl-5-
heptenal) support maternal NOAELs in a similar range (125-300 mg/kg). Note that repeat dose
studies, both short and long-term studies, for geranyl/citronellyl acetate, melonal and citral show no
evidence of changes to the reproductive organs of males or females. Studies for boundary
substances (geranyl acetate and citral) could be supported, for example, by screening studies for the
acetal and melonal. Such screening reproductive/developmental toxicity studies may be considered
rigorous for hazard evaluation purposes, if results are consistent with those of more rigorous studies
and QSAR assessment.
Data to measure developmental potential is similar to that for the reproductive endpoint. Data for
two studies on citral is supported by screening data from the acetal and melanal. Developmental
NOAELs from these four studies are on the same order of magnitude. QSAR and metabolic profiles
may suggest the need for further testing based on identification of boundary substances
Concluding Remarks
Experimental data on the metabolic fate and toxic potential for various endpoints for representative
members of the group support the following conclusions:
the esters and acetals in this chemical group are readily converted to the
corresponding alcohols and aldehydes, respectively, in this group.
all substances in this group and the constituents of the naturally occurring mixture
in this group participate in common metabolic pathways of detoxication.
the physiochemical properties of members and hydrolysis products are consistent
and based on model calculations and experimental biodegradation data that
indicate a lack of persistence for members of the group in the environment.
acute ecotoxicity data show a similar acute toxic potential for the esters, alcohols
and aldehydes. Hydrolysis data in aquatic species would support the conclusion
that these naturally-occurring substances do not bioaccumulate.
evaluation of toxicity enables clear similarities to be determined within the
category. Further assessment of the data and use of QSAR will provide
justification for the need to perform additional testing.
Using this approach one can identify a limited number of additional studies to strengthen the read-
across structure of the chemical group hazard assessment. Data currently available present strong
evidence that the hazard potential of a single member can be adequately assessed in the context of
the available data for the chemical group.
Barron M.G., Charron K.A., Stott W. T. and Duvall S.E. (1999) Tissue carboxylesterase activity of
rainbow trout. Environmental Toxicology and Chemistry, 18, 2506-2511.
Bickers D.R., Calow P., Greim H.A., Hanifin J.M., Rogers A.E., Saurat J.H, Sipes I.G., Smith R.L.
and Tagami H. (2003a) The safety assessment of fragrance materials. Regulatory Toxicology and
Pharmacology, 37, 218-273.
Bickers D.R., Calow P., Greim H.A., Hanifin J.M., Rogers A.E., Saurat J.H, Sipes I.G., Smith R.L.
and Tagami H. (2003b) A toxicologic abd dermatologic assessment of linalool and related esters
when used as fragrance ingredients. Food and Chemical Toxicolgy, 41(7), 919-942.
Bickers D.R., Calow P., Greim H.A., Hanifin J.M., Rogers A.E., Saurat J.H, Sipes I.G., Smith R.L.
and Tagami H. (2003b) A toxicologic abd dermatologic assessment of cinnamyl alcohol,
cinnamaldehyde and cinnamic acid when used as fragrance ingredients. Food and Chemical
Toxicolgy, 43(6), 799-836.
Boyer C.S. and Petersen D.R. (1990) The metabolism of 3,7-dimethyl-2,6-octadienal (Citral) in rat
hepatic mitochondrial and cytosolic fractions. Drug Metabolism and Disposition, 18, 81-86.
Brusick D. J. (1986) Genotoxic effects in cultured mammalian cells produced by low pH treatment
conditions and increased ion concentrations. Environmental Mutagenesis 8, 879-886.
Buck N. R. and Renwick, A. G. (1998) Report on the behaviour of linalool in artificial gastric and pancreatic juices. Unpublished report to FEMA.
Chadha A. and Madyastha K.M. (1984) Metabolism of geraniol and linalool in the rat and effects
on liver and lung microsomal enzymes. Xenobiotica, 14(5), 365-374.
Diliberto J.J., Srinivas P., Overstreet D., Usha G., Burka L.T. and Birnbaum L.S. (1990)
Metabolism of citral, an ,-unsaturated aldehyde, in male F344 rats. Drug Metabolism and
Disposition, 18, No. 6 866-875.
Grundschober F. (1977) Toxicological assessment of flavoring esters. Toxicology 8, 387.
Hall R.L. (1979) McCormick and Company, Inc. Private communication to FEMA.
Ishidate M. Jr., Sofuni T., Yoshikawa K., Hayashi M. Nohmi T., Sawada M. and Matsouka A.
(1984) Primary mutagenicity screening of food additives currently used in Japan. Food and
Chemical Toxicology, 22(8), 623-636.
Kasamaki A., Takahashi H., Tsumura N. Niwa J., Fujita T. and Urasawa S. (1982) Genotoxicity of
flavoring agents. Mutation Research, 105, 387-392.
Li S.N. and Fan D.F. (1997) Activity of esterases from different tissues of freshwater fish and
responses of their iosenzymes to inhibitors. J. Toxicology and Environmental Health, 2, 149-157.
Morgareidge K. (1962) In vitro digestion of four acetals. Food and Drug Research Laboratories. Unpublished report to the Flavor and Extract Manufacturers' Association. NTP draft (2001) National Toxicology Program. Toxicology and carcinogenesis studies of citral (microencapsulated) (CAS No. 5392-40-5) in F344/N rats and B6C3F1 Mice.(Feed
studies)Technical Report Series 505 NIH Publication No. 01-4439. U.S. Department of Health and
Human Services, Public Health Service, National Insitutes of Health.
Parke D.V. and Rahman H. (1969) The effects of some terpenoids and other dietary nutrients on
hepatic drug-metabolizing enzymes. Biochemical Journal, 113(2), 12P.
Russom C.L., Bradbury S.P., Broderius S.J., Hammermeister D.E. and Drummond RA. (1997)
Predicting modes of toxic action from chemical structure: acute toxicity in the fathead minnow
(Pimephales promelas). Environ. Tox. Chem., 16, 948-967.
Verhaar H.J.M., vanLeeuwen C.J. and.Hermens J.L.M. (1992) Classifying environmental
pollutants. 1. Structure-activity relationships for prediction of aquatic toxicity. Chemosphere, 25(4),
471-491.
Vicchio D. and Callery P.S. (1989) Metabolic conversion of 2-propylpentanal acetals to valproic
acid in vitro. Drug Metab. Dispos., 17, 513.
Appendix: DATA GRID for Monoterpene Primary Alcohols and Aldehydes and Related Esters and Acetals
Summary of Key Data for Terpene primary alcohols/aldehydes/acids/esters/acetals- Category 2
Substance
citronellyl & geranyl
citronellol/R and l-
geraniol/
citral dimethyl
citronellal
geranial/
neryl acetate
diethyl acetal
bergamal
citronellyl propionate
geranyl crotonate
Structure
Alcohols
Alcohols
Aldehydes
Aldehydes
citronellol, (+/-), R-, & l-
aldehydes
R=H formate (CF)
acetal (CMA)and (TG)
R=CH3 acetate (CA)
R=C4H7 tiglate (CT)
R= C3H7 propionate (CP)
R= C3H5 crotonate (CC)
R=H formate (GF) R=CH3 acetate (GA)
R=C4H7 tiglate (GT)
tetrahydrogeraniol (TG)
R=CH3 acetate-NA
Substance
citronellyl & geranyl
citronellol/R and l-
geraniol/
citral dimethyl
citronellal
geranial/
neryl acetate
diethyl acetal
bergamal
citronellyl propionate
geranyl crotonate
C-106-22-9/ 106-23-0/
CAS #
EO- 26330-65-4 /
CC-244-019-4 GF-203-339-4 GA-203-341-5 GT-232-078-9 NA-205-459-2
Metabolic
Hydrolysis to alcohols in
Oxidation of alc & omega
Oxidation of alc &
Same metabolites as
vitro intestinal fluids/rat
citral and alcohol
geraniol/nerol and
liver/rat blood/human blood
diacid- glutathione
analogous to those
(Barron, Renwick, etc.)
conj. Of bergamal
Hildebrandt's acid
Substance
citronellyl & geranyl
citronellol/R and l-
geraniol/
citral dimethyl
citronellal
geranial/
neryl acetate
diethyl acetal
bergamal
citronellyl propionate
geranyl crotonate
/0.2 mm Hg (calc)
0.07 mm Hg (FMA)
Pressure
(Vuilleumier, 1995a):0.009
CC 0.0063 GF 0.05 GA 0.03 GT 0.001 NA 0.03 (FMA)
C-Log KOW (calc)=3.56
(Syracuse Research Corp)
(calc)=3.45 (Syracuse
C-Log KOW (meas)=3.1
se Research Corp
(Givaudan-Roure, 1991g)
(Syracuse Research
NA 4.48 (Syracuse Research Corp)
Water Sol.
C-300 (BBA, 1990); 105
(SRA)/27.56 (calc)
CC 0.919 GF 23.7 GA 18.2 GT 0.319 NA 18.2 (EPIWIN)
Substance
citronellyl & geranyl
citronellol/R and l-
geraniol/
citral dimethyl
citronellal
geranial/
neryl acetate
diethyl acetal
bergamal
citronellyl propionate
geranyl crotonate
Biodegrad
GA Biodegradation by day
C-Biodegradation by day
Biodegradation by
28 was 82.2 % (Birch,
28 was 100%. (BBA,1990)
biodegradable in
99.5%(BBA, 1990)
CA 82.1% at 28 days in
OECD 301B (Quest, 1995)
C-63.8% by MITI test in 28
28 days. Secondary
days mg/l (Givaudan-
effluent from an
99.1% biodegraded within
activated sludge plant
28 days in OECD 301B
was used. Readily
>60% degraded within 10
days readily biodegradable
(80-90% in 28 days) in
OECD 301F ( BASF, 1988)
biodegradable
(H&R, 2001)
N-/ 92% after
28 days (81%
at 10 days) in
301F Giv,
1999); 85.9%
after 28 days in
301B (Quest,
1994)
Acute Tox
GA 96 hr LC50=6.12 mg/L C-10-22 mg/l (BASF,
4.5 mg/l (ECOSAR)
Fish, 96 hr
in rainbow trout (Ward,
LC50(mg/l)
3.9 mg/l (ECOSAR) /
TG- 5 mg/l-no effects at 24
hrs for trout, sunfish,
bluegill (EPA, 1987)
bluegill (EPA, 1987)
GA 62 mg/l (Grade, 1994)
C-2.38 mg/l (BASF, 1990)
1.29 mg/l /0.279
plants 48 hr
Substance
citronellyl & geranyl
citronellol/R and l-
geraniol/
citral dimethyl
citronellal
geranial/
neryl acetate
diethyl acetal
bergamal
citronellyl propionate
geranyl crotonate
EC50 (mg/l)
Substance
citronellyl & geranyl
citronellol/R and l-
geraniol/
citral dimethyl
citronellal
geranial/
neryl acetate
diethyl acetal
bergamal
citronellyl propionate
geranyl crotonate
GA 10 mg/l (Grade, 1994)
C-48 EC50=17.48 for dl-
1.96 mg/l /0.489
citronellol in Tween 80 (EU (Ward 2003)/
Inverts 96
Directive 79/831) (BASF,
mg/L (OECD 202) (Quest, 1995)
Acute Tox
Rat LD50 in mg/kg
C-Rat oral LD50= 3450
Rat oral >5000
Rat gavage LD50=
CF 8400 (Calandra, 1971)
mg/kg (Moreno,1973)
CA>5000 (Calandra, 1971)
CT>5000 (Moreno, 1978)
CP >5000 (Moreno, 1973)
CC >5000 (Moreno, 1975)
GF >5000 (Wier, 1971)
GA 6330 (Jenner, 1964)
GT >5000(Slanski, 1972)
NA >5000 (Moreno, 1978)
Acute Tox
CF>2000 (Calandra, 1971)
C-Rabbit dermal LD50 =
(Dermal)
CA>5000 (Calandra, 1971)
2650 mg/kg (Moreno,1973)
CT>5000 (Moreno, 1978)
CP >5000 (Moreno, 1973)
CC >5000 (Moreno, 1975)
GF >5000 (Wier, 1971)
dermal LD50 >
GT >5000(Slanski, 1972)
NA >5000 (Moreno, 1978)
Rabbit dermal LD50
> 5000 mg/kg
(Moreno,1976)
Substance
citronellyl & geranyl
citronellol/R and l-
geraniol/
citral dimethyl
citronellal
geranial/
neryl acetate
diethyl acetal
bergamal
citronellyl propionate
geranyl crotonate
Acute Tox
C-Mouse. inhalation ED25
= 990 ug/l (Troy,1977)
ED25 = 570 ug/l; (Troy,1977)/ N-Mouse inhalation ED25 = 590 ug/l; (Troy,1977)
In vivo
C-Rat gavage 100 µl no
Mouse micronucleus-
(Mirsalis et al., 1983)
effects, Ames assay 100
250 & 1000 mg/kg ip
(NTP, 2003) Mouse (blood) 14 week study- no increase in PCE (NTP, 2003)
GA 450, 900, 1800 mg/kg
ip, in mouse micronucleus- assay negative (Shelby, 1993)
Substance
citronellyl & geranyl
citronellol/R and l-
geraniol/
citral dimethyl
citronellal
geranial/
neryl acetate
diethyl acetal
bergamal
citronellyl propionate
geranyl crotonate
C-Rec assay 17 µg/disk B.
/Ames: neg (up to
mutation assay, 1-20
subtilis: negative (Oda et
500 ug/plate) (IFF,
(Eder,1982a) ; Ames
ul/plate =negative (Heck,
–negative (NTP,
1989) :Ames up to 3333
2001 Ames: neg (up
ug/plate –neg (Tennant,
to 700 ug/plate)
1987): Ames reverse
ug/plate (Zeiger,
1998); Ames (Gomez-Carneiro,
1998); Ames: neg
(Mortelmans, 1986)
GA- MLA assay up to 125
nl/ml-Pos (Lorillard, 1987);
GA-ABS assay in CHO –
neg (Lorillard, 1983)
GA UDS in rat hepatocytes
from Male F344 rats neg-
(Mirsalis, 1983);
GA ABS assay 50-150
ul/ml (Galloway, 1987)
GA – MLA –positive w/o
act (Heck, 1989) (false
GA MLA –pos w/o act
GF-Bac forward mutation
assay 18 ul-neg (Oda, 1978)
aberrations (ABS)
0.03 mg/ml in Chinese hamster fibroblasts w/o act: negative (Ishidate et al., 1984) No ABS for mice and rats in CHO cells (NTP, 2003)
SCE in CHO cells w
& w/o act. –pos
Substance
citronellyl & geranyl
citronellol/R and l-
geraniol/
citral dimethyl
citronellal
geranial/
neryl acetate
diethyl acetal
bergamal
citronellyl propionate
geranyl crotonate
subtilus at 17 ug/ml –
neg. (Oda, 1978)
assay in E. coli up to
(Ishidate et al.,
100 ug/plate – neg
negative (Eder,1982a)
mg: negative (Ishidate,1984)
Substance
citronellyl & geranyl
citronellol/R and l-
geraniol/
citral dimethyl
citronellal
geranial/
neryl acetate
diethyl acetal
bergamal
citronellyl propionate
geranyl crotonate
13 wks rats-NOAEL-2000
C-12-week- rats fed 50
Mixture of citral
13-weeks rats fed
mg/kg bw/d NTP, 1987
mg/kg/d in diet. No
and citral diethyl
1000 ppm, 2500 ppm,
(Test material-mixture of
treatment related effects
geranyl and citronellyl
(Trubek Labs, 1958i).
effects. (Hagan,1967)
mg/kg/d in diet.
13 wks rats-NOAEL 1000
90-day, rat, diet 820
mg/kg bw/d (NTP, 1987)
(M), 675 (F) mg/kg
reduced bw due to
palatability problem.
NOAEL 345(M) and
335 (F) (NTP, 2003)
90-day, mouse, diet
745 (M), 790 (F)
mg/kg reduced bw due to apparent early palatability problem. 3915(M) and 3870 (F) thickening of forestomach-hyperkeratosis (NTP, 2003)
103 wks mice NOAEL-
104 wks diet, mice
1000 mg/kg bw/d (NTP,
103 wks rats-NOAEL2000
mg/kg bw/d NTP, 1987
NOAEL=210 mg/kg bw/d NTP, 2001
Substance
citronellyl & geranyl
citronellol/R and l-
geraniol/
citral dimethyl
citronellal
geranial/
neryl acetate
diethyl acetal
bergamal
citronellyl propionate
geranyl crotonate
No effects to reproductive
Oral rat, 60, 250, 500
organs in 90-day or 2yr
or 1000 mg/kg/d on
studies with geranyl and
gestation days 6-15
citronellyl acetate mixture
in mice and rats (NTP,
toxicity based on
fertility index,
gestation length
sites, and litter
size (Vollmuth et
(Vollmuth et al., 1995) No organ wt or histopathological effects to reproductive organs in 28-d (Terrill, 1991) and 90-d studies (Gaunt, 1983)
Oral rat. 0, 50, 160 or
Substance
citronellyl & geranyl
citronellol/R and l-
geraniol/
citral dimethyl
citronellal
geranial/
neryl acetate
diethyl acetal
bergamal
citronellyl propionate
geranyl crotonate
500 mg/kg/day 2 wk prior to mating & through day 20 of gestation. 50 mg/kg maternal NOEL maternal no adverse effect level. 160 mg/kg fetal/pup NOEL (Hoberman,1989)
maternal or fetal
effects), 85 ppm
maternal toxicity-
mg/kg bw/d fetal
teratogenic, (York,
(Vollmuth et al.,
Rat oral <60 mg/kg
bw/d maternal, <60
mg/kg bw/d fetal
(Cristina, 1995)
Ip, rat, 360 mg/kg given on days 10-12 of gestation –no effects (Abramovici, 1980)
Pag. 1 ----> Pag. 180
European Commission
EUR 22481 EN – DG Joint Research Centre, Institute IHCP
Title: A compendium of case studies that helped shape the REACH guidance on chemical categories and
read across
Authors: Andrew Worth and Grace Patlewicz
Luxembourg: Office for Official Publications of the European Communities
2007 – 180 pp. – 21 x 29.7 cm
EUR - Scientific and Technical Research series; ISSN 1018-5593
This document is a compendium of case studies that were conducted as part of REACH Implementation Project (RIP) 3.3-2, which was charged with developing technical guidance on the use of chemical grouping approaches (chemical categories and read-across methods). The lessons and insights from each of the case studies helped to shape the technical content captured in the resulting guidance document. The case studies are presented in their original form. For ease of reference, they are grouped into two themes: current and prospective experiences in the formation and/or use of category approaches.
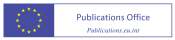
The mission of the JRC is to provide customer-driven scientific and technical support for the conception, development, implementation and monitoring of EU policies. As a service of the European Commission, the JRC functions as a reference centre of science and technology for the Union. Close to the policy-making process, it serves the common interest of the Member States, while being independent of special interests, whether private or national.
Source: http://reach.reach.gov.pl/media/file/Publikacje%20UE-REACH/LBNA22481ENC_002-1.pdf
February 29, 2016 WSPC Proceedings - 9.75in x 6.5in Recent results and perspectives on cosmology and fundamental physics from microwave surveys∗ Carlo Burigana,1,2,3,a Elia Stefano Battistelli,4,b Micol Benetti,5,c Giovanni Cabass,4,d Paolo De Bernardis,4,e Sperello Di Serego Alighieri,6,f Eleonora Di Valentino,7,g Martina Gerbino,8,9,4,h Elena Giusarma,4,i Alessandro Gruppuso,1,3,j
[Boletín de Infraestructuras de Burgos] [Cuarto trimestre 2011] Boletín de Infraestructuras de Burgos [ASOCIACIÓN PLAN ESTRATÉGICO DE BURGOS] [En este número] Ha pasado un año desde la publicación del último boletín. En este tiempo, la situación económica del país no ha permitido grandes novedades en el estado de la construcción de nuevas infraestructuras, salvo en la apertura