Usf_beitrag.pdf
Beiträge des Instituts für Umweltsystemforschung
der Universität Osnabrück
Herausgeber: Prof. Dr. Michael Matthies
Beitrag Nr. 50
Chemical Fate of Sulfadiazine in Soil:
Mechanisms and Modelling Approaches
Christiane Zarfl
November 2008
ISSN Nr. 1433-3805
Prof. Dr. Michael Matthies Universität Osnabrück Institut für Umweltsystemforschung Barbarastr. 12
D-49069 Osnabrück
0541/ 969 - 2576/2575
0541/ 969 - 2599
USF - Institut für Umweltsystemforschung, Universität Osnabrück
This publication contains the doctoral thesis of Christiane Zarfl, entitled Chemical
Fate of Sulfadiazine in Soil: Mechanisms and Modelling Approaches and accepted by
the Department of Mathematics & Computer Science of the University of Osnabr¨
in June 2008.
Die vorliegende Publikation enth¨alt die vom Fachbereich Mathematik/Informatik
der Universit¨at Osnabr¨
uck im Juni 2008 angenommene Dissertation von Christiane
Zarfl mit dem Titel Chemical Fate of Sulfadiazine in Soil: Mechanisms and Modelling
Published by Shaker Verlag, Aachen, 2008.
Having finished the scientific part of my thesis it is a great pleasure to take the
opportunity to thank all those who accompanied and supported me along the way.
First of all, I would like to express my appreciation and gratitude to Prof. Michael
Matthies and Dr. J¨org Klasmeier for giving me the possibility to conduct this thesis
at the Institute of Environmental Systems Research and especially for their encour-
agement, inspiration and continuous interest in the results of my research. I also
thank Andreas Focks for many helpful discussions and assistance on our way into
the scientific world.
This thesis would not have been possible without the cooperation and support of
my colleagues of the DFG research group. Thanks for great experimental work, data
sets and fruitful meetings, particularly to Markus F¨orster, Dr. Volker Laabs and
Prof. Wulf Amelung (University of Bonn), J¨
urgen Ebert and Dr. Burkhard Schmidt
(University of Aachen), as well as Dr. Marc Lamsh¨oft and Dr. Sebastian Z¨
(University of Dortmund). I am especially indebted to Dr. Wolfgang Tappe and Dr.
Joost Groeneweg (Research Centre J¨
ulich) for open minded, cheerful discussions
and for working hand in hand to successfully integrate effect data and mathemati-
cal modelling. For several intensive and progressive workshops bringing together the
experts on veterinary antibiotics (including both environmental fate and effects) I
would like to thank Dr. Heike Schmitt, Dr. Christian Stamm, Dr. Maren Kahle, Prof.
S¨oren Thiele-Bruhn, Dr. Marc-Oliver Aust and Ute Hammesfahr, Anja Kotzerke and
Nadine Kurowski. Thanks to Saskia Zimmermann and Dr. Anne Wehrhan for mak-
ing the knowledge and results on sulfonamides of their diploma and PhD thesis,
I am grateful to Dr. Gertje Czub for providing her model on bioaccumulation and for
cooperation in research areas beyond the contents of my thesis. I would like to thank
her as well as Prof. Michael McLachlan and Dr. Knut Breivik for accompanying me
on my first conference trip across the Atlantic Ocean.
I thank my colleagues from the Institute of Environmental Systems Research for
creating such an excellent working atmosphere, for assistance, encouragement and
shared and often also sporty activities. Thanks to Dr. Gisela Danz for her interest
in my work and for helpful advice.
This thesis also owes its existence to financial support: I would like to thank the Ger-
man Research Foundation (DFG) and the Hans M¨
uhlenhoff Foundation (especially
uhlenhoff himself) for enabling the intensive occupation with the presented
research field, close cooperation with our project partners and participation at na-
tional and international conferences for scientific exchange.
I cannot end without turning to the (more or less) non-scientific side of my thesis:
For sympathy, support, company (partly since early times at school), and many spe-
cial moments I would like to thank my friends: Jessica, Kristina, Johannes and Nina
(thanks also for providing helpful feedback on an early draft of my manuscript), Ka-
rina, Ole, Nele, Ivo, Tamara, Dominik, Anne, Sylvia, Christiane, Christoph, Tobias,
Jule, Nora, Judith and Larissa.
Finally, I wish to express my love and gratitude to all my family, especially to Andr´e,
Ann-Kathrin, Julia, Hendrik, Tobias, Fabian and Lucas, and, most importantly, to
my parents for loving and supporting me and for having an enormous amount of
faith in me.
Sulfadiazine (SDZ) belongs to one of the largest classes of antibiotics applied in
Europe in animal husbandry. Exposed to the environment by the release of manure
as fertilizer to agricultural soils or directly by the excrements of grazing animals
sulfonamides may affect soil microorganisms and soil functions, and may enhance
the spread of bacterial resistance. In order to asses the impact and the risk of SDZ
on soil organisms a fate model approach for prediction of temporally resolved pore
water concentrations has been developed. Pore water constitutes the easily available
fraction in soil which serves as interface variable between chemical fate and micro-
biological effect models.
Before combining all processes in one model it is necessary to develop mathemat-
ical descriptions for single processes and quantify the influence of substance and
environmental parameters. Thus, in a first step, relevant chemical processes of the
veterinary antibiotic sulfadiazine (SDZ) after medication to pigs were identified in
manure and manure-amended soil. Models to describe the chemical fate were devel-
oped, parameterized and integrated with growth inhibiting effects on soil bacteria.
Based on experimental data sensitive parameters and correlations between microbial
processes and substance fate were analyzed.
Sulfadiazine is a dissociating compound and its environmental fate as well as its
effect on microorganisms is strongly influenced by the pH value of the surrounding
solution. The dissociation equilibrium is determined by solution pH and the dissoci-
ation constant pKa of the substance from which the fraction of each species (anion,
neutral, cation) can be calculated. SDZ is partly metabolized to and excreted as
N4-acetyl-sulfadiazine (Ac-SDZ) which reacts back to SDZ during storage of the
manure prior to application and in the manure-amended soil. Substantial amounts
of the hydroxylated metabolite (OH-SDZ) were also identified in pig manure and
manure-amended soils but seem to remain unaffected by chemical transformation
processes on the investigated timescale.
Reversible equilibrium sorption in manure and soil depends on matrix properties
such as water content, organic carbon content, mineral surfaces, pH etc. Previously
measured equilibrium sorption coefficients are in the range of 1-10 L kg−1. In this
work a relatively small value of 0.56 L kg−1 has been estimated for two investi-
gated soils. However, estimations using this apparent partition coefficient K∗ show
that more than 80% of SDZ mass is sorbed to the solid matrix in investigated soils
Kaldenkirchen, a silty sand, and Merzenhausen, a sandy loam. Based on existing
literature data for different sulfonamides it was shown that an independent predic-
tion of sorption coefficients is still not possible as the widely used KOC concept does
not fully explain the observed variations in Kd values.
The chemical fate model was developed on the basis of available experimental data
in manure amended soil. It considers all dynamic fate and transformation processes
as first order kinetics. Model scenarios clearly show that dissipation of SDZ and
its metabolites in soil is dominated by a reversible translocation from the available
fraction into the residual fraction. This process may be based on a physical en-
trapment of the compounds with subsequent sorption. The irreversible formation of
bound residues (BR) proved to be of importance for sulfadiazine leading to a signi-
ficant reduction of the available antibiotic fraction. It is still not fully understood
which mechanistic processes are responsible for the observed BR formation. Latest
experimental results indicate a covalent binding between the amino group of the
sulfonamide and humic substances in soil (Bialk and Pedersen, 2008). This suggests
that formation of bound residues in soil can be restricted to SDZ and OH-SDZ which
is confirmed by the model.
Uptake and bioaccumulation of sulfonamides in bacterial cells is one link between
chemical fate and antibiotic effect of the compounds and is simulated by a dynamic
model describing the transport of neutral and ionic compound fractions through
the cell wall as a diffusion-like process. Comparison with effect data underlines that
dissociation in dependence of intra- and extracellular pH determines substance ac-
cumulation. The approach has been extended by including the enzymatic reaction
that is affected by sulfonamides, namely the production of DHP within the folic
acid cycle. Effects are modelled in terms of competitive inhibition of the enzyme
DHPS and are integrated with the chemical fate of SDZ on a molecular scale. In de-
pendence of enzymatic parameters (Vmax, Km) the applied substance concentration
which results in a 50% inhibition of the DHP production can be estimated.
Combining estimations on equilibrium sorption with the chemical fate model pore
water concentrations of SDZ can be simulated kinetically and compared to effective
concentrations which are exceeded for several days after manure application.
Mode of Action of Sulfonamides . . . . . . . . . . .
Environmental Relevance . . . . . . . . . . . . .
Summary of Experimental Investigations . . . . . . . .
Joint Research Project . . . . . . . . . . .
Feeding experiment . . . . . . . . . . . .
Investigated soils . . . . . . . . . . . . .
Central fate experiments . . . . . . . . . . .
2 Speciation of Sulfonamides
Investigated Substances
3 Sulfonamide Equilibrium Sorption
Sorption mechanisms and modelling approaches . . . . 18
Sorption behaviour of sulfonamides . . . . . . . . 21
Applied model approaches . . . . . . . . . . 24
Analysis of available literature data . . . . . . . . 25
Sensitivity analysis of the sorption behaviour of SDZ in the
Estimation of apparent sorption coefficients . . . . . 31
Effect of speciation and sorption on sulfonamide concentration
Dynamic distribution ratios . . . . . . . . . . 34
4 Transformation Reactions
Fit to manure extraction data . . . . . . . . . 44
Fit to extraction data of soils amended with fresh manure . 44
Simplification of the model . . . . . . . . . . 54
5 Uptake of Sulfonamides by Microorganisms
Transport into bacterial cells . . . . . . . . . . 60
Model parameterization and sensitivity analyses of the uptake
Development of the model for competitive enzymatic kinetics . 64
Parameterization of the enzymatic sub-model . . . . . 67
Accumulation of sulfonamides in bacterial cells . . . . . 68
Sensitivity of permeability ratio . . . . . . . . . 70
Influence of intracellular pH . . . . . . . . . . 71
Influence of extracellular pH . . . . . . . . . . 72
Comparison of model results with effect data . . . . . 73
pH dependent growth inhibition of soil bacterial isolates . . 75
Integration of enzymatic inhibition into the uptake model . . 79
6 Final Conclusions
Chemical structures of (a) sulfadiazine (SDZ) and its main metabo-
lites (b) N4-acetyl-sulfadiazine (Ac-SDZ) and (c) 4-hydroxy-sulfadiazine
Scheme of sulfadiazine ionisation in equilibrium . . . . . . 12
Fractions of ionic and uncharged forms of sulfadiazine in dependence
Fractions of neutral and anionic molecule of N4-acetyl-sulfadiazine in
Calculated speciation of five sulfonamides in the two soils of Kaldenkirchen
(a) Calculated fractions of the neutral and the anionic species of sul-
fachloropyridazine as a function of pH. (b) Measured and estimated
equilibrium sorption coefficients K∗ in a clay loam and a sandy loam,
respectively, as a function of pH . . . . . . . . . . . 26
Estimated versus measured (Ter Laak et al., 2006) apparent sorption
coefficients of SPZ calculated with species specific sorption coefficients
and with additional matrix properties in eleven different soils . . . 28
Effect of relative sorption strength of anionic (a) and cationic (c) sul-
fadiazine species on apparent K∗ in soils Kaldenkirchen and Merzen-
Model structure for transformations of SDZ and its metabolites Ac-
SDZ and OH-SDZ in manure and soil . . . . . . . . . 41
Measured data of sulfadiazine (SDZ), N4-acetyl-sulfadiazine (Ac-SDZ)
and 4-hydroxy-sulfadiazine (OH-SDZ) and simulated concentrations
Measured and simulated concentrations of sulfadiazine (SDZ), N4-
acetyl-sulfadiazine (Ac-SDZ) and 4-hydroxy-sulfadiazine (OH-SDZ)
in soil Kaldenkirchen amended with fresh manure in the (a) avail-
able and (b) residual as well as bound residue (BR) fraction . . . 47
Measured and simulated concentrations of sulfadiazine (SDZ), N4-
acetyl-sulfadiazine (Ac-SDZ) and 4-hydroxy-sulfadiazine (OH-SDZ)
in soil Merzenhausen amended with fresh manure in the (a) avail-
able and (b) residual as well as bound residue (BR) fraction . . . 48
Measured and simulated concentrations of sulfadiazine (SDZ) and
4-hydroxy-sulfadiazine (OH-SDZ) in soil Kaldenkirchen amended
with aged manure in the (a) available and (b) residual as well as
bound residue (BR) fraction . . . . . . . . . . . . 50
Measured data and simulated concentrations of sulfadiazine (SDZ)
and 4-hydroxy-sulfadiazine (OH-SDZ) in soil Kaldenkirchen amended
with aged manure in the (a) available and (b) residual as well as
bound residue (BR) fraction . . . . . . . . . . . . 51
Measured data and simulated concentrations of sulfadiazine (SDZ)
and 4-hydroxy-sulfadiazine (OH-SDZ) in soil Kaldenkirchen amended
with aged manure in the (a) available and (b) residual as well as
bound residue (BR) fraction . . . . . . . . . . . . 53
Simplified model structure for transformations of SDZ and its metabo-
lites Ac-SDZ and OH-SDZ in manure and soil . . . . . . . 54
Structure of the model integrating uptake of sulfonamides into the
bacterial cell and the subsequent enzymatic reaction in competition
Effect of pKa2 and Pd on potential sulfonamide (a) accumulation fac-tor (AF) and on (b) anion accumulation factor (AAF) in E. coli cells
Effect of pKa2 and intracellular pH on anionic accumulation factor inbacterial cells for pHenv = 7.0 . . . . . . . . . . . 71
Calculated anionic accumulation factor of nine sulfonamides with
different pKa2 in bacterial cells for an intracellular pH of 7.6 andthree different extracellular pH values . . . . . . . . . 73
Comparison of minimum inhibitory concentration (MIC) of 10 diffe-
rent sulfonamides (5.0 < pKa2 < 10.1) from Mengelers et al. (1997)measured at constant extracellular pH of 7.0 with the simulated anion
accumulation factor (AAF) . . . . . . . . . . . . 74
Simulated intracellular anion concentrations of sulfonamides normal-
ized to the maximum value for better comparison with effect data . 76
Growth inhibitory activities of sulfonamides (sorted with rising pKa2)as normalized reciprocal values at different pH on Pantoea agglomer-
Simulated intracellular anion concentrations of sulfonamides for diffe-
rent extracellular pH values and variable intracellular pH values (pHi) 78
Anion accumulation factor at pHenv = 7.0 of different sulfonamides
at two concentrations without (AAF) and with (AAFe) consideration
of the subsequent enzymatic reaction in dependence of the respective
5.10 Correlation between the pKa2 value and the simulated sulfonamide
concentration (DHPinh(50)) at which the inhibition results in a 50%
reduction of the DHP-formation in the cell . . . . . . . . 81
Soil parameters of the Kaldenkirchen and Merzenhausen sampling site
Important substance parameters for 13 sulfonamides . . . . . 10
Measured apparent sorption coefficients (K∗) and estimated ranges of
neutral sorption coefficient Kn . . . . . . . . . . . 30
K∗ (L kg−1) (mean ± standard deviation) calculated from subsequent
extraction experiments . . . . . . . . . . . . . 32
Kinetic rate constants resulting from a least-squares fit of the model
to extraction data of soils Kaldenkirchen and Merzenhausen after ap-
plication of fresh (3 weeks) manure . . . . . . . . . . 46
Kinetic rate constants resulting from a least-squares fit of the model
to original and adapted extraction data of soil Kaldenkirchen after
application of fresh (3 weeks) and aged manure (6 month) . . . 52
Kinetic rate constants resulting from a least-squares fit of the sim-
plified model to adapted extraction data of soils Kaldenkirchen and
Merzenhausen after application of fresh (3 weeks) and aged manure
Sulfadiazine (SDZ) is one of the oldest and still widely used sulfonamides, a group of
synthetically produced antibiotics, which was introduced in 1939 (Vree and Hekster,
1987). Gerhard Domagk, who was the director of Bayer's Institute of Pathology and
Bacteriology, studied the antibiotic effect of prontosil, a dye containing sulfamyl,
which protected mice from streptococcus. However, this product only showed its an-
tibiotic action in the living animal. In 1935, a French research team at the Institute
Pasteur in Paris discovered that prontosil is just the prodrug, i.e. the substance itself
is in an inactive form and is metabolized in the body into an active compound, in
this case into sulfanilamide. Since then, several molecules based on the effective sul-
fonamide group R-SO2NH2 have been developed to improve antibiotic activity andtolerance, i.e. lower dissociation constant to improve solubility and, consequently, to
avoid crystallisation in the kidneys (Vree and Hekster, 1987).
Since 2006 the use of antibiotics as food additives and growth promotors is for-
bidden in European animal husbandry1. Nevertheless, in several European countries
sulfonamides still constitute one of the largest groups of antibiotic compound classes
(Thiele-Bruhn and Aust, 2004). In Germany, for example, they come in third with
still increasing use volume after tetracycline and β-lactam antibiotics applied in
veterinary medicine (Schneidereit, 2006).
1 Regulation (EC) No 1831/2003 of the European Parliament and of the Council of 22 September
2003 on additives for use in animal nutrition. Official Journal of the European Union L 268/29-43.
CHAPTER 1. AIM AND SCOPE
Mode of Action of Sulfonamides
Sulfonamides act very effectively against bacterial or protozoal infections often in
combination with trimethoprim. They are bacteriostatic compounds meaning that
they inhibit bacterial cell growth reversibly. Based on their structural analogy to
para-aminobenzoic acid (pABA) which is necessary for the synthesis of folic acid
sulfonamides are competitive antagonists in the enzymatic reaction of pABA with
dihydropteroate synthase (DHPS) and inhibit the production of dihydropteroate
(DHP). If this key step is missing the lack of folic acid inhibits the bacterial cell to
generate nucleic acids (DNA) and, thus, to divide in the presence of sulfonamide.
Therefore, sulfonamides can only act against microorganisms which cannot take
up folic acid but have to synthesize it on their own using endogenous compounds.
Gram positive and negative coccoi, gram negative rods like E. coli, but also chlamy-
diaceae and protozoans belong to this group of microorganisms. This also explains
why mammalian cells which depend on dietary supply of folate are not affected by
sulfonamides (Vree and Hekster, 1987; Alexander et al., 1990). The bacteriostatic
effect of sulfonamides becomes visible after a few hours of latency, i.e. when the pool
of folic acid is depleted.
The combination with trimethoprim potentiates the antibiotic effect since trimetho-
prim inhibits the second step of the folic acid synthesis by acting against the dihy-
drofolate reductase. In addition, fewer resistances are generated if the combination
of the two antibiotics is applied.
Environmental Relevance
Antibiotics are defined as "low molecular weight microbial metabolites" that at low
concentrations inhibit the capability of microorganisms to reproduce as well as in-
hibit the growth of an individual cell (Lancini and Parenti, 1982; Thiele-Bruhn,
2003). Their properties (high stability and water solubility as well as high "toxicity"
against certain organisms) make them feasible to act very effectively against bacte-
rial infections or infections by protozoans even at low doses. Excess molecules of the
parent compound and the metabolites are completely excreted from the body after a
short time of residence. Therefore, residual concentrations of sulfonamides and their
metabolites can be found in the environment which may be very mobile, persistent
and effective/toxic. Consequently, urine of the treated animal contains a mixture
of parent compound and transformation products (Thiele-Bruhn and Aust, 2004;
1.2. ENVIRONMENTAL RELEVANCE
Boxall et al., 2002). Therefore, the most important emission pathway of sulfadiazine
and its main metabolite N4-acetyl-sulfadiazine (Kreuzig and H¨oltge, 2005) into the
environment is the excretion of urine of livestock animals including both, grazing
livestock and the use of liquid manure as fertilizer to agricultural land (Thiele-Bruhn,
2003; Boxall et al., 2003; Kay et al., 2005a). Emissions mainly occur to surface waters
and soil via aquaculture, intensive livestock treatments, and runoff from agricultural
soils, whereas releases of veterinary medicines into the atmosphere and impacts of
emissions from treating pets as well as direct disposal of unused or expired products
and waste containers are considered less relevant (Boxall et al., 2002).
Hardly any information is available about the ecological toxicity of antibiotics (Thiele-
Bruhn, 2003) which is necessary to address possible adverse effects of antibiotics in
the environment. Antimicrobial substances are of particular interest due to the pos-
sible dispersion of bacterial resistance (Sithole and Guy, 1987; Kay et al., 2005a;
G¨obel et al., 2005) in the environment triggered by emission of the compounds.
Resistant microbial populations are already wide-spread in the environment and
also present in soils since soil organisms produce several antibiotics and appropriate
avoidance strategies. The ongoing use of antibiotics in livestock and the application
of manure to agricultural soils, however, may further contribute to this problem
(Pils and Laird, 2007; Heuer and Smalla, 2007). Both, the antibiotics and resistant
organisms may be transferred to and accumulate in the food chain and thus reduce
the success of pharmacotherapy of animals and humans (Rhodes et al., 2000; Kumar
et al., 2005).
The application of contaminated manure enhances the distribution of bacteria which
obtain an evolutionary advantage by resistance genes. There are at least two diffe-
rent ways of "developing" resistances: Some microbes have its own genes that impart
resistance by mutation; other antibiotic-susceptible bacteria receive resistance genes
via plasmids (packets of genetic material) from already resistant bacteria and in-
corporate them into their own chromosomes (Josephson, 2006). For sulfonamides,
different resistant genes could be isolated from Escherichia coli found in pigs and
even in humans also indicating a possible transfer via the food chain (Infante et al.,
2005; Hammerum et al., 2006). Especially in environments influenced by agriculture
an increased occurrence of sul-genes was detected (Pruden et al., 2006). In addition,
the appearance of the sulfonamide in the environment may affect the composition
of microbial communities and, accordingly, ecological functions.
Burkhardt and Stamm (2007) investigated the fate and depth distribution of three
CHAPTER 1. AIM AND SCOPE
sulfonamides in a loamy grassland soil and detected sulfonamide concentrations in
pore water close to the soil surface of 20 to 50 µg L−1 which are in the same range as
effect concentrations (B¨ohm, 1996). Accordingly, Christian et al. (2003) determined
sulfonamide concentrations in soils of 15 µg kg−1 (d.w.) seven month after manure
application. From soil, sulfonamides may leach into groundwater (Hamscher et al.,
2005) or may be transported into surface water via runoff (Kay et al., 2005a,b).
Nevertheless, observed concentrations in groundwater and runoff are low (Blackwell
et al., 2007). This is confirmed by investigations of Kreuzig et al. (2005) who point
out that runoff of sulfonamides can be neglected after tillage of the agricultural soil.
In order to evaluate influences of soil properties and environmental conditions on
the chemical fate of sulfonamides, however, a deeper knowledge of underlying mech-
anisms is necessary. Finally, risk assessment has to include both parent compound
and its metabolites. Otherwise, the environmental effects of sulfadiazine and of vet-
erinary medicines in general, might be underestimated (Boxall et al., 2003; G¨obel
et al., 2005).
Summary of Experimental Investigations
Joint Research Project
This thesis was developed within a research project funded by the German Research
Foundation (DFG) called "Veterinary Medicines in Soils: Basic Research for Risk
Analysis". The aim of the investigations was to elucidate the fate and effects of sul-
fadiazine and its metabolites in homogenized soil systems. This thesis is based on
the experimental results obtained by project partners within the research project.
A brief summary of the experiments is given in this chapter.
During a feeding experiment sulfadiazine was applied to pigs and manure was col-
lected daily. Incubation experiments with fresh (3 weeks after production) and aged
manure (after 6 month storage) were carried out in two different soils in the absence
of plants and macrofauna under controlled laboratory conditions, i.e. constant tem-
perature and moisture conditions. The applied manure was completely incorporated
into the investigated soils and the chemical fate of SDZ and its two main metabolites
was analytically followed for 218 days. Simultaneously, biological effects of sulfadi-
azine on soil microorganisms and soil functions were determined in both test soils.
This included the investigation of influences of the antibiotic itself and of manure
application on the microbial community structure by analyzing DNA sequences. In
1.3. SUMMARY OF EXPERIMENTAL INVESTIGATIONS
addition, the antibiotic effect on functional diversity of soil microbes was elucidated
by measuring soil respiration and impacts on nitrogen turnover (N-mineralization,
nitrification, denitrification etc.). Further studies differentiated whether the appli-
cation of the sulfonamide or the input of nutrient and resistant bacteria via ma-
nure affect abundance, diversity and transfer of antibiotic resistances in soil bacteria
(Kotzerke et al., 2008; Heuer et al., 2008; Schauss et al., 2008).
Feeding experiment
14C-labelled as well as non-labelled SDZ was administered to four growing pigs
and manure was collected daily. SDZ and its metabolites were determined and
quantified in homogenized manure samples. Besides the two main metabolites N4-
acetyl-sulfadiazine (Ac-SDZ) and 4-hydroxy-sulfadiazine (OH-SDZ) (Fig. 1.1) two
minor metabolites were recovered, i.e. N-acetyl-4-hydroxy-sulfadiazine and N-formyl-
sulfadiazine which were neglected in further investigations since they accounted for
less than 2% of the total radioactivity in manure. During manure storage under aero-
bic and anaerobic conditions at 20◦C up to 165 days, concentrations of SDZ, Ac-SDZ
and OH-SDZ were analyzed to follow dissipation and transformation processes of the
antibiotics in manure (Lamsh¨oft et al., 2007; Spiteller, 2007).
Figure 1.1: Chemical structures of (a) sulfadiazine (SDZ) and its main metabolites (b) N4-acetyl-
sulfadiazine (Ac-SDZ) and (c) 4-hydroxy-sulfadiazine (OH-SDZ).
Investigated soils
Within the central experiment of the research project two typical German soils,
namely silty sand from Kaldenkirchen (pH 5.7) and sandy loam from Merzenhausen
(pH 6.8) were used for application of manure resulting from the feeding experiment.
CHAPTER 1. AIM AND SCOPE
Table 1.1: Soil parameters of the Kaldenkirchen and Merzenhausen sampling site.
soil density (kg L−1)
water content (kg kg−1)
All subsequent investigations on the chemical fate and biological effects of sulfadi-
azine were conducted in these homogenized soil samples. Important properties of
the two soils are summarized in Table 1.1.
Central fate experiments
Homogenized soil samples were amended with fresh (3 weeks after production) and
aged (6 month storage) manure from pigs treated with 14C-SDZ. Soil moisture was
adjusted to 30% of the maximum water holding capacity (WHCmax) resulting in
a water content of 7.2% and 11.5%, respectively. Transformation and sequestration
processes were observed in the two different soils. A sequential extraction method was
developed by Amelung and Kaupenjohann (2007) and applied to the homogenized
soil samples resulting in three different sulfonamide fractions that were extractable
with (i) CaCl2, (ii) methanol (MeOH) and (iii) by applying high temperatures (mi-crowave extraction for 15 minutes with acetonitrile/water at 150◦C) only (residual
fraction). Each fraction was investigated by LC/MS to quantify the amounts of SDZ,
Ac-SDZ and OH-SDZ. Finally, bound residues defined as the fraction not extractable
by any of the methods applied are identified by measuring the radioactivity in the
extracted soil sample (Amelung and Kaupenjohann, 2007). These time-dependent
extraction data served as the basis for the estimation of apparent sorption coeffi-
cients K∗ (Chapter 3) and for the process model developed in this thesis (Chapter
The overall objective of this thesis is to develop a mechanistic simulation model pre-
dicting temporally resolved concentrations of sulfadiazine and its metabolites in soil
pore water and the subsequent uptake of the antibiotically active compounds by mi-
croorganisms. Before combining all processes in one model, however, it is necessary
to understand the mechanisms of relevant processes and to quantify the influence of
substance and environmental parameters. Therefore, important processes governing
the chemical fate of sulfadiazine and its main metabolites in manure and manure-
amended soil shall be identified and described mathematically. Partition coefficients,
transformation rates and other process variables shall be estimated from experimen-
tal results of project partners. Influences of environmental conditions and substance
properties shall be figured out and the chemical fate shall be linked to biological
effects. The pore water fraction determines bioaccumulation, toxicity and leaching
potential and thus, represents the interface between chemical fate and biological ef-
fect of sulfadiazine. For prolonged contact times in soil, available SDZ in pore water
and consequently efficiency of the veterinary medicine is reduced, e.g. by sorption,
diffusion into micropores and/or transformation processes.
This thesis is subdivided into specific chapters which are structured according to
following processes. Since sulfonamides belong to the group of dissociating com-
pounds, the pH value is expected to play an important role for their environmental
fate (Chapter 2). The sorption behaviour of SDZ and its metabolites will be reviewed
and investigated based on available data. Model approaches for sorption of organic
substances in soil shall be evaluated on their appropriateness to describe sulfadi-
azine sorption in manure and soil (Chapter 3). A system and data analysis helps to
understand observed transformation and sequestration kinetics of SDZ in manure
and manure-amended soils under different environmental conditions (Chapter 4).
Finally, a link between the chemical fate and the antibiotic effect on soil microbes
shall be realized by combining the fate model with a mathematical description of
the uptake of the antibiotic compound into the bacterial cell and the subsequent en-
zymatic inhibition of the folic acid cycle (Chapter 5). This is the first approach that
successfully integrates both, fate and effect, into a consistent model describing the
whole process chain from manure application to soil until the uptake of the relevant
sulfonamide fraction into the bacterial cell and the subsequent enzymatic inhibition
of bacterial growth. Such a model would constitute a valuable tool in the process of
risk analysis and risk assessment of such compounds.
Speciation of Sulfonamides
Investigated Substances
Within the research project the focus was on the environmental fate and effect of
sulfadiazine, a bacteriostatic substance which belongs to the group of sulfonamides.
Since only few data on the environmental fate and effects of sulfadiazine (equilib-
rium sorption, transformation, sequestration, enzymatic inhibition) are available and
chemical structure of substances within the sulfonamide group is similar, experimen-
tal results on sorption, transformation and sequestration behaviour and on effective
concentrations of other sulfonamides are included in the theoretical discussion of
this thesis in order to allow for deeper insight into general mechanisms. Table 2.1
summarizes some basic properties of relevant sulfonamides.
Many antibiotic compounds such as sulfonamides dissociate or protonate in depen-
dence of the pH value of the surrounding medium and their specific dissociation
constants (pKa). The ionized species may show a different distribution behaviour
and reactivity in comparison to the uncharged neutral form (Thiele-Bruhn, 2003;
utzhoft et al., 2000). This already points out the necessity to include sulfon-
amide speciation in environmental fate modelling (Gao and Pedersen, 2005). Thiele-
Bruhn (2005) showed that effective doses on the microbial iron(III)-reduction in soils
vary depending on the speciation of sulfonamides. Therefore, with regard to fate and
effect it is important to know, to what extent anions and/or cations are formed in
a given environmental surrounding.
CHAPTER 2. SPECIATION OF SULFONAMIDES
Table 2.1: Important substance parameters for 13 sulfonamides.
n. a. - not available; a pKa and Kt data from Sakurai and Ishimitsu (1980) except otherwise noted;
bHolm et al. (1995); cLin and Lu (1997); dMengelers et al. (1997); eVree and Hekster (1987);
f Thiele-Bruhn and Aust (2004), Sarmah et al. (2006); gGarten Jr. and Trabalka (1983); hHansch
Sulfonamides are characterized by two pKa values: The lower one indicates protona-
tion of the amino group whereas the other one signifies deprotonation of the SO2NHmoiety (Ingerslev and Halling-Sørensen, 2000). The fractions of the three possible
species (acidic, neutral, basic) can be estimated as follows:
Given are the proton transfer reactions of the acidic species SH+
species SH (i.e. the sum of the uncharged and possibly zwitterionic form) and of the
neutral species to the anion S− with the dissociation constants Ka =[SH][H+] and
Ka =[S−][H+]. The fraction α
of the cationic species is calculated according to
Schwarzenbach et al. (2003) describing the equilibrium in acid-base reactions:
2 ] + [S−]/[SH +
1 + Ka /[H+] + K · K /[H+]2
1 + 10pH−pKa1 + 102·pH−pKa1−pKa2
The fractions of the other two species are derived accordingly resulting in:
1 + 10pKa1−pH + 10pH−pKa2
a2 −2·pH + 10pKa2−pH
To be more precise we also have to investigate whether the molecule of sulfadiazine
may exist as a zwitterionic species, i.e. as a molecule which is both negatively and
positively charged at the same time. If the pKa-values act in the order of classic
ampholytes which means if the acidic pKa-value is smaller than the basic pKa-value,
a zwitterion can exist. First, with increasing pH the acidic pKa-value affects the
deprotonatable group of the molecule in a way that the cation which predominates
at lower pH-values is additionally charged negatively. This mechanism results in a
zwitterion. If the pH value further increases the basic pKa-value causes the proto-
natable group to release its hydrogen molecule and it becomes negatively charged.
As far as ampholytes are concerned there exist detailed illustrating publications (e.g.
Pagliara et al. (1997)).
However, if the pKa-values are located close to one another and an intramolecu-
lar charge exchange may occur, zwitterions can even exist in the opposite case, i.e.
the acidic pKa-value is larger than the basic one. This situation applies to sulfadi-
azine and to sulfonamides in general and was investigated by Sakurai and Ishimitsu
(1980). These authors have calculated tautomeric constants of selected sulfonamides
describing the concentration ratio of zwitterionic and uncharged species. As the neu-
tral fraction represents the sum of uncharged and zwitterionic species, which show
deviating environmental behaviour because of their different charge distribution, the
equations above have to be supplemented for the distinction of the neutral fraction
αSH using the tautomeric constant Kt:
CHAPTER 2. SPECIATION OF SULFONAMIDES
Figure 2.1: Scheme of sulfadiazine ionisation in equilibrium.
Figure 2.2: Fractions of ionic and uncharged forms of sulfadiazine in dependence of pH (pKa1 =
2.49, pKa2 = 6.48, Kt = 147.9, Sakurai and Ishimitsu (1980)).
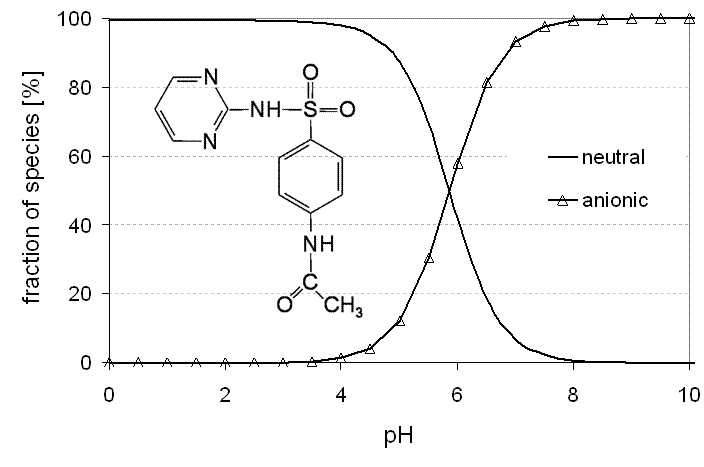
αS−H+ = αSH − αSH0
αSH0 and αS−H+ are the fractions of the uncharged and the zwitterionic form, re-spectively.
The complete dissociation scheme of sulfadiazine is shown in Figure 2.1 which il-
lustrates the transitions from one ionisation state to another in equilibrium. In Fig-
ure 2.2 the speciation of sulfadiazine in dependence of the pH value is given over the
full range of possible values.
The fraction of the zwitterionic species of sulfadiazine is negligible (< 0.7%) com-
pared to the neutral form due to its large tautomeric constant. Thus, this species is
considered to be of minor importance and is not explicitly distinguished from the
neutral form in the model. However, small fractions of a species can affect the over-
all sorption if strong sorption to specific binding sites occurs. This was pointed out
by Gao and Pedersen (2005) for the adsorption of sulfamethazine to clay minerals
Figure 2.3: Fractions of neutral and anionic molecule of N4-acetyl-sulfadiazine in dependence of
pH (pKa = 5.86, Vree and Hekster (1987)).
CHAPTER 2. SPECIATION OF SULFONAMIDES
at low pH values. The cationic form dominates the adsorption up to a pH of 4.6,
although this species constitutes only 0.45% of total sulfamethazine at this pH. A
similar effect of the zwitterionic form, however, was not observed by the authors.
In order to completely assess the environmental risk of sulfadiazine metabolites of
the parent compound have also to be considered. The N4-acetyl-metabolite is char-
acterized by a single dissociation constant of pKa=5.86 (Vree and Hekster, 1987)
which indicates deprotonation of the SO2-NH-moiety. The protonation of the aminogroup as observed for the parent compound sulfadiazine is not possible because the
amino group is blocked by acetylation. Thus, in dependence of the surrounding pH,
N4-acetyl-sulfadiazine may exist as neutral and anionic species (Figure 2.3). The
second major metabolite is the 4-OH-metabolite that is formed by hydroxylation
at the sulfonamide's pyrimidine ring. The hydroxy-sulfadiazine may be additionally
deprotonated at the OH-group. However, since the respective dissociation constant
is far below the environmental relevant range (pKa <2, Mason (1958)) this can be
neglected. Unfortunately, the dissociation constants specifying protonation of the
amino group and deprotonation of the SO2NH moiety are not known and it is thusassumed that they are close to the respective pKa-values of the parent compound.
Sulfonamide Equilibrium Sorption
Sorption is one of the most important processes hampering transport of chemicals to
groundwater or surface water and also affecting transformation reactions and effects
on soil microbes (Schwarzenbach et al., 2003). Therefore, an extensive knowledge on
parameters affecting the sorption of antibiotics to soil is a necessary prerequisite for
risk and exposure assessment. For sulfonamides, which partly dissociate in depen-
dence of the actual pH, the different species-specific sorption mechanisms have to
be distinguished. These mechanisms depend on soil composition and chemical prop-
erties resulting in different apparent sorption coefficients for each investigated soil
and sulfonamide. Besides the pH ionic strength, clay minerals and organic matter
content and composition may play a critical role for the sorption of sulfonamides to
the soil matrix. Several investigations on sulfonamide sorption to soil (Thiele-Bruhn
and Aust, 2004; Ter Laak et al., 2006; Langhammer, 1989; Drillia et al., 2005), soil
fractions (Thiele-Bruhn et al., 2004) and clay minerals (Gao and Pedersen, 2005)
have yet been conducted. Reported sorption coefficients of sulfonamides are in the
range of 0.6 to 7.4 L kg−1 (Sarmah et al., 2006) which translates into a mass fraction
of 50% to 95% of the compound that is sorbed under average conditions in natural
soils. Therefore, knowledge of the apparent Kd, i.e. the "visible" sorption coefficient,
is not sufficient, because it cannot be transferred to other soils. Gao and Pedersen
(2005) investigated the sorption of three sulfonamides to three clay minerals over a
large pH range from 3.5 to 9.3 and estimated species-specific sorption coefficients by
CHAPTER 3. SULFONAMIDE EQUILIBRIUM SORPTION
fitting the results to a model explicitly considering the contributions of the cationic,
zwitterionic, uncharged and anionic species to the apparent sorption coefficient. Al-
though the results varied across the different clay minerals and can thus not be used
to describe soil sorption of sulfonamides in general, the investigation clearly shows
the necessity to include speciation in environmental fate modelling. In this work,
a mechanistic model simultaneously considering speciation and sorption of sulfon-
amides to available sorption data of sulfonamides in soil matrices is applied. The
model is used to identify sensitive parameters under average conditions in natural
In contrast to air and water, soil does not constitute a homogeneous compartment
but is of heterogeneous composition combining the lithosphere, the hydrosphere, the
atmosphere, and the biosphere by formation of the pedosphere. Thus, it is the upper
animated layer of the earth´s surface that is not saturated by water (Trapp and
Matthies, 1998). The German Federal Soil Protection Act (Bundesbodenschutzgesetz
§ 2)1 defines the soil by means of its functions, i.e. the soil is the upper layer of the
earth´s crust including the soil solution and the soil air (but not the groundwater)
which fulfils the following functions:
• natural functions as basis of life and habitat for soil organisms, plants, animals
and humans, as part of the ecosystem including water and nutrient cycles,
and as a medium for degradation, balancing and development resulting from
filtering, buffering and transformation properties particularly with regard to
the protection of groundwater
• functions as archive for natural and cultural history
• functions as raw material deposit, area of settlement and recreation, location
of agriculture and forestry, of commercial and public use, traffic, provision and
1 Bundesministerium f¨ur Umwelt, Naturschutz und Reaktorsicherheit, BBodSchG 1998,
After all, soil is characterized by interactions between solid minerals, water, gases
and dissolved inorganic and organic substances (Korte, 1992). Therefore, according
to Trapp and Matthies (1998) the fate and transport of a contaminant in soil is
influenced by several processes:
• diffusion and dispersion in gas and water filled pores
• advection with soil water and leaching to groundwater
• bioturbation by earthworms, mice and soil organisms
• sorption to soil solids (minerals and organic matter)
• sorption to dissolved organic matter (DOM)
• volatilization into the atmosphere
• transformation and degradation
• uptake by plant roots
Soil composition with respect to organic matter content and composition of the
mineral fraction (sand, silt, clay) has an influence on the actual sorption capacity
of a given soil. Other important soil properties are porosity, water content, specific
surface area (of minerals mainly) and cation exchange capacity.
The specific surface area is the sum of all boundary layers between solid and liquid
or solid and gaseous phases and is measured in m2 g−1. It ranges from a few m2 g−1
up to 500 m2 g−1 and increases with rising content of clay, expandable minerals and
organic substances. The surface charge, a parameter which plays an important role
when investigating polar or ionizable compounds, depends on the pH value: Sorption
of cations increases with increasing pH, whereas sorption of anions increases with
decreasing pH. Consequently, the proportion of the soil´s charge mainly results from
the type and amount of its sorbents. In temperate climate, soils are characterized
by clay minerals and a negative net charge over the whole range of pH from 3 to
8 as the negative charge always exceeds the positive. If the number of positively
charged groups equals the number of negatively charged groups there is only mini-
mal interchange activity. This point is called charge zero point. The cation exchange
mechanism describes sorption via electrostatic bindings. The sum of all exchangeable
cations (in cmolc kg−1) is called cation exchange capacity (CEC) and increases with
increasing pH. The potential CEC (CECpot) is measured at pH-values of 7 to 7.5,
CHAPTER 3. SULFONAMIDE EQUILIBRIUM SORPTION
the highest possible value in soils of humid climates, i.e. the effective CEC (CECeff )
is lower than CECpot when the pH value falls below 7. For a single cation its contri-
bution to the cation film increases with increasing concentration in the equilibrium
solution. The exchange approximates asymptotically a threshold which is the CEC.
However, in soils different cations compete for exchange sites. Therefore, the contri-
bution of a cation in the film under ecological conditions depends on its contribution
in the equilibrium solution and its ability to compete for sorption sites compared to
other cations.
Finally, as far as chemicals are concerned that dissociate to anions or cations in a
pH range typical of central European soils, i.e. 3 to 8, the pH value is an important
influencing factor for sorption processes. As pollutants are not ecologically effective
until desorption and transfer to the soil solution occured, reversibility of sorption
processes is also of great importance (Schachtschabel et al., 1998).
Sorption mechanisms and modelling approaches
Environmental modelling is a common approach for risk assessment in science and
policy, i.e. it supports the evaluation and prediction of a substance's behaviour
in the environment under the assumption of certain environmental conditions and
substance properties. Such a model is not only restricted to prediction, but also
allows for deducing mechanisms and structures of the represented system. In this
context, the equilibrium partitioning of organic substances is of specific interest for
analyzing the fate and transport of sulfadiazine in soil (Schwarzenbach et al., 2003),
as the mechanistic background of the involved sorption processes is not yet fully
The relationship between the equilibrium concentrations of the substance sorbed to
the soil matrix (Cs, g kg−1 soil) and the concentration dissolved in solution (Cw,
g cm−3 solution) can be expressed by the Langmuir model that assumes a limited
sorption capacity Q due to a limited surface:
with factor b representing the affinity of the surface for the solute. Under these re-
strictive assumptions the Langmuir isotherm is only observed in a few cases (Delle Site,
2001), e. g. for the adsorption of polycyclic aromatic hydrocarbons from water onto
activated carbon (Walters and Luthy, 1984).
Experimental data, however, is often best fitted by the Freundlich model (Weber Jr.
et al., 1992) which assumes neither homogeneous energies nor restricted sorption.
Kf is the Freundlich coefficient and the exponent n is a measure of nonlinearity.
A special case of this model occurs for n = 1 which leads to a linear relationship
between Cs and Cw. In the low concentration region, the Freundlich isotherm also
obeys to a quasi-linear relationship. Thus, for environmental conditions this linear
approach is often used to describe sorption of a solute to sediment or soil by the
partition coefficient Kd between soil matrix and water as follows:
The Kd value can be determined by measuring the linear part of the adsorption
isotherm or, as nonpolar substances are mainly sorbed to the organic matter of the
soil matrix, it can be estimated from the octanol-water partition coefficient KOW
and the organic carbon content (Trapp and Matthies, 1998). However, this estima-
tion does not consider polar interactions that may be important for antibiotics and
neglects the complexity and diversity in soil components and their interactions with
organic solutes. Nor, does it provide a deeper insight into sorption mechanisms and
influencing factors. Hence, Weber Jr. et al. (1992) proposed the Distributed Reac-
tivity Model (DRM) which contributes to the significance of "different distributions
of sorption reactions and mechanisms for different solute-solid combinations" com-
bining components of different linear (l) and nonlinear (nl) sorption:
Cs is the total sorbed substance equilibrium concentration, x
l is the summed mass
fraction of solid phase showing linear sorption, KD is the mass-averaged partition
coefficient for the summed linear components, Cw is the equilibrium concentration
in solution, and (xnl)i is the mass fraction of the ith nonlinearly sorption process
each described by the Freundlich model. For practical purposes, the number of dis-
tinguishable nonlinear components will not exceed 1 or 2 (Weber Jr. et al., 1992).
An even more mechanistically based model called polyparameter linear free energy
relationships (PP-LFERs) or cavity model was introduced by Goss and Schwarzen-
bach (2001). The single parameter relationships like the often applied KOC approach
are only valuable for one single compound class and do not allow any inference on
CHAPTER 3. SULFONAMIDE EQUILIBRIUM SORPTION
the involved mechanisms in order to understand the variability of sorption behaviour
between substance classes or between different natural organic phases. Based on this
situation Goss and Schwarzenbach (2001) developed a concept that takes all parti-
tioning interactions of organic compounds into account by specific parameters. In
this way, mechanistic insight and understanding of the various interactions that in-
fluence the environmental distribution of organic chemicals is provided. Thus, the
partitioning Ki12 of a substance i between two bulk phases 1 and 2 which accountsfor van der Waals forces and H-bonding take the following form:
ln (Ki12) = a12 · CAi · vdWi + b12 · Vi + d12 · HAi + e12 · HDi + c12
CAi is a measure for the contact area between i and the phases, Vi is the mo-
lar volume of i, HAi and HDi are quantitative descriptors of the H-accepting and
H-donating properties of the interacting partners of i, and a12 to e12 are regressionparameters dependent on the properties of the phases. Once determined for a certain
surface (which in fact is a quite laborious effort), such a PP-LFER allows to describe
sorption of an organic chemical without any additional chemical input parameter.
However, this model has not been tested and adapted for use with ionic compounds
or at fairly high concentrations (Goss and Schwarzenbach, 2001) and is, thus, not
yet applicable for substances like sulfonamides dissociating in an environmentally
relevant pH range.
Besides others, Breivik and Wania (2003) successfully applied the concept of PP-
LFERs to multimedia fate models, as many chemicals of environmental concern
like modern pesticides and pharmaceuticals show a partitioning behaviour different
from those of the pollutants for which single parameter approaches work quite well.
Nguyen et al. (2005) also showed that the PP-LFER approach surpasses other cur-
rent methods which aim at estimating the equilibrium partition coefficient KOC of
organic compounds between water and the natural organic matter in soils. Only very
little effort has yet been put on modelling the sorption of antibiotics to soils, nei-
ther using the classical approach nor by applying the PP-LFER concept. Figueroa
et al. (2004) fitted sorption isotherms for the zwitterion of tetracycline antibiotics to
clay to the Langmuir model. The results are restricted to the indication that phar-
maceutical sorption interactions with clay are controlled "by the ionic functional
groups of the base compound structure within a pharmaceutical class". Another ap-
proach was pursued by Wehrhan et al. (2007) investigating the transport behaviour
of sulfadiazine in soil. The "concentration dependent, rate-limited and possibly ir-
reversible sorption processes" were fitted by a kinetic model with two reversible at-
tachment/detachment sites and one irreversible sorption site. The soil concentration
profile, though, could not be described by this approach. This may be due to the
experimental setup, because the authors did not analytically distinguish between
the parent compound and possible transformation products so that the observed
radio-label signal includes sulfadiazine and its metabolites. However, the fate of the
individual compounds in the soil column may be quite different and data do not
describe the transport of sulfadiazine alone. Thus, the model does not provide any
mechanistic insight as underlined by Goss and Schwarzenbach (2001) and Breivik
and Wania (2003).
Usually, modelling approaches describing the fate of organic chemicals in soil com-
bine sorption and degradation behaviour. Liu et al. (2007) developed a mass balance
model including equilibrium sorption to the solid matrix, an irreversible kinetic se-
questration process and biodegradation by soil microorganisms following Monod
kinetics. Simulation results were in good agreement with data from biodegradation
batch tests with benzene. Saffih-Hdadi et al. (2003) simultaneously modelled the
fate of the pesticide parathion and its highly toxic metabolite paraoxon underlining
that both, the parent compound and the metabolite, have to be considered for risk
assessment especially if the transformation product is active or even more toxic than
the pesticide itself. Both substances were assumed to sorb into a weak sorption phase
followed by a strong sorption phase which might be irreversible. These approaches
indicate that a distinction between sulfadiazine and its metabolites is required in
order to analyse and model the effect of its exposure to soil. Furthermore, in a de-
tailed investigation dissociation of single species which underlie different sorption
processes in dependence of their charge should be explicitly considered. In this way,
deeper mechanistic insight and an improved risk assessment is possible even with
a small data set (as compared to the PP-LFER approach) combining matrix and
Sorption behaviour of sulfonamides
Sorption of sulfonamides is mainly driven by the aromatic amino group which also
forms covalent bonds (bound residues) with phenolic humic substances (Bialk et al.,
2005; Bialk and Pedersen, 2008). Since for the metabolite N4-acetyl-sulfadiazine
(Ac-SDZ) this functional group is replaced, weaker sorption and bound residue for-
mation is expected as compared to its parent compound. The chemical moiety of the
amino group also determines the antibiotic effect of the sulfonamides which means
CHAPTER 3. SULFONAMIDE EQUILIBRIUM SORPTION
that adsorption might inhibit the antibiotic effect (Thiele, 2000). Sorption equilib-
rium of sulfonamides in soil is reached very fast after several hours (Thiele-Bruhn,
2003; Langhammer, 1989). In equilibrium, the distribution between solid and liquid
phase can be described by the partition coefficient Kd representing the ratio between
equilibrium concentrations in solids and aqueous solution. Batch experiments indi-
cate sorption nonlinearity for sulfonamides (Thiele-Bruhn and Aust, 2004; Wehrhan
et al., 2007) best fitted by Freundlich isotherms. However, in a concentration range
of 350 to 1500 µg kg−1 linear sorption isotherms have been reported (Zimmermann,
Sorption to soil. Tolls (2001) summarized Kd values of sulfonamides in different
soils varying from 0.6 to 4.9 L kg−1. Langhammer (1989) observed that Kd values
of a given compound differ considerably in diverse soils and that these variations
cannot be solely explained by differences in organic carbon content. Boxall et al.
(2002) investigated the effect of pH on sorption of sulfachloropyridazine in the pH
range from 4.6 to 7.8. Measured sorption coefficients increased from less than 1 L
kg−1 to 12 L kg−1 with decreasing soil pH. Recalling that the fraction of the anion
increases with increasing pH sorption of the anionic sulfachloropyridazine appears
to be significantly weaker than that of the neutral form. The cationic species can
be neglected within the investigated pH range. Also, Kahle and Stamm (2007) re-
ported that sorption of sulfathiazole to organic matter and minerals decreases with
inreasing pH. In analogy, Kurwadkar et al. (2007) showed that apparent sorption
coefficients increased significantly (about one order of magnitude) with increasing
pH for sulfamethazine and sulfathiazole in three different soils and pH variations
between 2.3 and 8.0. The resulting sorption coefficients for each of the sulfonamide
species (cationic, neutral, anionic) were consistent in all three soils and were de-
termined by an empirical model. They indicated strongest sorption for the cationic
form followed by the sorption strength of the neutral and the anionic sulfonamide
molecule. Accordingly, Gao and Pedersen (2005) point out that anionic species of
sulfonamides do not participate in sorption interactions, whereas the cationic form
dominates adsorption to clay minerals even at pH values at which this form covers
only 0.45% of the investigated compound. The zwitterionic form, however, does not
seem to influence sorption behaviour of sulfonamides.
Sorption of sulfonamides to soil organic matter (SOM) is generally stronger than
to soil minerals (Thiele-Bruhn, 2003), and depends on composition of SOM. Previ-
ous studies indicate (Thiele-Bruhn and Aust, 2004; Thiele-Bruhn et al., 2004) that
sorption of sulfonamides is also influenced by the molecular structure and by physico-
chemical properties of the substance in dependence of pH, by functional groups at
organic surfaces, and by cavities in the structure of soil organic matter. Several in-
teractions between substance and soil can be involved in the sorption process of the
ionic and neutral species (Liu and Yu, 2005). Sorption can be attributed to van-
der-Waals forces and hydrogen bonding forming complexes between the sulfonamide
and soil organic matter (Thiele-Bruhn et al., 2004) as well as covalent binding, sur-
face complexation in case of mineral adsorbents (Schwarzenbach et al., 2003) and
electrostatic interactions in case of ionic and polar species.
Sorption to manure. In general, the adsorption of antibiotics to manure that is
rich in organic matter is strong (Thiele-Bruhn, 2003; Delle Site, 2001) and attributed
to ionic interactions and hydrogen bonds (Tolls, 2001). Numerous carboxylic and
phenolic components which may act as exchange sites for sulfonamides result in a
significantly stronger sorption to manure than to soil (Thiele-Bruhn and Aust, 2004).
Burkhardt and Stamm (2007) determined sorption coefficients in manure (pH 8.1)
ranging from 30 to 40 L kg−1 for sulfathiazole and sulfadiazine which is about one
order of magnitude above reported Kd values in soil. But what happens when manure
is amended to soil? Manure contains high levels of ammonia increasing the pH value
of the soil solution after addition. Besides an increase of the cation exchange capac-
ity (CEC) (Schachtschabel et al., 1998) the species distribution of the compound is
altered. Boxall et al. (2002) related the mobilizing effect of manure on sulfonamides
in soil to the pH effect of the alkaline manure favouring the anionic species.
Increasing mobility of sulfonamides has also been observed in the presence of dis-
solved organic matter (DOM). DOM is suspected to act as a co-solvent that enhances
concentrations in the dissolved phase by sorption of the substrate (in this case sulfon-
amides) to DOM. Manure contains N-heterocyclic hydrocarbons which can compete
for adsorption sites at soil particles with N-heterocyclic sulfonamides but a competi-
tive effect would only be visible if available adsorption sites were almost saturated
with the competitors from manure. Thus, for typical manure application rates Kd
values for sulfachloropyridazine were almost constant for different DOM concentra-
tions (Boxall et al., 2002). In addition, despite the rather fast initial sorption of
sulfonamides to soil after manuring Stoob et al. (2007) found that sulfonamides are
still present in the soil after a long time period.
CHAPTER 3. SULFONAMIDE EQUILIBRIUM SORPTION
Materials and Methods
Applied model approaches
Equilibrium sorption. Equilibrium sorption was estimated based on fundamentals
of sorption theory (Schwarzenbach et al., 2003) considering characteristic physico-
chemical parameters of sulfonamides and relevant soil properties. The apparent sorp-
tion coefficient K∗ is given by the total equilibrium concentrations in the sorbed
phase (Ctot,sorb) and the dissolved phase (Ctot,diss) and is expressed as the mass
fraction weighted average of the contributions of the cationic, neutral and anionic
species (eq. 3.6). As the zwitterionic fraction of sulfonamides is known to be small
under environmental conditions (Sakurai and Ishimitsu, 1980), it is not explicitly
αi is the fraction of the cationic (c), neutral (n) and anionic (a) species, and Ki the
respective species-specific sorption coefficients. These sorption coefficients represent-
ing the interactions between a single species and the solid matrix can be assumed to
be nearly constant within each soil even for different pH values. Thus, for a specific
soil or manure sample the apparent Kd depends on the species distribution as a
function of pH and pKa and the ratio of the species-specific distribution coefficients.
However, depending on the matrix properties the latter are not constant for different
soils. To allow for transferring from one soil to another the species-specific interac-
tions with the solid matrix have to be identified and quantitatively estimated.
The cationic species undergoes electrostatic interactions with negatively charged
mineral surfaces which could be related to the cation exchange capacity (CEC) of
the soil. The anionic species can be attracted by the soil matrix via surface-bridging
mechanisms. Sorption of the neutral molecule is most probably due to unspecific
van-der-Waals interactions or hydrogen bonding. The former depends on the total
fraction of soil organic matter, whereas the latter also accounts for H-donor and
H-acceptor properties of the soil matrix and the compound. It is desirable to in-
dependently estimate the species-specific sorption coefficients of sulfonamides for
a given soil. However, information that allows for establishing quantitative rela-
tionships using soil and substance properties is not yet available. For this reason,
equation 3.6 was used to conduct a sensitivity analysis for the contributions of the
different species to the apparent K∗. A number of scenarios were simulated for soils
Kaldenkirchen (pH 5.7) and Merzenhausen (pH 6.8) which are described in Chapter
3.4. RESULTS AND DISCUSSION
1.3. As the pKa2 of SDZ is between the average pH values of the two soils, theyreflect conditions that result in a significantly different speciation of SDZ, i.e. the
neutral species dominates in soil Kaldenkirchen (86%) whereas in soil Merzenhausen
SDZ mainly exists as anion (68%). The estimated cationic fraction is very small in
both soils (< 0.1%). Consequently, if neutral and anionic species of sulfadiazine ex-
hibit significantly different sorption strength this might be reflected by diverging
equilibrium sorption observations for the two soils.
Results and Discussion
Analysis of available literature data
Equation 3.6 describes the contribution of the cationic, neutral and anionic species
to the apparent sorption coefficient K∗. Most of the sulfonamides are dominated ei-
ther by the neutral or by the anionic fraction under natural pH conditions as shown
in Chapter 2. The estimated species distribution for five sulfonamides in the two
different soils Kaldenkirchen (K) and Merzenhausen (M) is shown in Figure 3.1. It
can be seen that the neutral fraction is higher in soil K except for SPY which does
not show significant dissociation in both soils.
Estimation of species-specific sorption coefficients for SPZ. For sorption of
sulfachloropyridazine (SPZ) to soils two independent data sets are available (Boxall
et al., 2002; Ter Laak et al., 2006). Boxall et al. (2002) investigated the effect of pH
on sorption of sulfachloropyridazine (SPZ) onto the solid matrix of a clay loam and
a sandy loam in a pH range of 4.6 to 7.8. Measured sorption coefficients were in the
range of 12 L kg−1 to less than 1 L kg−1 and showed a clear tendency of decreasing
with increasing soil pH. As the neutral fraction of sulfachloropyridazine also de-
creases with increasing soil pH in the two soils (Fig. 3.2a), this indicates that overall
sorption is dominated by interactions of the neutral molecule with the soil matrix. If
the contributions of the cationic fraction are considered to be negligible, a bivariate
linear regression of K∗ versus α
n and αa delivers estimates for the species-specific
sorption coefficients Kn and Ka. At high pH values where the anionic species consti-
tutes to almost 100% of total SPZ the respective K∗ should approximately equal K
Regression analysis for the two data sets resulted in significantly different values for
Kn (7.2 L kg−1 for sandy loam and 14.0 L kg−1 for clay loam) and Ka (0.6 L kg−1
sandy loam, 1.2 L kg−1 clay loam). Kn is approximately one order of magnitude
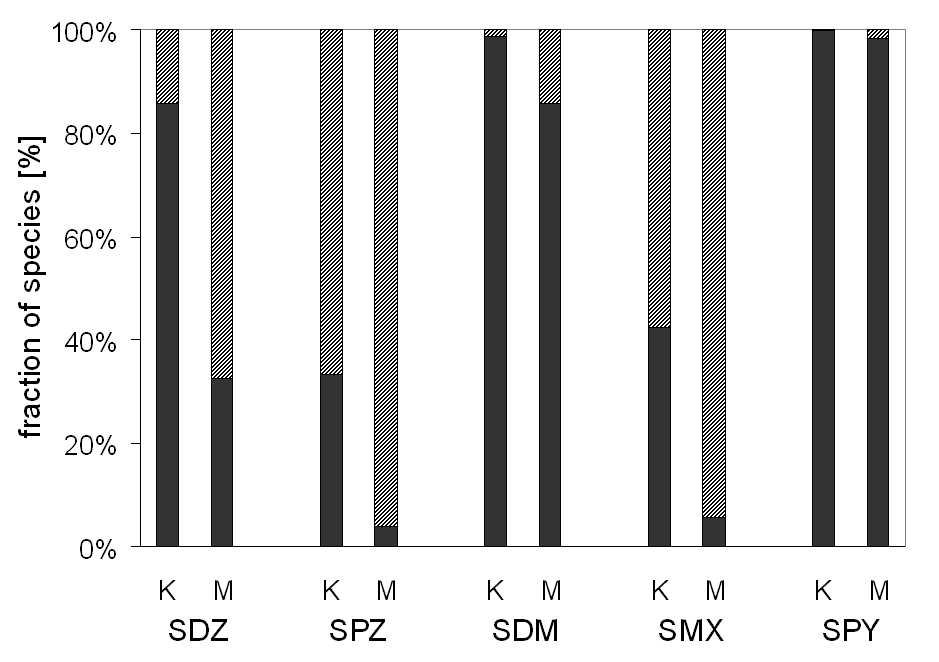
CHAPTER 3. SULFONAMIDE EQUILIBRIUM SORPTION
Figure 3.1: Calculated speciation of five sulfonamides (SDZ: sulfadiazine, SPZ: sulfachloropyri-
dazine, SDM: sulfamethazine (=sulfadimidine), SMX: sulfamethoxazole, SPY: sulfapyridine) in the
two soils of Kaldenkirchen K (pH 5.7) and Merzenhausen M (pH 6.8). The neutral fraction (%)
is given in black, the anionic fraction is given in diagonally stripes. Relevant substance properties
are presented in Table 2.1.
Figure 3.2: (a) Calculated fractions of the neutral and the anionic species [%] of sulfachloropy-
ridazine (pKa1=1.88, pKa2=5.4, Lin and Lu (1997)) as a function of pH. (b) Measured (symbols)
and estimated (lines) equilibrium sorption coefficients K∗ (L kg−1) in a clay loam and a sandy
loam, respectively, as a function of pH (data from Boxall et al. (2002)).
3.4. RESULTS AND DISCUSSION
(factor 10) larger than Ka. Additionally, both partition coefficients are larger for the
clay loam as compared to the sandy loam supporting that species-specific sorption
coefficients vary between different soils and matrices. Figure 3.2b shows that this
simple estimation is able to describe the pH-dependency of the observed partition
Ter Laak et al. (2006) published sorption coefficients of sulfachloropyridazine (SPZ)
in 11 different soils accomplished by important soil parameters (pH, OC, CEC)
which permits to investigate possible correlations of sulfonamide sorption behaviour
to matrix properties. Fitting equation 3.6 to these sorption data by a least-square-
method (LSM) results in distribution coefficients of Kn = 15.3 L kg−1 ± 97.4% and
Ka = 1.6 L kg−1 ± 231% with a small r2 of 0.32. This indicates that sorption of
sulfonamides to soil cannot be explained by overall species-specific sorption coeffi-
cients. Cationic sorption is negligible (Kc = 0 L kg−1). However, since the cationic
fraction is small (less than 3%) in all investigated soils this term is very insensitive
to the optimization routine.
Kn and Ka derived from Boxall et al. (2002) are both significantly different within
the two investigated soils indicating that specific soil properties play an important
role for sorption of SPZ. This is corroborated by the large standard deviations ob-
tained above for the data set of Ter Laak et al. (2006). Thus, species-specific sorption
coefficients cannot be transferred between different soils without taking the variabil-
ity of matrix properties into account.
According to Tolls (2001) several mechanisms are involved in sorption of veterinary
pharmaceuticals to soils. He proposes to differentiate between sorption to organic
matter and mineral constituents, ion exchange and reactions like H-bonding and
complexation. Therefore, it is of particular interest to gain additional information
on the involved sorption mechanisms and determining parameters of the sulfadiazine
species in order to transfer sorption data from one soil to another.
The cationic contribution to overall sorption is assumed to be related to the cation
exchange capacity (CEC) of the soil matrix. A common approach to estimate sorp-
tion of organic molecules to solid material is the KOC-concept. Here, it is assumed
that sorption is proportional to the organic carbon content (OC) and therefore the
sorption coefficient K∗ can be estimated by a normalized partition coefficient be-
tween organic carbon and water (KOC) (Trapp and Matthies, 1998). However, the
validity of this concept for veterinary pharmaceuticals in particular and for many
polar organic compounds in general is doubtful (Thiele-Bruhn and Aust, 2004; Tolls,
CHAPTER 3. SULFONAMIDE EQUILIBRIUM SORPTION
2001). The concept assumes sorption to be dominated by van der Waals interactions
with soil organic carbon neglecting hydrogen bonding forces.
Nevertheless, this is tested and it is assumed that Kn and Ka are attributed to
van-der-Waals interactions proportional to the organic carbon content, (KOC,n and
KOC,a, respectively) and other interactions, e.g. H-donor-acceptor forces and/or ion
exchange (K′ and K′ ).
Fitting equation 3.7 to the sorption data of Ter Laak et al. (2006) results in distri-
bution coefficients of KOC,n = 283.7 L kg−1 ± 12.4% and KOC,a = 61.0 L kg−1 ±
77.5% with r2 = 0.86. Cationic sorption proved negligible again. The contribution
of K′ and K′ also turns out to be negligible according to the model fit. In Figure
3.3 measured and estimated sorption coefficients calculated with the two equations
(eq. 3.6 vs. eq. 3.7) in the eleven different soils are compared. It can be seen that the
introduction of the additional parameter OC (eq. 3.7) improves the agreement of the
estimated values with data from experiments for most of the data points. However,
Figure 3.3: Estimated versus measured (Ter Laak et al., 2006) apparent sorption coefficients
of SPZ calculated with species specific sorption coefficients (eq. 3.6, grey diamonds) and with
additional matrix properties (eq. 3.7, black triangles) in eleven different soils.
3.4. RESULTS AND DISCUSSION
overall sorption can equivalently be described with K′ alone leaving out the addi-
tional term (OC · KOC,a). This results in an only slightly worse fit (r2 = 0.83) with
almost the same estimated K∗ values (data not shown in Fig. 3.3). Nevertheless,
there are still obvious deviations that clearly show the need for more experimental
data to enable deeper mechanistic insight.
After all, the analysis of SPZ sorption data corroborates the assumption that cationic
sorption does not contribute significantly to overall sorption of sulfonamides under
environmental pH conditions, since the cationic fraction is negligible. It can also be
concluded that anionic sorption of SPZ - and probably other sulfonamides as well - is
significantly weaker than neutral sorption (one order of magnitude). An investigation
by Gao and Pedersen (2005) of the sorption of three sulfonamides (sulfamethazine,
sulfamethoxazole, sulfapyridine) to clay minerals corroborates the small contribution
of the anionic species to overall sorption. They concluded that the anionic species
does not participate in sorption interactions with clay minerals which is justified
by electrostatic repulsion of the anion by the negatively charged surfaces. Approxi-
mation of SPZ sorption to different soils by OC-normalized distribution coefficients
KOC,i does not fully explain the observed variation in partitioning of SPZ and is
thus only a first step towards a generally applicable equation for the independent
estimation of sulfonamide sorption in soils from substance and matrix properties.
SDZ sorption data. Literature data for K∗ of SDZ are scarce and are restricted
to a narrow pH range (6.9 - 7.5). Thus, it is not surprising that variation of the
observed sorption coefficients is also small (1.4 to 2.8 L kg−1) (Thiele-Bruhn and
Aust, 2004; Thiele-Bruhn et al., 2004; Sarmah et al., 2006). With the assumption
that the cationic species does not contribute significantly to the overall sorption,
upper and lower boundaries for Kn can be estimated. The upper limit is given by
the assumption that sorption of the anion is also negligible and sorption is solely
explained by the neutral fraction (K∗ ≥ α
n · Kn). The lower limit makes use of the
estimated ratio of 0.1 for Ka/Kn derived above from SPZ literature data. Inserting
this ratio into equation 3.6 results in K∗ ≤ K
n · (αn + 0.1 · αa). Applying these
estimations to available literature data, results in a range of 6.4 L kg−1 to 22.9 L
kg−1 for the Kn of SDZ (Table 3.1).
CHAPTER 3. SULFONAMIDE EQUILIBRIUM SORPTION
Table 3.1: Measured apparent sorption coefficients (K∗) and estimated ranges of neutral sorption
coefficient Kn assuming that the cationic fraction is negligible and, for minimum estimation,
sorption of the anion is smaller than of the neutral species (Ka = 0.09 · Kn) and, for maximum
estimation, that anionic sorption strength is negligible (αn·Kn ≤ K∗). Data from 1Thiele-Bruhn
et al. (2004) and 2Thiele-Bruhn and Aust (2004).
1clay fraction of silt loam 1
1sand fraction of silt loam 1
Sensitivity analysis of the sorption behaviour of SDZ
in the investigated soils
The pKa2 value of SDZ is close to neutral and thus, fractions of neutral and anionicmolecules are very sensitive to pH changes in the environmentally relevant range.
Sulfadiazine (pKa2 6.48) is mostly neutral (86%) in soil Kaldenkirchen (pH 5.7),whereas in soil Merzenhausen (pH 6.8) the anionic fraction prevails (68%). The minor
role of the anionic fraction to sulfadiazine sorption in these two soils can be confirmed
by a sensitivity analysis. Figure 3.4 displays the apparent sorption coefficient K∗ in
dependence of the sorption strength of the anionic and cationic species relative to
the neutral species expressed as the ratio of the species-specific sorption coefficients.
It can be seen that under these circumstances a significant effect of anion sorption on
K∗ requires higher sorption strength of the anion compared to the neutral molecule
(Ka > Kn) which is in contrast to the findings derived from literature data above. The
cationic species in the two soils is rather small (< 0.1%) due to the low dissociation
constant pKa1 which is responsible for protonation of the amino group. Thus, it canonly be of importance for the overall sorption, if the cationic sorption coefficient (Kc)
exceeds the neutral one (Kn) by a factor of at least 100. Figure 3.4 shows that K∗dvalues increase for Kc/Kn > 100 in Kaldenkirchen soil, whereas in Merzenhausen soil
even a ratio of 1000 does not significantly increase overall sorption. This sensitivity
analysis corroborates the minor role of the cationic species for the overall sorption of
sulfonamides to soils under natural conditions. However, this also implies that the
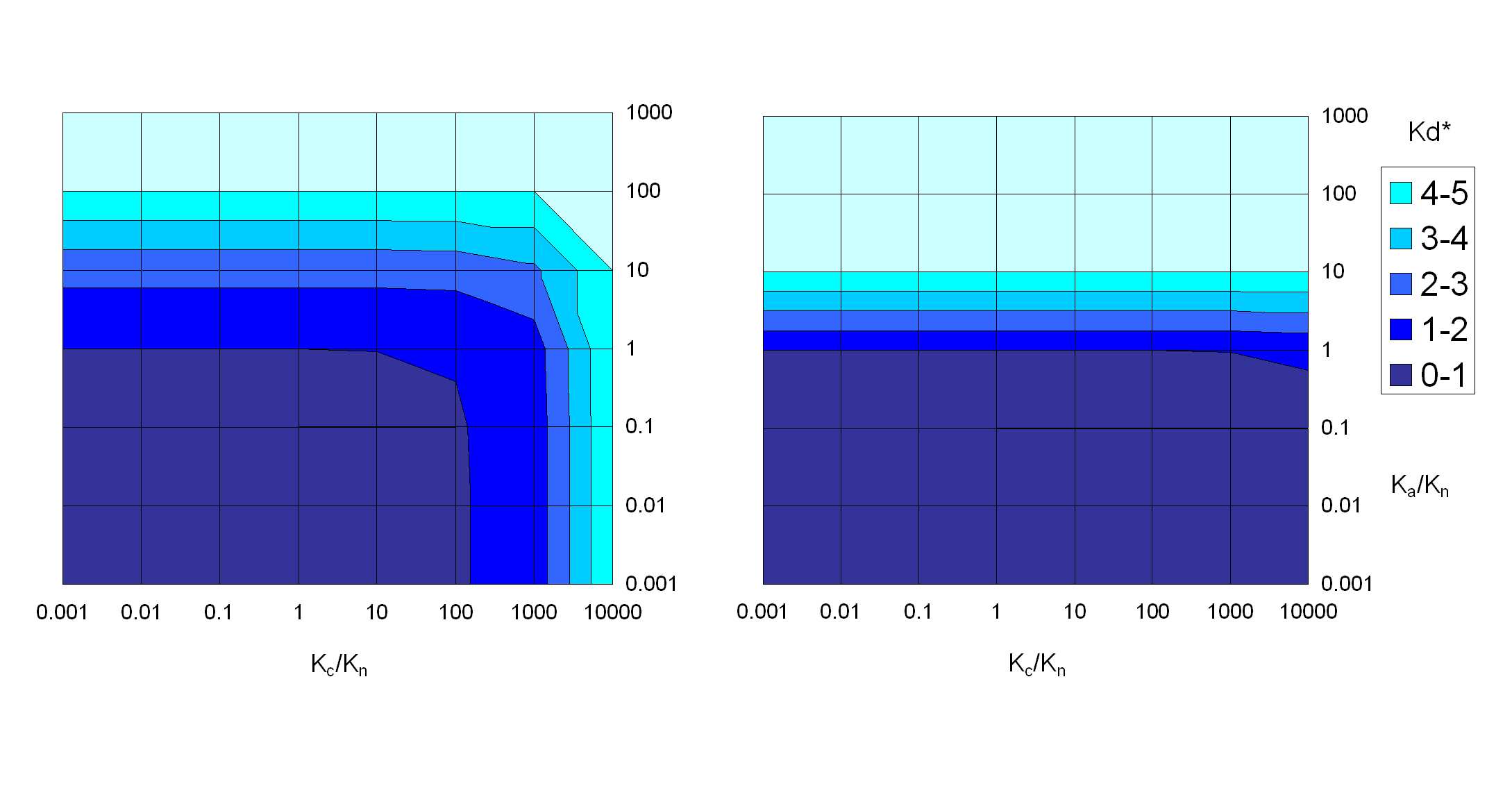
3.4. RESULTS AND DISCUSSION
Figure 3.4: Effect of relative sorption strength of anionic (a) and cationic (c) sulfadiazine species
on apparent K∗ in soils Kaldenkirchen (left) and Merzenhausen (right). K
n was arbitrarily set to
sorption coefficient Kc cannot be reliably estimated from existing sorption data due
to its insensitivity.
Estimation of apparent sorption coefficients
The experimental determination of sorption coefficients is usually performed with
soil samples that have been spiked with the substance of interest. Aliquots of the
spiked sample are then equilibrated with deionized water or CaCl2 solution. Afteran appropriate equilibration time the aqueous solution is separated from the solid
matrix (often by centrifugation) and analysed. This fraction is assumed to contain
the dissolved equilibrium concentration. Correctly, the equilibrium concentration on
the adsorbent had to be determined independently after extraction. However, this is
often omitted and a simple mass balance calculation is used to estimate the amount
of substance sorbed.
Within the research, the two manure-amended soils were sequentially extracted at
specific dates with CaCl2 solution followed by methanol (Amelung and Kaupen-johann, 2007). The results can be used to get a first estimation of the sorption
behaviour of SDZ and its metabolites in the two soils. It is assumed that the sub-
stance mass in the CaCl2 extract represents the dissolved fraction in equilibrium
CHAPTER 3. SULFONAMIDE EQUILIBRIUM SORPTION
with the fraction reversibly sorbed to the soil matrix during extraction. The dis-
tribution coefficient K∗ (L kg−1) of this equilibrium process is given by the ratio
between the concentration on the solid matrix (µg kg−1 dry soil matter) and the
concentration in the CaCl2 extract. Assuming further that methanol exhaustivelyextracts the remaining reversibly sorbed substance fraction from the solid matrix
after CaCl2 extraction the apparent sorption coefficients of SDZ, Ac-SDZ and OH-SDZ can be estimated from available extraction data (Amelung and Kaupenjohann,
2007). The concentration on the solid matrix is then calculated by the substance
mass in the methanol extract divided by the dry weight of the extracted soil sample.
The concentration of the dissolved fraction is given by the substance mass in the
CaCl2 extract divided by the total volume of the solution. The latter is composedof the volume of the CaCl2 solution added to the soil sample and, if fresh and notdried soil samples are investigated, of the soil water of the sample.
Experimental data indicate that the total mass in the extracts decreased with time.
Nevertheless, the calculation described above can be performed for all soil samples
since in each extraction step the actual equilibrium concentration of the CaCl2 so-lution and the solid matrix were determined by independent analytical procedures.
Table 3.2 shows the resulting K∗ values calculated from the extraction data. Only
data points where both concentrations were above the quantification limit have been
used. For each soil and substance the variation between the single data points is
equally small with a coefficient of variation between 16% and 35%. Large variation
is only observed for the two smallest data sets (n=3) with individual data points
close to the quantification limit. Mean K∗ values are at the low end of literature
values for SDZ in different matrices, e.g. K∗ of SDZ in soil-slurry mixture 1.18 L
kg−1 (Thiele-Bruhn and Aust, 2004), K∗ of SDZ in soil 2.0 L kg−1 (Thiele-Bruhn
et al., 2004). This indicates that the above assumptions are valid and the apparent
distribution coefficients can be reliably estimated with the approach. Calculated K∗dvalues for SDZ and OH-SDZ are very similar in the two soils indicating that the
Table 3.2: K∗ (L kg−1) (mean ± standard deviation) calculated from subsequent extraction
experiments (Amelung and Kaupenjohann, 2007).
Kaldenkirchen 0.56 ± 0.09 (n=7) 0.16 ± 0.04 (n=5) 0.40 ± 0.09 (n=4)
0.57 ± 0.12 (n=6) 0.15 ± 0.05 (n=3) 0.26 ± 0.07 (n=3)
3.4. RESULTS AND DISCUSSION
effect of the different speciation (neutral fractions are 86% and 32%, respectively)
on sorption is balanced by the difference in the soil matrices. If we recall that for
sulfonamides Ka is approximately ten times smaller than Kn, the Kn value in soil
M is estimated to be a factor of three larger than in soil K. This is in line with the
larger fraction of organic carbon (0.52% vs 0.39%) and the significantly higher clay
contents (23.1% vs 3.0%) of soil M resulting in a larger surface area available for
binding processes.
Effect of speciation and sorption on sulfonamide con-
centration in soil solution
An important point is the availability of a compound which is a prerequisite for
uptake by organisms and thus, in case of sulfonamides also for their inhibitory effect
(Zarfl et al., 2008b). Generally, the available fraction of a compound in soil is the
dissolved fraction in the soil solution. If it is assumed that environmental conditions
(e.g. pH, temperature, water content) are more or less constant over time the relative
distribution between the cationic, neutral and anionic species is constant in a given
system. In the two investigated soils, concentrations in soil solution (Cw) only depend
on sorption of the neutral molecule (Kn) and the anion (Ka) since the cation is not
able to persist under the respective pH conditions. In order to elucidate the effect
of speciation and sorption on the actual concentration in soil solution, the dissolved
sulfadiazine concentration Cw (in mol · L−1) can be predicted by using the following
wcont + K∗ · (1 − wcont)
Ctot is the total sulfonamide concentration in soil in mg · kg−1, wcont is the water
content (w/w), and ρW is the density of water (kg · L−1). This equation is valid if
substance distribution into soil air can be neglected and the assumption of instan-
taneous equilibrium holds, i.e. additional kinetic losses can be excluded. Equation
3.8 is used to estimate SDZ concentrations in soil solution of Kaldenkirchen and
Merzenhausen (properties see Table 1.1) using estimated K∗ values (Table 3.2). Af-
ter application of 2.8 mg kg−1 SDZ (44% of the total radioactivity (Lamsh¨oft et al.,
2007; Schmidt et al., 2008)) to investigated soils the pore water concentrations of
SDZ are calculated to be 4.7 mg L−1 in soil Kaldenkirchen and 4.5 mg L−1 in soil
Merzenhausen. Although estimated K∗ values are small and pore water concentra-
tions are high the fraction of the sorbed sulfadiazine in relation to the total SDZ
CHAPTER 3. SULFONAMIDE EQUILIBRIUM SORPTION
amount applied to soil accounts for more than 80% due to a low water content. The
concentration of dissolved sulfadiazine is essential for the antibiotic effect on soil
microorganisms. Tappe et al. (2008) determined pH dependent growth inhibitory
concentrations (EC50) of SDZ to range from 0.5 mg L−1 (pH 7) to 2.57 mg L−1 (pH5) for Pantoea agglomerans, a very sensitive soil bacterium, and from 2.85 mg L−1
(pH 5) to 15.4 mg L−1 (pH 7) for Pseudomonas aeruginosa. Thus, under the ap-
plied test conditions concentrations of SDZ are predicted to be in the effective range
for sensitive soil bacteria like P. agglomerans whereas the dissolved SDZ fraction
is probably too small to affect bacteria like P. aeruginosa. Consequently, antibiotic
effects were observed to a smaller extent in experiments with SDZ applied soil sam-
ples than with in-vitro laboratory experiments.
Applied K∗ values are at the low end of literature data which explains the relatively
high pore water concentrations. However, even with these low K∗ values the fraction
of sorbed sulfadiazine accounts for more than 80% of total SDZ in soil due to the
low water content during the experiments (7.2%, w/w and 11.5%, w/w). This means
that on the one hand larger partitioning coefficients would decrease actual pore wa-
ter concentrations possibly leading to a reduction of effectiveness. On the other hand
the sorbed fraction constitutes an easily available reservoir that can maintain the
pore water concentration level for longer periods of time.
Dynamic distribution ratios
Some investigations indicate that equilibrium sorption of sulfonamides includes an
additional kinetically limited sorption process which follows the fast initial phase
(Kreuzig and H¨oltge, 2005; Langhammer, 1989). In newer literature, the term "dy-
namic Kd values" has been used to describe sulfonamide sorption to manure, organic
matter or soil (Kahle and Stamm, 2007; Amelung and Kaupenjohann, 2007). In fact,
the observed increase in distribution ratios between solution and solid matrix cannot
be equalled with the equilibrium distribution coefficient K∗ which describes the con-
centration ratio of reversibly sorbed substance concentration and solved concentra-
tion in equilibrium. In contrast, this parameter includes the kinetic sequestration
process which is usually assigned as formation of "non-extractable" residues. In soil
science, the complexity of the compartment soil (Chapter 3.2.1) poses a challenge
to determine single processes and its parameters. Soil solution, air and solid matrix
(consisting of varying fractions of sand, silt, clay and organic carbon) represent an in-
tricate structure in which the compound underlies several processes simultaneously.
3.4. RESULTS AND DISCUSSION
As far as sorption of sulfonamides is concerned this means that a fast equilibrium
process and different binding processes following slower or irreversible kinetics can-
not be distinguished with one single measurement in time. After application of the
antibiotic compound to the soil sample and with increasing time the extractable
fraction may be additionally reduced by kinetically controlled translocation and/or
binding processes that can be either reversible or irreversible (bound residues). In
order to distinguish between the different fractions, Amelung and Kaupenjohann
(2007) developed a sequential extraction procedure which allows for a deeper insight
into underlying mechanisms. This is discussed in detail in the following Chapter 4.
Transformation Reactions and
Formation of Bound Residues1
Transformation reactions in manure. Veterinary antibiotics are prone to diffe-
rent metabolism reactions in the treated animal after administration. These pro-
cesses mainly lead to more polar structures that exhibit enhanced water solubility
favouring excretion via urine. Main metabolites of sulfadiazine (SDZ) metabolism in
pigs are long known to be N4-acetyl-sulfadiazine (Ac-SDZ) and 4-hydroxy-sufadiazine
(OH-SDZ) (Vree and Hekster, 1987). These two main metabolites were also identi-
fied in manure collected from pigs fed with 14C-SDZ (Lamsh¨oft et al., 2007).
During manure storage the parent compound and its excreted metabolites may be
further transformed or completely mineralized. Degradation of sulfonamides during
manure storage has been observed to proceed very slowly (Langhammer, 1989; Box-
all et al., 2003). For example, 40% of sulfadimidine and 60% of sulfathiazole were
unchanged after 5 weeks of manure storage under aerobic conditions (Langhammer,
1989). The most important transformation in manure is de-acetylation of Ac-SDZ
back to SDZ (Grote et al., 2004). This transformation was also observed for several
acetylated sulfonamide metabolites in wastewater treatment plants (G¨obel et al.,
2007). The OH-SDZ metabolite has been shown to be stable in manure under aer-
obic as well as anaerobic storage conditions (Heuer et al., 2008). Other metabolites
of minor importance have also been identified in manure, e.g. OH-N4-acetyl-SDZ
(Lamsh¨oft et al., 2007).
1 This chapter is in preparation for publication in Zarfl et al. (2008a).
CHAPTER 4. TRANSFORMATION REACTIONS
Transformation reactions in soil. Experimental investigations on formation of
CO2 from radio-labelled SDZ and its metabolites in manure-amended soils revealedthat less than 2% of the applied substance is mineralized (Kreuzig and H¨oltge, 2005;
Schmidt et al., 2008) even after 218 days. In analogy, biodegradation of sulfamethox-
azole in test schemes according to ISO standards was observed to be negligible
(Gartiser et al., 2007a,b). Thus, mineralization of sulfonamides in soil is of minor
importance. Though the FOCUS working group on degradation kinetics 20062 in-
cludes "products that are in bound residues" into one degradation sink, the evolution
of CO2 is the only appropriate endpoint for ultimate biodegradation (D¨orfler et al.,1995; Matthies et al., 2008). Whereas no hints for acetylation of sulfonamides in
the environment exist, rapid de-acetylation has been observed for sulfamethoxazole
(H¨oltge and Kreuzig, 2007). Ac-SDZ seems to be a metabolite which is only pro-
duced in the SDZ-treated animal and not in the environment whereas hydroxylation
of SDZ to OH-SDZ in soil has not yet been explicitly described.
Non-extractable residues and formation of bound residues. The issue of
non-extractable and bound residues first appeared in the context of risk assessment
of pesticides (Roberts et al., 1984). Here, both termini are equally applied assuming
that the non-extractable fraction completely belongs to the fraction of bound pesti-
cide residues. According to the European directive 91/414/EEC3 "non-extractable
residues present in the soil [. .] are chemical species (parent compound and metabo-
lites, or fragments) [. .], that cannot be extracted by methods which do not signif-
icantly change the chemical nature of these residues". This definition particularly
aims at the covalently bound fraction. Decreasing extractability is often described
as "aging" or sequestration (Amelung and Kaupenjohann, 2007). This can be at-
tributed to the formation of bound residues (BR) in which the compound is cova-
lently bound to the soil matrix (e.g. to humic materials (Bialk and Pedersen, 2008)),
but also to storage in cavities not accessible for extraction solvents (non-extractable
residues, NER) or to incorporation into microbial biomass (Charnay et al., 2004). In
2 FOCUS, 2006. Guidance Document on Estimating Persistence and Degradation Kinetics from
Environmental Fate Studies on Pesticides in EU Registration. Report of the FOCUS Work Group
on Degradation Kinetics, EC Document Reference Sanco/10058/2005 version 2.0.
3 European Economic Community, Council Directive 91/414/EEC of 15 July 1991 concerning the
placing of plant protection products on the market (Official Journal of the European Communities
L230, 19.08.91. p. 1).
4.1. INTRODUCTION
the following it will be clearly distinguished between non-extractable residues (NER)
as a "technical" term based on analytical methods and bound residues (BR) as a
"mechanistical" term related to the compound's fate. Bound residues describe the
fraction of a compound which is not available for extraction and uptake by organ-
isms without changing the chemical nature of the matrix. Formation of BR is thus
considered to be an irreversible sequestration process on the observed time scales.
Assuming that bound residues cannot be extracted at all by any extraction method
this fraction constitutes the lower boundary for the non-extractable residues. NER
are defined technically as the substance fraction which is not extracted when apply-
ing a certain extraction procedure to the investigated sample. This means that the
extraction procedure used determines the amount of non-extractable residues and
has to be specified together with the result.
Another problem is that bound residues may be formed from the parent compound
or from metabolites or degradation products with differing fractions depending on
substance and soil properties. This leads to a great uncertainty as far as the regu-
latory significance is concerned (Craven and Hoy, 2005). In particular, it is unclear
if the parent compound is immobilized directly or if the reaction proceeds via inter-
mediates that may be one of the known or unknown metabolites.
Bound residue formation from SDZ. Experimental results indicate that forma-
tion of BR from SDZ can be excluded during manure storage (Spiteller, 2007). In
soil and manure-amended soil experimental data show a fast decrease of extractable
SDZ and metabolites with half-lives of less than seven days (Kreuzig and H¨oltge,
2005; Schmidt et al., 2008; Amelung and Kaupenjohann, 2007). It has also been ob-
served that application of stronger extraction procedures (e.g. higher temperatures)
enhance the recovery of sulfonamides from spiked and manure-amended soils (Stoob
et al., 2006; Amelung and Kaupenjohann, 2007). Nevertheless, in the long run there
is a decrease in recovery even with the more effective extraction methods that is most
likely due to the formation of covalently bound residues. However, the mechanisms
that are responsible for emergence of NER and formation of BR are still not fully
understood (Matthies et al., 2008). Much stronger formation of bound residues was
observed in non-sterile soils than in sterile soils implicating an essential influence
of microbial activity on bound residue formation (Kreuzig and H¨oltge, 2005). Bialk
and Pedersen (2008) investigated the bound residue formation of sulfonamides with
humic acids in the presence of a fungal enzyme (peroxidase). They suggest covalent
binding between the amino group of the sulfonamide and humic substances that
CHAPTER 4. TRANSFORMATION REACTIONS
result in a deactivation of the antibiotic effect. In this case, Ac-SDZ cannot par-
ticipate in sequestration of sulfadiazine, whereas the hydroxylated metabolite with
its unchanged amino group may contribute to the bound residue formation. Since
hydroxylation does not alter the NH2-moiety, the various OH-metabolites are alsostill biologically active (Nouws et al., 1985) and thus of large interest to be included
in risk assessment. Thus, all of the various interconnected processes affecting fate
and effect of sulfonamides in soils have to be considered simultaneously to give a
complete picture.
The objective of this study is to elucidate the mechanisms affecting the observed
decrease of extractable SDZ and its metabolites over time in manure-amended soils.
Experimental data on the fate of SDZ in manure and manure-amended soils are
analyzed to identify the most relevant processes and reaction pathways. Influences
of manure application on the chemical fate of SDZ shall be identified.
Model development
Sulfonamide dynamics in manure and manure-amended soils are determined by a
number of different overlying processes that might influence each other and thus have
to be considered simultaneously in the model approach. The speciation equilibrium
of the compounds depends on the dissociation constants (pKa) and on solution
pH. The fraction of each species (cation, neutral, and anion) can be calculated as
described in Chapter 2. Transformation reactions from Ac-SDZ to SDZ and from
SDZ to OH-SDZ may occur during manure storage prior to application and in the
manure-amended soil. Since experiments in soil with and without manure did not
clearly show the effect of additional nutrients and microbial activity from the ma-
nure on apparent reaction rates (Spiteller, 2007; Schmidt et al., 2008) a possible
biomass influence on transformation kinetics is neglected. Reversible equilibrium
sorption of the compounds to solid matrices in manure and soil is described by the
apparent distribution coefficient K∗ which depends on the substance speciation and
on soil properties (Chapter 3). By the sequential extraction experiment three diffe-
rent fractions were determined (Amelung and Kaupenjohann, 2007) that have to
be assigned to process variables of the model. Here, we assume that the amount of
substance on the CaCl2 extract and the methanol extract is an appropriate measurefor the total substance mass in soil solution and the sorbed phase that has not yet
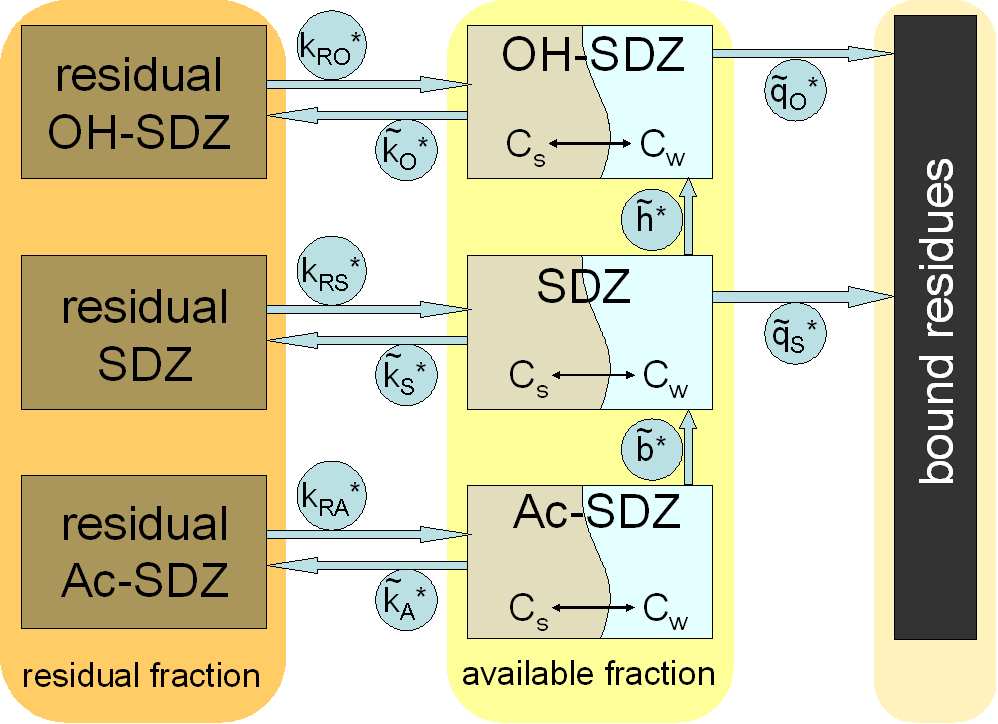
been subject to sequestration. The remaining residual fraction (RES) constitutes the
amount that has been sequestered by a reversible process requiring stronger condi-
tions for extraction. Bound residues constitute an ultimate sink for the chemicals
and their formation is regarded as an irreversible process in the model. Bialk and
Pedersen (2008) found that the covalent coupling of sulfonamide antibiotics most
likely proceeds between the amino group of the compound and humic substances.
Since Ac-SDZ is acetylated at this amino group the capability of the metabolite to
form such covalent bindings is inhibited and the respective pathway is not considered
in the model. The complete model structure for the fate of SDZ and its metabolites
Ac-SDZ and OH-SDZ in manure-amended soil is shown in Figure 4.1.
Model assumptions. Sulfadiazine and its metabolites can be regarded as an ad-
ditional carbon substrate for degrading organisms. However, compared to the total
Figure 4.1: Model structure for transformations of SDZ and its metabolites Ac-SDZ and OH-SDZ
in manure and soil. Processes include equilibrium sorption between dissolved (Cw) and sorbed (Cs)
state, reaction from Ac-SDZ back to SDZ with an apparent bulk rate constant ˜b∗, hydroxylation
from SDZ to OH-SDZ with an apparent bulk rate constant ˜
h∗, kinetically controlled translocation
with apparent bulk rate constants ˜
k∗ and apparent rate constants k∗, and formation of bound
residues (BR) with an apparent bulk rate constant ˜
CHAPTER 4. TRANSFORMATION REACTIONS
mass of carbon substrates in soil the compounds only serve as secondary substrates
and are transformed by "co-metabolism" (Matthies et al., 2008). Thus, all transfor-
mation reactions of SDZ, Ac-SDZ and OH-SDZ including the formation of BR are
assumed to follow first order kinetics. For the sake of simplicity, equilibrium sorption
is not explicitly included in the model. Although only the dissolved fraction in soil
solution is available for transformation, uptake, sequestration and BR formation,
the model uses the sum of dissolved and equilibrium sorbed fraction (called avail-
able fraction) as central state variable for sulfadiazine and its main metabolites.
This is justified, because the concentration in soil solution is related to the total
concentration by a constant factor which is determined by the apparent partition
coefficient K∗ and bulk soil properties such as dry soil density and volumetric water
content. Thus, all rate constants estimated below for the available fraction are bulk
rate constants (indicated by ∼) that implicitly consider the specific sorption equi-
librium in the investigated soil. Moreover, all rate constants have to be looked at as
apparent rate constants (indicated by ∗) that implicitly consider the speciation of
SDZ and its main metabolites.
According to the model structure given in Figure 4.1 the following system of ordi-
nary differential equations describes the fate of SDZ and its two main metabolites
in manure and manure-amended soil:
= ˜b∗ · A + k∗ · RS − ˜h∗ + ˜
= k∗ · RA − ˜b∗ + ˜
= ˜h∗ · S + k∗ · RO − ˜
dRS = ˜k∗ · S − k∗ · RS
dRA = ˜k∗ · A − k∗ · RA
dRO = ˜k∗ · O − k∗ · RO
dBR = ˜q∗ · S + ˜q∗ · O
S, A, and O are the concentrations of SDZ, Ac-SDZ and OH-SDZ in the available
fraction, RS, RA, and RO are the respective concentrations in the residual fraction,
and BR is the fraction of bound residues which cannot be further differentiated. All
concentrations are given in µmol kg−1 dry soil to assure consistency of the model
simulations. ˜b∗ is the apparent bulk rate constant for the transformation from Ac-
SDZ back to SDZ, ˜h∗ is the apparent bulk rate constant describing hydroxylation
from SDZ to OH-SDZ, ˜
q∗ are the apparent bulk rate constants for the for-
mation of bound residues from SDZ and OH-SDZ, respectively. The apparent bulk
rate constants ˜
k∗ describe the transfer kinetics into the residual fraction
are the apparent rate constants for the reverse process.
Model parameterization and sensitivity analyses. Independent data for the
kinetic rate constants are not available from the literature. Storage experiments with
contaminated manure from feeding experiments under aerobic and anaerobic condi-
tions revealed that OH-SDZ remains unchanged and formation of bound residues in
manure can be neglected (< 2%) (Spiteller, 2007). A rough estimation of the rate
constant for the de-acetylation of Ac-SDZ to SDZ in manure as the predominant
process was made from the data. As far as the fate of SDZ in soil is concerned, all
kinetic constants were deduced by fitting the model to the experimental data from
Amelung and Kaupenjohann (2007) in soils from Kaldenkirchen and Merzenhausen.
Also, the model was fitted to adapted data with a reduced amount of OH-SDZ in
the residual fraction resulting from a correction according to the the mass balance
(subsection 1.3.4) and compared with results derived from the original data set.
Least-squares fitting was performed with the computer software Scientist for Win-
dows Version 2.014. Initial conditions (t = 0 d) for the state variables S, A, O, RS,
RO and BR were inversely calculated and optimized in analogy to the rate constants
since measured data are only available for day 1. The initial condition RA for the
residual fraction of Ac-SDZ was set to zero due to insensitivity of the fitting proce-
dure against the small values of Ac-SDZ measured in the residual fraction. Scenario
analyses were conducted to identify transformation pathways of minor importance
that can be omitted in the model without deteriorating model performance.
For model comparison the model selection criterion (MSC) was chosen which does
not only consider deviations from experimental data but also the number of fitted
parameters. This criterion is a modification of the Akaike Information Criterion
(Akaike, 1976) representing the information content of the parameter set p resulting
from the fit of the model to n observations:
i=1 wi · (Yobs,i − Ycalc,i)2
The data set was weighted with the respective inverse value (i.e. wi = Y −1 ) in
order to consider deviations from data with low values equally to data with higher
CHAPTER 4. TRANSFORMATION REACTIONS
values. M SC is the largest for the most appropriate model. To verify the validity
of the model approach data from another independent experiment were compared
with simulated data using the same parameter set derived from fitting.
Results and Discussion
Fit to manure extraction data
The simplified model approach describing the chemical fate of sulfadiazine in manure
includes the reaction of Ac-SDZ back to SDZ but neglects all other transformation
processes. This results in the following reduced system of ordinary differential equa-
dA = −˜b∗ · A
With initial conditions S(0) = S0 and A(0) = A0 the analytical solution is as
This solution is fitted to measured concentrations of SDZ and Ac-SDZ during manure
storage under anaerobic conditions at 20◦C (Spiteller, 2007). The apparent bulk
rate constant ˜b∗ is determined resulting in a half life of 30 days (r2=0.996). Data
points and model simulations are shown in Figure 4.2 demonstrating the continuous
decrease of Ac-SDZ whereas the concentration of SDZ increases. These observations
are comparable to experimental results from Grote et al. (2004) which lead to a half
life for reaction of Ac-SDZ to SDZ of 15.5 days under aerobic conditions.
Fit to extraction data of soils amended with fresh ma-
The general fate model was fitted to data sets from experiments in soils Kaldenkirchen
and Merzenhausen (Amelung and Kaupenjohann, 2007) with goodness-of-fit criteria
of r2 = 0.99 and MSC = 4.2 - 4.6. Various scenarios assuming different model struc-
tures showed that a best fit for SDZ, Ac-SDZ and OH-SDZ in the three fractions
4.3. RESULTS AND DISCUSSION
Figure 4.2: Measured data (Spiteller, 2007) of sulfadiazine (SDZ), N4-acetyl-sulfadiazine (Ac-
SDZ) and 4-hydroxy-sulfadiazine (OH-SDZ) and simulated concentrations (lines) of SDZ and Ac-
SDZ in manure. Model results are based on a least-squares fit to experimental data.
(available, residual, bound residues) could be achieved assuming SDZ and OH-SDZ
to form bound residues out of the available fraction and not via the residual fraction.
In both soils which were amended with fresh manure kinetic constants resulting from
a least-squares fit were of the same order of magnitude (Table 4.1). Half-lives for
the de-acetylation were estimated to be four to five days which is a factor of four
faster than observed in manure under aerobic conditions (Grote et al., 2004). Hy-
droxylation of SDZ is negligible in both soils as estimated rate constants are small
(˜h∗ → 0). This is consistent with observations in manure where neither formation
nor degradation of OH-SDZ occurred during storage (Schmidt et al., 2008; Heuer
et al., 2008).
These results suggest that soil type does not affect the chemical fate of SDZ and its
metabolites significantly. Figures 4.3 and 4.4 demonstrate measured and simulated
concentrations of SDZ, Ac-SDZ and OH-SDZ after fitting the model to extraction
data from Amelung and Kaupenjohann (2007). It can be seen that the model fit is
CHAPTER 4. TRANSFORMATION REACTIONS
Table 4.1: Kinetic rate constants resulting from a least-squares fit of the model (Figure 4.1) to
extraction data of soils Kaldenkirchen and Merzenhausen (data from Amelung and Kaupenjohann
(2007)) after application of fresh (3 weeks) manure.
parameter Kaldenkirchen Merzenhausen
able to explain the data well. An important result is the fact that the relocation
of the three compounds in the residual fraction must be reversible to explain the
available data. The transfer of SDZ, Ac-SDZ and OH-SDZ into the residual fraction
is of the same order of magnitude for all of the three compounds indicating that
this transfer may be a diffusion-controlled physical entrapment. Relocation, how-
ever, is one order of magnitude slower for SDZ and OH-SDZ, but remains in the
same range for Ac-SDZ. Since SDZ and OH-SDZ form bound residues they may also
be sequestered more strongly in the residual fraction whereas the reversibility for
Ac-SDZ is not decelerated by additional binding processes in the residual fraction.
However, the processes governing sequestration of Ac-SDZ proved to be insensitive
to the model outcome because of the small amounts of Ac-SDZ present.
Experimental data also suggest that transfer of the compounds into the residual
fraction occurs on a similar timescale as compared to bound residue formation. Thus,
these sequestration processes dominate the substance's fate. In the long run, Ac-SDZ
disappears rather fast, whereas SDZ and the hydroxylated metabolite can still be
found in the available fraction seven month after manure application to soils. Since
these two substances are both antibiotically active they may affect soil microbial
organisms and soil functions over a long timescale.
4.3. RESULTS AND DISCUSSION
Figure 4.3: Measured (Amelung and Kaupenjohann, 2007) and simulated concentrations (µmol
kg−1, d.w.) of sulfadiazine (SDZ), N4-acetyl-sulfadiazine (Ac-SDZ) and 4-hydroxy-sulfadiazine
(OH-SDZ) in soil Kaldenkirchen amended with fresh manure in the (a) available and (b) resid-
ual as well as bound residue (BR) fraction. Model results are based on a weighted least-squares fit
to experimental data.
CHAPTER 4. TRANSFORMATION REACTIONS
Figure 4.4: Measured (Amelung and Kaupenjohann, 2007) and simulated concentrations (µmol
kg−1 (d.w.)) of sulfadiazine (SDZ), N4-acetyl-sulfadiazine (Ac-SDZ) and 4-hydroxy-sulfadiazine
(OH-SDZ) in soil Merzenhausen amended with fresh manure in the (a) available and (b) residual
as well as bound residue (BR) fraction. Model results are based on a weighted least-squares fit to
experimental data.
4.3. RESULTS AND DISCUSSION
Another important factor is the problem of the initial conditions. Since the first
sample was taken approximately 24 hours after the start of the experiments the
initial concentrations of the compounds in the fractions were not known. Data sug-
gest that significant amounts of SDZ and OH-SDZ have to be already present in the
residual and in the bound residue fraction immediately after starting the experiment
indicating that this transfer is due to a fast process already occurring during sample
preparation (i.e. homogenization of the soil-slurry mixture).
Evaluation of the model
For a first evaluation of the validity of the model outcome, independent experimental
data of amending aged manure (after 6 month of storage) to soil Kaldenkirchen were
used. Again, SDZ and the hydroxylated metabolite persist in the available fraction
seven month after manure application to soil. In the aged manure, the amount of
Ac-SDZ is negligible, because it has been completely transformed back to SDZ dur-
ing storage. Thus, rate constants related to the fate of Ac-SDZ (˜b∗, ˜
be derived. However, experimental data cannot be explained by the same parameter
set extracted from the experiments with fresh manure. Especially the concentrations
in the available fraction are underestimated by the model between day 30 and 120
after manure application (Figure 4.5).
Thus, the model was independently fitted to the data (r2 = 0.98; MSC = 4.5) re-
sulting in a much better agreement (Figure 4.6). Kinetic parameters describing the
interchange between the available and the residual fraction (˜
k∗ and k∗) as well as
the formation of bound residues via SDZ (˜
q∗ ) are of the same order of magnitude as
for the experiments with fresh manure. Again, hydroxylation of SDZ is slow, but a
slower BR formation via OH-SDZ as compared to the fresh manure data is simulated
(Table 4.2).
It can be seen from Figures 4.5 and 4.6 that standard deviations of measured OH-
SDZ concentrations in the residual fraction were quite large (up to 50%) indicating
severe analytical uncertainties. Over-estimation of the OH-SDZ caused by matrix
effects could not be completely excluded and is subject to ongoing verification tests
by the project partners. Indeed, the mass balance established by comparison with
total radioactivity measurements (see subsection 1.3.4) revealed a significant differ-
ence in the residual fractions with too large values for the sum of SDZ, Ac-SDZ
and OH-SDZ. Thus, OH-SDZ concentrations in the residual fractions were arbitrar-
ily adapted for all experiments based on this mass balance. Adapted data from all
CHAPTER 4. TRANSFORMATION REACTIONS
Figure 4.5: Measured data (Amelung and Kaupenjohann, 2007) and simulated concentra-
tions (µmol kg−1, d.w.) of sulfadiazine (SDZ) and 4-hydroxy-sulfadiazine (OH-SDZ) in soil
Kaldenkirchen amended with aged manure in the (a) available and (b) resdiual as well as bound
residue (BR) fraction. Model results are based on the data set derived from investigations of soil
amended with fresh manure (Table 4.1).
4.3. RESULTS AND DISCUSSION
Figure 4.6: Measured data (Amelung and Kaupenjohann, 2007) and simulated concentra-
tions (µmol kg−1, d.w.) of sulfadiazine (SDZ) and 4-hydroxy-sulfadiazine (OH-SDZ) in soil
Kaldenkirchen amended with aged manure in the (a) available and (b) residual as well as bound
residue (BR) fraction. Modelling results are based on a weighted least-squares fit to experimental
CHAPTER 4. TRANSFORMATION REACTIONS
Table 4.2: Kinetic rate constants resulting from a least-squares fit of the model (Figure 4.1)
to original and adapted extraction data of soil Kaldenkirchen (original data from Amelung
and Kaupenjohann (2007)) after application of fresh (3 weeks) and aged manure (6 month). The
measured overestimation of OH-SDZ in the residual fraction was adapted to the mass balance on
parameter fresh manure
three scenarios were again fitted to the model (r2 = 0.98 - 0.99; MSC = 3.8 - 4.5).
Only slight differences in the parameter values for the two experiments with fresh
manure occurred (data not shown for soil Merzenhausen). In the experiment with
aged manure the results show a more consistent picture if the model was fitted to
the adapted OH-SDZ data in the residual fraction (Figure 4.7 and Table 4.2). Here,
parameter values for SDZ and Ac-SDZ remain virtually unchanged and parameters
describing the translocation of OH-SDZ into the residual fraction are of the same
order of magnitude (˜
k∗ , k∗ ). In agreement with the results of the fresh manure
experiments, hydroxylation is negligible and BR formation is now predicted with a
bulk rate constant similar to those of the fresh manure data. Since parameter values
for the fate of SDZ and its metabolites in soil are in the same range regardless of
the manure's age, it can be concluded that manure storage does not significantly
influence the SDZ kinetics in soil.
4.3. RESULTS AND DISCUSSION
Figure 4.7: Measured data (Amelung and Kaupenjohann, 2007) and simulated concentra-
tions (µmol kg−1, d.w.) of sulfadiazine (SDZ) and 4-hydroxy-sulfadiazine (OH-SDZ) in soil
Kaldenkirchen amended with aged manure in the (a) available and (b) residual as well as bound
residue (BR) fraction. Modelling results are based on a weighted least-squares fit to experimental
data adapted for OH-SDZ to the mass balance on radioactivity.
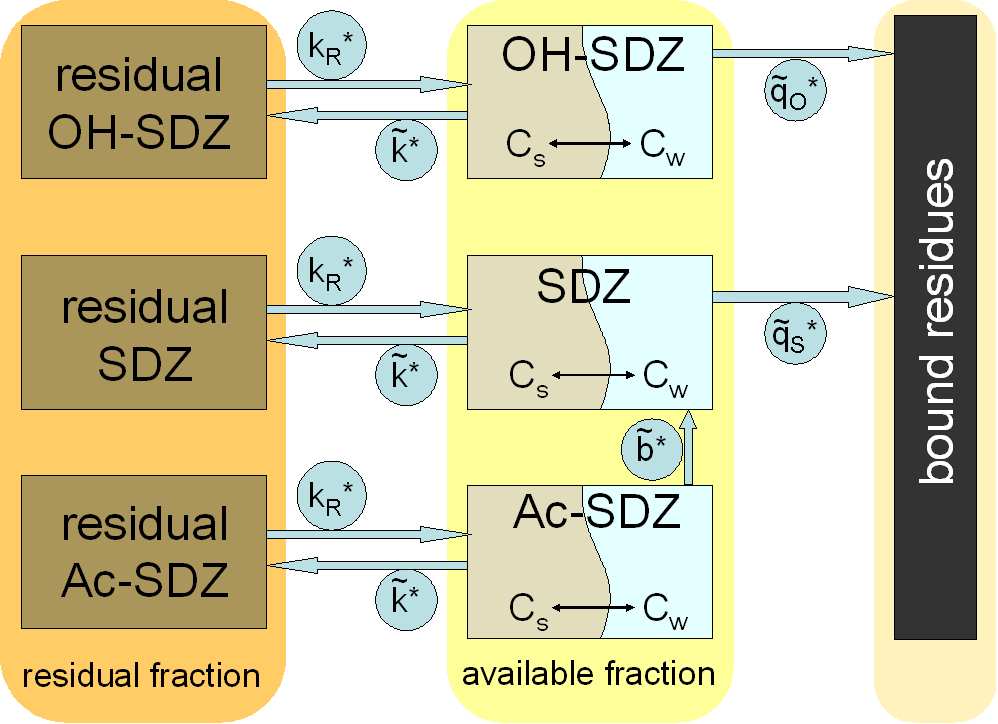
CHAPTER 4. TRANSFORMATION REACTIONS
Simplification of the model
Summarizing the parameter results of the fitting procedures and according to the
observed fate of SDZ and its metabolites in soils Kaldenkirchen and Merzenhausen
the initial model presented in Figure 4.1 can be simplified from ten to five kinetic
constants (Figure 4.8). On the observed timescale and in the investigated soils,
hydroxylation of SDZ can be neglected (˜h∗ = 0). However, a sensitivity analysis on
the kinetic rate constant ˜h∗ showed that hydroxylation may proceed up to an order of
magnitude of 1E-03 d−1 without deteriorating the simulation results. The respective
half-life of about two years out-scored by the much faster formation of bound residues
and is thus insensitive in the model. The interchange of the compounds between the
available and the residual fraction occurs with the same parameter values (˜
k∗ ; k∗ := k∗
= k∗ ). Based on the available extraction data
Figure 4.8: Simplified model structure for transformations of SDZ and its metabolites Ac-SDZ
and OH-SDZ in manure and soil. Hydroxylation from SDZ to OH-SDZ can be neglected. Processes
still include reaction from Ac-SDZ back to SDZ with an apparent bulk rate constant ˜b∗, kinetically
controlled translocation with generalized apparent bulk rate constant ˜
k∗ and an apparent rate
constant k∗ for all compounds and formation of bound residues (BR) with apparent bulk rate
Table 4.3: Kinetic rate constants resulting from a least-squares fit of the simplified model
(Figure 4.8) to adapted extraction data of soils Kaldenkirchen and Merzenhausen (original data
from Amelung and Kaupenjohann (2007)) after application of fresh (3 weeks) and aged manure
parameter Kaldenkirchen Merzenhausen Kaldenkirchen
the state variable for the residual fraction of Ac-SDZ may also be neglected since
its amount in the corresponding extract tends to zero. Consequently, the model is
insensitive when fitting the respective kinetic constants (k∗ = ˜
k∗ ) to the extraction
data. Mechanistically, however, there is no evidence that Ac-SDZ should behave
differently as far as the relocation into the residual fraction is concerned and thus,
it is still included in the mechanistic model approach. The values of the resulting
kinetic constants (Table 4.3) can be deduced by fitting the model to the adapted
data without loss of goodness (r2 = 0.97 - 0.99; MSC = 3.3 - 4.7) and thus confirm
the assumptions on the fate of SDZ and its main metabolites in soil.
Comparison of experimental data on the fate of SDZ and its metabolites in manure-
amended soil with model scenarios helped to elucidate the most relevant transforma-
tion pathways and parameters. Rapid decrease of the Ac-SDZ metabolite to SDZ and
significant formation of bound residues from SDZ as well as the OH-SDZ metabolite
are the dominant transformation reactions, while hydroxylation of SDZ seems to be
of minor importance under environmental conditions. Besides equilibrium sorption
that has not been investigated in more detail another reversible process was iden-
tified which leads to a kinetically controlled reduction of the immediate available
fractions of SDZ and at least the hydroxylated metabolite. This translocation into a
so-called residual fraction may serve as a long-term reservoir for degradation, uptake
CHAPTER 4. TRANSFORMATION REACTIONS
into plants and soil organisms, inhibitory effects on soil bacteria and formation of
bound residues.
Thus, the results indicate a possible long-term relevance of the hydroxy-metabolite.
Knowledge about the fate of hydroxylated metabolites in comparison to their parent
compound is scarce and is only available for pesticides like atrazine. For example,
Seybold and Mersie (1996) as well as Moreau and Mouvet (1997) point out that
the hydroxy functional group leads to a higher sorption of hydroxylated atrazine to
soils. This is confirmed by investigations of Kruger et al. (1996) who suppose that
the additional OH-group may increase binding reactions. Thus, it is essential to in-
clude individual sorption experiments for the acetyl- and the hydroxy-metabolite to
elucidate the contribution of the functional groups to the environmental fate of sul-
fadiazine in soil. The mechanism behind the reversible translocation process has not
yet been identified. An explanation may be a slow diffusion process in soil leading
to a physical entrapment of the compounds in small pores. This is also indicated
by similar kinetic constants for the three investigated compounds SDZ, Ac-SDZ
and OH-SDZ. Two different models have already been proposed by Pignatello and
Xing (1996): The first one describes an intraparticulate diffusion through organic
matter (in combination with physical entrapment), whereas the second is charac-
terized by a sorption-retarded diffusion in soil micropores. Presumably, both model
approaches are involved and can explain the "residual fraction". Determination of
sorption enthalpies may help to gain more insight into sorption mechanisms. As far
as sequestration is concerned the developed fate model can be compared to sorption
models proposed by Wehrhan et al. (2007). There, breakthrough curves describing
transport of SDZ in soil columns were best fitted with a sorption model assuming
three sorption sites whereof two were reversible kinetically controlled sorption sites
and one was an irreversible sorption site (i.e. five kinetic rate constants). Metabolites
could be neglected since SDZ was spiked to the soil columns and, according to the
results presented above, seem not to be generated or degraded in significant amounts
in soil. Explicitly, the general fate model described above is based on one irreversible
sorption process for SDZ and OH-SDZ as well, i.e. the formation of bound residues
by covalent binding of the amino group to humic acids. A reversible kinetically con-
trolled process is the back and forth transfers of SDZ, OH-SDZ and Ac-SDZ from
the available fraction into the residual fraction. A second reversible sorption process
is implicitly considered by the equilibrium sorption described by the apparent parti-
tion coefficient K∗ which is included in the apparent bulk degradation rate constants.
Consequently, the developed mechanistic fate model includes two sorption sites and
three kinetic rate constants and is equivalent to the model description in the soil
columns. In general, the model structure is transferable to describe the environmen-
tal fate of other sulfonamides and also of other compound classes. In dependence of
the respective metabolism and sequestration behaviour state variables and kinetic
rate constants would have to be adapted.
After all, the amount of solute in pore water is the central state variable to link
chemical fate to the biological effects. With the developed chemical fate model the
total concentration in the available fraction can be predicted over time. Using equa-
tion 3.8 the respective pore water concentration can be simply calculated from K∗dand the actual water content. The effect for a certain end point provoked by this
concentration is dependent on the regarded organism and its habitat, e.g. the antibi-
otic activity depends on the mode of action of the compound. The reaction of the
effective compounds (SDZ and OH-SDZ) with the enzyme DHPS in the bacterial cell
in competition to the natural substrate para-aminobenzoic acid interferes with the
folic acid cycle and, consequently, inhibits bacterial growth. Thus, for sulfonamides
the link between chemical fate and biological effect is realized by the uptake of the
antibiotic from solution by bacteria and a subsequent accumulation in the bacterial
cell. The applied model concept and comparison of simulation results with measured
effect data is described in the following chapter.
Uptake of Sulfonamides by
Sulfonamides interfere with the folic acid cycle in microorganisms by competing for
one of the key enzymes, dihydropteroate synthase (DHPS). As a result the produc-
tion of folic acid is reduced which leads to a complete inhibition of cell reproduction
(Brown, 1962; Vinnicombe and Derrick, 1999). Therefore, sulfonamides are charac-
terized as broadband antibiotics in pharmacotherapy. Use of growth regulators in
livestock feeding is interdicted since 2006 according to the EC directive 1831/20032,
but sulfonamides are still applied in large amounts in veterinary medicine (Thiele-
Bruhn, 2003; Kreuzig et al., 2003; Schneidereit, 2006). Sulfonamides have to be
transported into the cell to compete with p-aminobenzoic acid (pABA) for the en-
zyme DHPS. It is known that the antibiotic effect of sulfonamides on cell activity
differs depending on substance properties and pH conditions. Mengelers et al. (1997)
investigated the effect of several sulfonamides on Actinobacillus pleuropneumoniae,
a pathogenic bacterium causing pig respiratory disease (Marsteller and Fenwick,
1999), and observed that the minimal inhibitory concentration (MIC) varied with
the pKa2 of the substance and the extracellular pH. Thiele-Bruhn and Beck (2005)showed that effective doses for the suppression of the microbial iron(III)-reduction
in soils also vary depending on the speciation of the antibiotics that is influenced
1 Reprinted in parts from Zarfl et al. (2008b), Copyright (2007), and Tappe et al. (2008), Copy-
right (2008), with permission from Elsevier.
2 Regulation (EC) No 1831/2003 of the European Parliament and of the Council of 22 September
2003 on additives for use in animal nutrition. Official Journal of the European Union L 268/29-43.
CHAPTER 5. UPTAKE OF SULFONAMIDES BY MICROORGANISMS
indirectly by the soil pH. Therefore, the pH-dependent uptake and accumulation of
sulfonamides in bacterial cells is of particular interest not only for human and veteri-
nary chemotherapy but also for the assessment of fate and effects of pharmaceuticals
in the environment. In this chapter, the influence of the speciation on the potential
intracellular accumulation of sulfonamides is investigated. For this purpose a re-
cently published mechanistic model for the transport of chemicals into cells (Trapp
and Horobin, 2005) has been adapted and supplemented. The model is based on the
assumption that not only neutral molecules but also the dissociated species are able
to pass the cell envelope. Critical model parameters are identified and their sensitiv-
ity on model results is tested within realistic parameter ranges. Finally, enzymatic
reactions are integrated into the uptake model to simulate the competitive effect of
sulfonamides on pABA and to assess the impact of the additional process within
realistic parameter ranges. The aim of this investigation is to find out in how far the
observed substance-specific and pH-dependent antibiotic effects can be explained by
a different accumulation of sulfonamides in bacterial cells.
Transport into bacterial cells
To affect a bacterial microorganism, the solute substance molecules have to pass
the cell envelope and enter the cytoplasm. Including cell wall and plasma membrane
the cell envelope can already be regarded as an intrinsic drug barrier. As far as
permeability of biological membranes is concerned four different uptake mechanisms
can be differentiated (Rang et al., 2007):
• diffusion through the lipid bilayer
• diffusion through porins formed by proteins
• transmembrane transport proteins which bind a molecule on one side of the
membrane, then change their conformation and leave the molecule on the other
side of the membrane
• endocytose: uptake of small amounts of liquid including solved (pinocytose) or
solid (phagocytose) substances.
Non-polar substances mainly diffuse through the cell membrane influenced by two
physicochemical parameters, the partitioning coefficient between lipid and water
(KOW ) and the diffusion coefficient which hardly varies between different pharma-
ceuticals (Rang et al., 2007). For sulfonamides active efflux transport systems have
been identified (K¨ohler et al., 1996) but so far no specific transport protein for sul-
fonamide influx is known (Schrempf, 2007). Although active transport by unspecific
carrier mechanisms cannot be ruled out, uptake kinetics of organic contaminants by
bacteria are usually regarded as diffusive transfer across the cell membranes (Nouws
et al., 1985; Hancock, 1997; Kitahara et al., 1997; Tras et al., 1998). The ratio of
substance concentrations in the cell (Ccell) and the surrounding medium (Cenv) can
be regarded as a measure for the maximal accumulation of the antibiotic in the
cell. Equation 5.1 describes a simple relationship for this accumulation assuming
that uptake is solely determined by mass flux of the neutral molecule, while charged
species cannot cross the cell envelope barrier (B¨
1 + 10pHcell−pKa2
1 + 10pHenv−pKa2
However, this is a strong assumption that is not necessarily valid (Rang et al., 2007).
Recently, Trapp and Horobin (2005) published a mechanistic model assuming total
uptake to be the sum of mass fluxes of the neutral and charged species of the
molecule. In their model, the flux of the neutral molecule across the cytoplasmic
membrane is driven by the chemical potential gradient and can be specified by
Fick's first law of diffusion (Atkins (1990), p.735):
Jn = −Pn · (acell,w,n − aenv,w,n)
where Jn is the net flux with the concentration gradient from the outside into the
cell in mol · m−2 · s−1, Pn is the permeability of the membrane for neutral (n)
molecules in m · s−1, and acell,w,n and aenv,w,n are the activities of the dissolved (w)
neutral molecules (n) in the cell (cell) and in the surrounding environment (env),
The flux of ions across electrically charged membranes is more complex since it
depends on the chemical potential as well as on the electrochemical potential. The
assumption of a linear potential gradient across the membrane then leads to the
Nernst-Planck equation (Briggs et al., 1961):
cell,w,d · eN − aenv,w,d
where Jd is the net flux of the dissociated (d) species (cationic and anionic, respec-
tively) in mol · m−2 · s−1, Pd is the permeability of the membrane for an ion, acell,w,d
CHAPTER 5. UPTAKE OF SULFONAMIDES BY MICROORGANISMS
and aenv,w,d are the activities of the dissolved (w) dissociated species in the cell and
in the surrounding environment, respectively. The constant N is defined as follows:
with z being the electric charge (+ 1 for cations, - 1 for anions), E the membrane
potential, F the Faraday constant (96484.56 C mol−1), R the universal gas constant
(8.314 J ·mol−1·K−1), and T the absolute temperature (K). The membrane potential
E for E. coli is about -0.11 V (Zilberstein et al., 1984).
In general, it is assumed that the permeability of the cell envelope is significantly
lower for charged molecules than for neutral molecules (Pd < Pn) but cannot be
neglected (Escher et al., 1999). If we additionally assume that the reaction kinetics
of sulfonamides in the cell is much slower than the uptake kinetic, steady-state
accumulation in the cell can be estimated by solving the following system of ordinary
differential equations describing the change of total substance concentration in a
single cell (Ccell) and the total substance concentration in the surrounding matrix
(Cenv), both in mol · m−3.
n · γn · fcell,w,n − Pc ·
c · fcell,w,c · eNc
a · fcell,w,a · eNa
env · (Pn · fenv,w,n
= − cells · Vcell ·
Pc and Pa are the permeabilities of the cell membrane for the cationic (c) and the
anionic (a) species, respectively, Nc and Na are defined as above with z = +1 or
−1, Venv is the total volume of the environment, Acell is the cell surface, Vcell is
the cell volume of a bacterium, and ncells is the total number of bacterial cells in
the environment. fcell,w,i and fenv,w,i are the fractions of the dissolved cationic (c),
anionic (a) and neutral (n) species of the total substance mass in the cell and in
the surrounding medium, respectively. γi is the activity coefficient of the respective
species in the cell which relates concentration to activity according to acell,w,i =
γi · Ccell. Replacing the terms Pn · γn · fcell,w,n + Pc · Nc · γ
c · fcell,w,c · eNc + Pa ·
γa · fcell,w,a · eNa by a "release velocity" kr and Pn · fenv,w,n + Pc · Nc · f
env,w,a by an "uptake velocity" ku in equation 5.5 leads to the following
simplified differential equation for the cell concentration:
r · Ccell + ku · Cenv ]
Note that kr and ku are in units of m·s−1. With dCcell = 0 the ratio of C
at steady state is given by the ratio of uptake and release rate:
This concentration ratio describes the potential accumulation of the substrate in
the cells relative to their surroundings and is defined as the accumulation factor
AF (eq. 5.8). It is commonly supposed that the antibiotic effect of sulfonamides
is solely due to the anionic sulfonamide species (Henry, 1943), because of its close
structural similarity to the original substrate p-aminobenzoate. Thus, to relate sul-
fonamide accumulation to growth inhibition of bacterial cells, accumulation of the
anionic species in the cells is the relevant parameter. This is described by the anion
accumulation factor (AAF) which relates the effective concentration (intracellular
anion concentration) to the applied dose (total sulfonamide concentration in the
a,cell = AF · αa,cell
The fraction of anion in the cell and thus also the AAF depend on the internal pH
and the pKa2 of the substance.
Model parameterization and sensitivity analyses of the
Sulfonamides accumulate in the particle-free cytoplasm (=liquid phase) of the cells,
as it was shown for sulfadiazine in Escherichia coli cells (B¨
uttner, 1972).
Thus, intracellular sorption to lipids and other compounds can be neglected. Fur-
thermore, external and intracellular pH values are constant over time and speciation
is in equilibrium described by equations 2.1-2.6. From equation 2.1 it follows that
the cationic species can be neglected as the pKa1 of sulfonamides is several orders ofmagnitude below environmentally relevant pH values. Permeabilities of the cell en-
velope are estimated analogously to Trapp (2004) from the octanol-water partition
coefficient (KOW ) and a combination of estimated cell envelope thickness ∆x for bac-
teria (20 nm) and an average diffusion coefficient D for neutral organic compounds
CHAPTER 5. UPTAKE OF SULFONAMIDES BY MICROORGANISMS
in biomembranes of 10−14 m2 · s−1(Lieb and Stein, 1971):
log Pn = log KOW + log
The permeability of biomembranes for organic ions is approximately 3.5 orders of
magnitude smaller than for neutral molecules (Raven, 1975; Trapp and Horobin,
log Pd = log KOW − 10.2
Substance properties for a variety of sulfonamides were collected from the literature
and are summarized in Table 2.1. Measured log KOW values for several sulfonamides
range from -1.22 (sulfaguanidine) up to 1.63 (sulfadimethoxine) resulting in Pn values
from 3.0 · 10−8m · s−1 to 2.1 · 10−5m · s−1.
A reference scenario is chosen representing neutral extracellular conditions (pH =
7.0) and a typical pH value of 7.5 for bacterial cytoplasm (Zilberstein et al., 1984;
Beales, 2004). The permeabilities are calculated for an intermediate log KOW (0.0)
resulting in values of 5.0 · 10−7m · s−1 (Pn) and 1.6 · 10−10m · s−1 (Pd), respectively.
With the ionic strength I of the cytoplasm of E. coli of about 0.3 mole (Weiden
et al., 1967) the activity coefficient γn of the neutral molecule in the cytoplasm can
be estimated from the Setchenov equation to be 1.23. The activity coefficient for the
monovalent cation and anion ( z = 1) is calculated with the Davies approximation to
0.74 (γc = γa) at I=0.3 mole (Appelo and Postma, 1999). Extracellular sulfonamide
concentrations were not corrected for ionic strength, i.e. activities were assumed
to be equal to concentrations. Sensitivity analyses are performed for the effect of
intracellular and extracellular pH as well as for the permeability difference between
neutral and ionic sulfonamide species.
Development of the model for competitive enzymatic
As described in section 1.1 sulfonamides represent a structural analogue to para-
aminobenzoic acid (pABA) which is necessary for the synthesis of folic acid. Thus,
they act as competitive antagonists in the reaction of pABA with the enzyme dihy-
dropteroate synthase (DHPS) and inhibit the production of dihydropteroate (DHP)
which is a co-factor for the formation of folic acid. This enzymatic reaction can
be described according to the well-known Michaelis-Menten equation for both the
enzymatic reaction with pABA and the one with the sulfonamide:
↽ ES → E + DHP ∗
↽ EP → E + DHP
E is the concentration of the enzyme DHPS, S and P are the concentrations of the
sulfonamide and of pABA, respectively. The pABA concentration is assumed to be
in steady state, i.e. the total available amount of pABA in the cell is not affected by
any enzymatic reaction (dpABA = 0). ES and EP are the respective concentrations
of the enzyme-substrate complex and DHP ∗ is the DHP analogue. Additionally,
for enzyme reactions it can be assumed that the total amount of enzyme is constant
and that the enzyme-substrate complexes reach quasi-stationarity:
Then, the change of product and sulfonamide concentrations is described by the
following kinetic equations:
With the condition (a) equation 5.15 can be solved for ES:
For equation 5.16 it holds accordingly:
CHAPTER 5. UPTAKE OF SULFONAMIDES BY MICROORGANISMS
Km,S and Km,P are the Michaelis constants for the sulfonamide and for pABA,
Inserting the right-hand side of equation 5.19 into equation 5.18 leads to:
E0 − (E0−ES)·P · S
(Km,P + P ) · (Km,S + S)
To solve for ES, all terms including this variable are separated on the left-hand side
of the equation resulting in:
Km,P · Km,S + Km,P · S + Km,S · P
Replacing ES in equation 5.14 with this term leads to:
Analogously, insertion of equation 5.18 in equation 5.19 leads to:
Vmax,S and Vmax,P are the maximum velocities for the enzymatic reaction of the sul-
fonamide and of pABA, respectively, and the terms Km,S ·P and Km,P ·S characterize
the respective competition between pABA and sulfonamide for the enzyme DHPS.
Equation 5.20 describing the concentration change of the sulfonamide by the enzy-
matic reaction can now be amended to the uptake model as additional loss term
for Ccell in equation 5.7 where the sulfonamide concentration S reflects the effective
Figure 5.1: Structure of the model integrating uptake of sulfonamides into the bacterial cell and
the subsequent enzymatic reaction in competition to pABA.
anionic fraction αa, only. The steady state of the uptake model which is described
by the relation of uptake and release rate (AF) changes with the integration of the
enzymatic equations to:
m,S +αa·Ccell+ K
In this way, an additional dependency of AFe on the intracellular sulfonamide con-
centration (Ccell) is introduced. The AF (without considering the enzymatic reac-
tion), in contrast, remains unaffected by the amount of applied substance concentra-
tion (5.8). Figure 5.1 illustrates the integration of the uptake model with enzymatic
reactions of pABA and the sulfonamide.
Parameterization of the enzymatic sub-model
In order to parameterize the enzymatic sub-model available literature on dihy-
dropteroate synthase (DHPS) and on the impact of sulfonamides as substrates for
the enzyme was investigated. For pABA kinetics enzymatic parameter values are
given with Km,P = 31.5 nmol · L−1 (Roland et al., 1979) and Vmax,P = 10.5 nmol ·
L−1 · s−1 (Talarico et al., 1991). Assuming an intact bacterial cell the concentration
of pABA is in the range of the Michaelis constant for half saturation (Km,P ) and is
varied for sensitivity analysis of the model within one order of magnitude around
the value for Km,P , i.e. in the range of 3.15 to 315 nmol · L−1. For sulfonamides re-
spective parameters can be estimated from experimental data from Swedberg et al.
CHAPTER 5. UPTAKE OF SULFONAMIDES BY MICROORGANISMS
(1979), Roland et al. (1979) and Talarico et al. (1991) in relation to parameters for
pABA. This results in a parameter range of [0.45 − 0.75]·Vmax,P (Roland et al., 1979;
Swedberg et al., 1979) for the maximum velocity Vmax,S and in [0.05 − 0.55] · Km,P
(Talarico et al., 1991; Swedberg et al., 1979) for the Michaelis constant Km,S. Simu-
lations of the AFe with varying enzymatic parameter values under different sulfon-
amide concentrations are compared with the original AF to identify the impact of
the enzymatic reaction on the intracellular substance accumulation.
Results and Discussion
Accumulation of sulfonamides in bacterial cells
Dynamic simulations with the uptake model revealed that steady state is reached
within a few minutes for all compounds, if permeabilities are calculated for -1.2 < log
KOW < 1.6 according to equations 5.10 and 5.11. In analogy, Kitahara et al. (1997)
observed a rapid accumulation of ciprofloxacin in cells of the widespread bacterium
Pseudomonas aeruginosa reaching equilibrium after 3 to 38 minutes in dependence
of temperature (37◦C and 17◦C, respectively).
To test if uptake of sulfonamides into bacterial cells dominates enzymatic reaction
kinetics in the cell, velocities of both processes have to be estimated. Swedberg
et al. (1979) determined maximal enzymatic reaction velocities (Vmax) of sulfathi-
azole (STZ) in relation to the amount of proteins resulting in 0.265 to 0.333 nmol
STZ per minute and mg protein. With a protein content of 55% of which 10% are
able to transform para-aminobenzoic acid, and with a cellular mass of 2.80 · 10−10
mg (Moat and Foster, 1995) Vmax for STZ ranges from 6.5 · 10−5 nmol s−1 to 8.2 ·
10−5 nmol s−1. On the other hand, maximal uptake velocity is reached if equation
5.7 is maximal, i.e. if Ccell = 0 and, thus, Cenv(t) = Cenv(t=0).
Consequently, for log KOW values of sulfonamides between -1.22 and 1.6 L kg−1 (Ta-
ble 2.1) ku ranges from 2.1 · 10−8 to 1.4 · 10−5 m · s−1 resulting in a maximal velocity
of STZ of 5.3 · 102 to 3.5 · 105 nmol · s−1 with Cenv(t=0) = 5000 nmol (Swedberg
et al., 1979) and a cellular radius of 6.0 · 10−7 m (Moat and Foster, 1995). Compared
to the estimations above the reaction kinetics of sulfonamides in the cell are seven to
ten orders of magnitude slower than the uptake. The steady-state ratio of the total
sulfonamide concentration in the cell (Ccell ) to the applied sulfonamide dose (Cenv)
(potential accumulation factor AF) can thus be used as a measure for the maximum
accumulation of sulfonamides. Only few experimental data exist on sulfonamide ac-
5.3. RESULTS AND DISCUSSION
Figure 5.2: Effect of pKa2 and Pd on potential sulfonamide (a) accumulation factor (AF) and on
(b) anion accumulation factor (AAF) in E. coli cells for the reference scenario (Pn=5.0 · 10−7 m
s−1, pHcell = 7.6; pHenv = 7.0).
cumulation in bacterial cells. For E. coli accumulation ratios of three sulfonamides
(sulfamethoxazole, sulfathiazole, sulfanilamide) from 1.4 to 2.8 have been reported
(Roland et al., 1979; B¨
uttner, 1980). This is in good agreement with
model simulations of the reference scenario (AF: 0.8 - 3.2, see Figure 5.2a).
As the antibiotic effect of sulfonamides is supposed to be solely due to the an-
ionic sulfonamide species (Henry, 1943), accumulation of the anionic species in the
cells is the relevant parameter. The anion accumulation factor (AAF) relates the
effective concentration (intracellular anion concentration) to the applied dose (total
sulfonamide concentration in the surrounding medium). For constant intracellular
pH the anionic fraction decreases with increasing pKa2 values and thus, the AAFdiffers most from the AF in the range of higher pKa2 (Figure 5.2b). The accumu-lation predicted by the model is caused by an "ion-trapping effect" which was first
described by De Duve et al. (1974). Because the external pH (7.0) is lower than the
intracellular pH (7.6), the fraction of neutral species in the surrounding solution is
larger than in the cytoplasm. In order to balance the resulting activity difference,
the neutral species is increasingly transported into the cell, where it is partly depro-
tonated and trapped in form of the anion. Accumulation is largest for pKa-values in
the range from 5 to 7. Compounds with pKa2 values above 9 are not accumulated atall. They exhibit constant AF-values of approximately 0.8 due to the different activ-
ity coefficients inside and outside the cell (Fig. 5.2 a). For these substances, anion
concentration in the cell is negligible and thus the AAF is almost zero (Fig. 5.2 b).
Under such conditions no antibiotic effect of the compound should be observed.
For pKa2 values below 5.0 simulated accumulations depend on the assumed perme-
CHAPTER 5. UPTAKE OF SULFONAMIDES BY MICROORGANISMS
ability ratio for the neutral and ionic species. If ions cannot pass the membrane the
"ion-trapping effect" leads to a constantly large accumulation in this pKa2 range.
Increasing the permeability for the anion results in decreased accumulation due to
the increasing potential for transport of the anions (out of the cell). In the following,
the results of sensitivity analyses for the four most significant parameters determin-
ing the AAF in the model are presented: the permeability ratio of the cell envelope
for the neutral and the ionic species, the extra- and intracellular pH value, and the
dissociation constant pKa2.
Sensitivity of permeability ratio
A critical parameter for the potential accumulation is the ratio of the cell wall perme-
ability for the neutral and the ionic species. If uptake of ions is completely neglected
(Pd = 0) like it was generally assumed for a long time (e.g. Baronofsky et al. (1984)),
accumulation increases with decreasing pKa2 following a sigmoid curve (see Figures5.2a, b). For the reference scenario with a permeability ratio of 103.5 between the
neutral and the ionic species, a maximum potential accumulation of 2.9 is calculated
for sulfonamides with a pKa2 of 6.0. If the permeability for the ions is arbitrarilydecreased by a factor of 10, the maximum accumulation is higher (3.1) and is shifted
towards lower pKa2 values.
For most sulfonamides the permeability of the bacterial cell for ions is not very sen-
sitive to the calculated AAF. This holds true for all sulfonamides with a pKa2 inthe range, where ionic transport velocity across the cell membrane does not signifi-
cantly affect the accumulation behaviour. If we allow a maximum relative deviation
of 5% between simulation results for no ion permeability (AFPd=0) and the referencescenario (AFref , log Pd = log Pn - 3.5), i.e.
AFref − AFPd=0 < 0.05,
the resulting pKa2 limit simply calculates to pHcell - 1.5 independent of the extra-cellular pH. As AAF and AF are linearly related, results are identical no matter
whether AF- or AAF-values are compared3. A decrease of the permeability for the
a · AFref − AFPd=0 =
αa is the anionic sulfonamide fraction in the cell which is constant in the uptake model assuming
constant intracellular pH.
5.3. RESULTS AND DISCUSSION
Figure 5.3: Effect of pKa2 and intracellular pH on anionic accumulation factor in bacterial cells
for pHenv = 7.0. (a) E. coli: regulation of internal pH ; (b)Clostridium thermoaceticum: weak
regulation of internal pH.
ions by a factor of 10 (relative to the neutral form) is propagated linearly through
the model and the limit for pKa2 accordingly decreases to pHcell - 2.5. Consequently,the intracellular pH value and the permeability ratio for the neutral and the ionic
species determine for which sulfonamides anion transport could affect the accumu-
lation behaviour. The intracellular pH in bacterial cells normally does not exceed
a value of 8 (Beales, 2004) resulting in a pKa2-limit of 6.5 for the default perme-ability assumption. For all sulfonamides characterized by a pKa2-value larger than6.5, uptake of the ionic species into bacterial cells is negligible. Although there are
a number of sulfonamides with dissociation constants below this value, sensitivity
to the calculated AAF is rather small and it seems difficult to confirm or reject the
hypothesis that not only neutral sulfonamide molecules but also anions can pass
bacterial cell envelopes on the basis of these data.
Influence of intracellular pH
Most bacteria are able to regulate their intracellular pH, but they differ in the range
of preferred pH values depending on the pH regulation mechanisms of microbial
species (Beales, 2004). For example, the enterobacterium E. coli keeps its intracellu-
lar pH nearly constant between 7.6 and 7.8 regardless of the external pH (Zilberstein
et al., 1984). In contrast, the clostridiaceum Clostridium thermoaceticum, an ubiq-
uitous bacterium primarily occurring in soils and in the intestinal tract, prefers pH
values from 5 to 8 for growth and allows internal pH values to vary from 5.7 to 7.3
(Beales, 2004). Using these two microorganisms as examples the effect of intracel-
lular pH on anionic sulfonamide accumulation is demonstrated. Figure 5.3a shows
CHAPTER 5. UPTAKE OF SULFONAMIDES BY MICROORGANISMS
that even a relatively small shift of 0.2 pH units in an E. coli cell results in diffe-
rent accumulation of sulfonamides with pKa2 values around the optimum (± 1.5 logunits). For conditions with similar extra- and intracellular pH values accumulation
factors are close to 1, i.e. sulfonamides are not accumulated, as has been experi-
mentally observed by Roland et al. (1979). In Figure 5.3b scenarios for minimum
and maximum internal pH in cells of C. thermoaceticum are displayed. It can be
seen that the AAF of sulfonamides with pKa2 = 6 increases by a factor of 10, ifthe intracellular pH increases from 6 to 7. Obviously, the accumulation in bacterial
cells is highly sensitive to the relation between extra- and intracellular pH value. If
the pH value in solution exceeds the intracellular pH value, no accumulation occurs
(AAF < 1), because the neutral fraction is larger inside the cell than outside.
In these cases, the AAF is the smaller the larger the differences between extra- and
intracellular pH are. For compounds with dissociation constants clearly above the
intracellular pH, the AAF approaches zero.
Influence of extracellular pH
Excluding pH optima for growth of extremophiles the physiological pH range of or-
ganisms varies from 5 to about 9 (Madigan et al., 2003). The sensitivity of external
pH on the potential accumulation of a number of sulfonamides with pKa2 valuesranging from 5.7 to 11.8 (Table 2.1) is demonstrated in Figure 5.4. The ratio be-
tween intra- and extracellular concentration increases with decreasing pKa2 valuesand is largest for low external pH values. Sensitivity to external pH variations is
larger for compounds with a small pKa2 value, for which little variations of externalpH lead to a significant change of potential sulfonamide accumulation in the cell.
This implies that the effectiveness of sulfonamides with low pKa2 values should behighly dependent on extracellular pH for bacteria that keep internal pH within a
small pH range. Figure 5.4 shows that the sulfonamide that accumulates the most
changes from sulfisoxasole (SIX) at pHenv = 5.5 to sulfachloropyridazine (SPZ) and
sulfamethoxazole (SMX) at pHenv = 7.0. At pHenv = 8.5 there is no accumulation for
any of the compounds (AAF ≪ 1).This can be attributed to the difference between
extra- and intracellular pH and the respective species distribution in dependence
of pKa2. Thus, the relative antibiotic activity of sulfonamides with different pKa2-values on the same bacteria is not necessarily the same at different extracellular pH
values. The membrane potential E is strongly dependent on the extracellular pH
(Hirota et al., 1981), i.e. with increasing extracellular pH E changes with an average
5.3. RESULTS AND DISCUSSION
Figure 5.4: Calculated anionic accumulation factor of nine sulfonamides with different pKa2 in
bacterial cells for an intracellular pH of 7.6 and three different extracellular pH values.
slope of -22 mV per pH unit (Felle et al., 1980). Over the environmental relevant pH
range, a membrane potential of approximately -70 mV at pH 5 up to -150 mV at pH
9 can be expected. Nevertheless, since E affects only the ion uptake (equation 5.3),
the steady state accumulation is relatively insensitive to variations in membrane po-
tential. Therefore, pH effects on sulfonamide accumulation cannot be attributed to
changes in membrane potential but directly to the substance dissociation behaviour.
Comparison of model results with effect data
Assuming that the accumulation for all sulfonamides is not significantly affected
by cell metabolism and that all sulfonamides are comparably effective as enzyme
inhibitor, the antibiotic effect should be the stronger the higher the accumulation
in the cell is. In other words, minimum inhibitory concentrations (MIC) should be
inversely proportional to the accumulation of the effective molecular species. As
mentioned above, it is commonly supposed that the antibiotic effect of sulfonamides
is solely induced by the anionic sulfonamide species (Henry, 1943). Therefore, MIC
values (in units of µg mL−1) against the gram-negative bacterium Actinobacillus
CHAPTER 5. UPTAKE OF SULFONAMIDES BY MICROORGANISMS
Figure 5.5: Comparison of minimum inhibitory concentration (MIC) of 10 different sulfonamides
(5.0 < pKa2 < 10.1) from Mengelers et al. (1997) measured at constant extracellular pH of 7.0
with the simulated anion accumulation factor (AAF).
pleuropneumoniae determined for 10 sulfonamides by Mengelers et al. (1997) are
compared to the AAF representing the anionic sulfonamide accumulation in the
cells. Under the assumption that all sulfonamide anions exhibit similar inhibitory
effects on the enzyme DHPS the reciprocal of the MIC should be linearly correlated
to the AAF. In Figure 5.5 calculated AAF-values for three different permeability
ratios (anion vs. neutral form) are displayed against the reciprocal MIC values. If
the hypothesized linear relationship between anionic accumulation of sulfonamides
in the cell and the observed growth effect was true, all data points should be close to
the line displayed in the diagram. Deviations of data points from this hypothesized
linear relationship are largest for the scenario with the largest Pd-value (ratio: 103.5),
whereas there is almost no difference between the two other scenarios. As only three
of the ten compounds react sensitive to a change in the permeability assumption of
the cell wall against the anion in the model, it is not possible to ultimately decide
whether a different inhibitory effect or the different permeability ratio produce the
deviation from the linear relationship.
5.3. RESULTS AND DISCUSSION
pH dependent growth inhibition of soil bacterial iso-
Tappe et al. (2008) conducted growth inhibition tests of two sensitive soil bacterial
isolates, Pseudomonas aeruginosa and Pantoea agglomerans against eight sulfon-
amides. Dissociation constants pKa2 of the compounds ranged from 5.7 (SPZ) to11.7 (SGD) (Table 2.1) and additionally, the extracellular pH value was varied be-
tween 5 and 8. Since growth rates of both bacteria are pH dependent, for each of
the four investigated pH values (5, 6, 7, 8) a specific growth rate was determined
in the control sample (without sulfonamide) and set to 100%. The sulfonamide con-
centrations resulting in a 50% reduction of the unaffected growth rates from these
controls were referred to as effective concentration (EC50).
Again, under the assumption that inhibition is proportional to the intracellular an-
ion concentration of the respective sulfonamide, normalized reciprocal EC50 valuesare compared to normalized anion concentrations in the cell simulated for different
scenarios. Simulated intracellular anion concentrations are normalized to the maxi-
mum to enable a direct correlation between anionic sulfonamide accumulation in
the cell and measured growth inhibition. The use of reciprocal effect concentrations
allows for a direct comparison of measured data and observed effects in the graphical
representation, i.e. the higher the column the higher is the inhibitory effect of the
sulfonamide on bacterial growth. In analogy to the reference scenario of the uptake
model, it is assumed that most bacteria are able to maintain an intracellular pH
near neutral growing over a wide pH range (pH homeostasis) (Beales, 2004). This
reference scenario is applied to experimental pH conditions and investigated sulfon-
Reference scenario with pH homeostasis. Data from Mengelers et al. (1997)
and investigations on model parameters and model sensitivity showed that sulfon-
amides with lowest pKa2 accumulate the most in cellular cytoplasm. Model simula-tions (Fig. 5.6) demonstrate that highest intracellular anion concentrations can be
expected at low extracellular pH values (pH 5). This is consistent with experimental
results for P. aeruginosa which showed strongest growth inhibition for sulfonamides
with small pKa2 and at the lowest pH value (pH 5).
Same experiments with P. agglomerans resulted in a different picture than for P.
aeruginosa. The three sulfonamides with the lowest pKa2 (SPZ, SMX, and SDZ)effectively inhibited growth of P. agglomerans at all pH values tested with a clear
CHAPTER 5. UPTAKE OF SULFONAMIDES BY MICROORGANISMS
Figure 5.6: Simulated intracellular anion concentrations of sulfonamides normalized to the maxi-
mum value for better comparison with effect data. The model is parameterized according to the
reference scenario with constant intracellular pH (7.5) and different extracellular pH values.
optimum at pH 7. The next 4 sulfonamides (SDT, STZ, SDM, SPY) ranked for
their increasing pKa2 values, showed an optimum at pH 8. The sulfonamide withthe highest pKa2 (SGD, pKa2=11.69) showed no inhibition at all even at the high-est concentration applied (20 mg L−1). Experimental effect data are illustrated in
Figure 5.7. Spearman's rank correlation coefficient r2 between measured effect and
simulated concentration data is independent of the frequency distribution of the
investigated variables (measured vs. simulated data) and does not require the rela-
tionship between these variables to be linear.
Thus, a bivariate analysis on correlation was performed indicating that measured
effect data and simulated anion concentrations do not correlate significantly (n=32,
5.3. RESULTS AND DISCUSSION
Figure 5.7: Growth inhibitory activities of sulfonamides (sorted with rising pKa2) as normalized
reciprocal values at different pH on Pantoea agglomerans (Tappe et al., 2008).
r2(Spearman)=0.43, p level4 = 0.02). This is probably due to the assumption of a
constant intracellular pH of 7.5 independent of the respective extracellular pH.
Scenario with varying intracellular pH. The strongest inhibition of P. agglom-
erans was obtained at the highest pH values of 7 and 8. According to the model
assumptions this should be associated with the highest internal anion concentration
which does not comply with the assumption of a constant intracellular pH. In order
to explain the discrepancy between data on growth inhibition of P. agglomerans and
model results a consistent shift of simulated internal anion concentrations to higher
pH values is necessary and requires variable intracellular pH values in the model.
Under environmental conditions, this may be explained by a restricted ability of P.
4 Significance of the correlation is determined with SPSS 14.0.1, SPSS Inc. Chicago, Illinois,
CHAPTER 5. UPTAKE OF SULFONAMIDES BY MICROORGANISMS
Figure 5.8: Simulated intracellular anion concentrations of sulfonamides for different extracellular
pH values and variable intracellular pH values (pHi). The respective pHi values were inversely
calculated in order to obtain the best fit to experimental effect data of P. agglomerans given in
Figure 5.7. For extracellular pH values of 5, 6, 7, and 8 the corresponding pHi values are 5.4, 6.0,
7.2 and 7.7.
agglomerans to maintain quasi-constant pH values against a strong gradient from
inside to outside (homeostasis (Kobayashi et al., 2000)). In order to investigate if
such a variable intracellular pH could explain the effect data, pHi values were deter-
mined which fit the model results best. Applying a least-square method (LSM) the
model was fitted to the data for all sulfonamides at the four different extracellular
pH values and intracellular pH values were inversely calculated. For extracellular
pH values of 5, 6, 7, and 8 the corresponding pHi values are 5.4, 6.0, 7.2 and 7.7.
The resulting intracellular anion concentrations predicted by the model are shown
in Figure 5.8.
In contrast to the correlation analysis between simulation results assuming pH home-
5.3. RESULTS AND DISCUSSION
ostasis and measured effect, data of P. agglomerans show a highly significant corre-
lation (n=32, r2(Spearman)=0.92, p level < 0.001) when variable intracellular pH
values are assumed. The calculated pHi values imply a very weak pH regulation in
P. agglomerans. Thus, it is likely that a the impact on the microbial population in
soil through sulfonamide action strongly depends on the way bacteria regulate their
pH in cytoplasm. Measurements of pHi and intracellular substance concentration in
soil bacteria could provide further insight into underlying mechanisms determining
pH- and pKa-dependency of effects.
Integration of enzymatic inhibition into the uptake
So far, a model to estimate intracellular concentrations of the sulfonamide anion in
dependence of the pKa2 and the pH value in the cell and the surrounding mediumwas introduced. Observed effect data (MIC, EC50) on growth inhibition can be qual-itatively explained by the model. Nevertheless, the uptake model does not explicitly
simulate the growth inhibitory effect of sulfonamides.
The next consequential step to an integration of chemical fate and antibiotic effect
focuses on a combination of the uptake model with a kinetic model describing en-
zymatic processes during the folic acid cycle and its inhibition by sulfonamides. A
sensitivity analysis of the enzymatic parameters Michaelis constant (Km) and maxi-
mum velocity (Vmax) allows for an evaluation of the impact of the additional process
on model predictions.
The uptake model calculates the accumulation of the effective anionic sulfonamide
fraction in a bacterial cell (ion trapping) without considering the enzymatic reaction
expressed as the anion accumulation factor (AAF) which depends on the substance
specific dissociation constant pKa2 of the sulfonamide and the extra- and intracel-lular pH value (subsection 5.3.1). The steady state of the integrated uptake and
enzyme reaction model (AAFe) additionally depends on the choice of enzymatic pa-
rameter values (Km, Vmax) and on the applied sulfonamide concentration (equation
5.22). Figure 5.9 compares the anion accumulation factor of different sulfonamides
at pH 7.0 without (AAF) and with (AAFe) consideration of the subsequent enzy-
matic reaction in dependence of the respective pKa2 value for E. coli (pHcell = 7.6).
Simulations were performed with mean values of the parameter range (reference
scenario) and for the maximum and minimum parameter values, respectively (best
and worst case scenarios). Figure 5.9 shows that at high sulfonamide concentra-
CHAPTER 5. UPTAKE OF SULFONAMIDES BY MICROORGANISMS
Figure 5.9: Anion accumulation factor at pHenv = 7.0 of different sulfonamides at two concen-
trations without (AAF) and with (AAFe) consideration of the subsequent enzymatic reaction in
dependence of the respective pKa2 value. AAF is independent of the applied sulfonamide concen-
tration. pHcell was selected for E. coli (7.6). Error bars indicate maximum variability in the selected
parameter range.
tion the AAFe is smaller than AAF for sulfonamides with pKa2 values below 6.0,with only small variability caused by the enzymatic parameters (< 5%). At low
concentrations, however, the simulated AAFe values are much lower than AAF and
also react very sensitive to changes in Vmax,S and Km,S values. This is because the
applied sulfonamide concentration is so low that the respective anionic fraction in
the cell is directly transformed into the DHP analogon and cannot accumulate to
a large extent. At high sulfonamide concentrations, however, intracellular anion ac-
cumulation dominates in the integrated uptake and enzymatic reaction model and
results in an AAFe close to AAF. Nevertheless, the anion accumulation factor alone
does not reflect the effective concentration. Thus, according to equation 5.21 the
model also delivers the amount of produced DHP. In order to determine an effective
sulfonamide concentration, the amount of DHP, produced when no sulfonamide is
5.3. RESULTS AND DISCUSSION
applied, is taken as reference value. Then, for each sulfonamide the simulated con-
centration is determined at which the inhibition results in a 50% reduction of the
DHP-formation in the cell (DHPinh50) as a reference value. Simulations are basedon the assumption that all sulfonamides affect the enzymatic reaction to the same
extent, i.e. enzymatic parameters for all sulfonamides are equal. Consequently, this
means that the intracellular anion concentration that reduces the DHP-formation by
50% is all the same. Then, the DHPinh50 is the concentration that has to be appliedof the specific sulfonamide to achieve this anion concentration in the cells. Figure
5.10 illustrates the correlation between the pKa2 value and the simulated effectivesulfonamide concentration (DHPinh50). Lowest effect concentrations inhibiting theDHP production by 50% are predicted for sulfonamides with pKa2 values between6 and 7.5 which includes SDZ (pKa2 = 6.48). Smaller pKa2 values induce a strongdepletion in the cell and higher values additionally result in a small intracellular
anionic fraction.
Figure 5.10: Correlation between the pKa2 value and the simulated sulfonamide concentration
(DHPinh50) at which the inhibition results in a 50% reduction of the DHP-formation in the cell.
Colours for sulfonamide representation are chosen according to Fig. 5.9.
CHAPTER 5. UPTAKE OF SULFONAMIDES BY MICROORGANISMS
Potential accumulation of sulfonamides in bacterial cells is sensitive to the pH val-
ues in- and outside the cells if cell metabolic reactions do not affect the steady-state
concentration in the cell. The ratio of intra- to extracellular pH and the absolute
pH values determine the total sulfonamide accumulation in dependence of pKa2.
Intracellular pH directly affects the concentration of the effective anionic species
and thus, the antibiotic inhibition of DHPS. The model allows for differentiating
between the uptake of the neutral and ionic species and intracellular shift to anionic
species, which is essential to produce the antibacterial effects of sulfonamides. Rank-
ing of observed effect data agrees with simulated anion accumulation in the cell, but
further experiments are necessary to confirm the proposed relationship between an-
tibiotic inhibition and intracellular accumulation. The uptake model can be extented
by the enzymatic reaction which reduces, in dependence of the amount of applied
sulfonamide, the anionic accumulation in the cell. This integrated model allows to
estimate (effective) sulfonamide concentrations resulting in a 50% reduction of the
Experimental and modelling investigations indicate that bacteria with a strong con-
trol of intracellular pH (pH homeostasis) would be strongly inhibited at low soil pH,
especially by sulfonamides with low pKa values. However, sorption of the neutral
species of sulfonamides seems to be significantly stronger than that of the anionic
species (Boxall et al., 2002). As a competitive process, sorption to soil may then
increase with decreasing pH resulting in a decreased availability for the organisms.
Bacteria with a poor regulation, as postulated for P. agglomerans, would be weakly
inhibited at low soil pH and exhibit strongest inhibition at pH values of 7 to 8, which
is the prevailing pH range in pig manure after storage and alkalinic fermentation.
Briefly summarized, it is likely that a possible impact on the microbial population
in soil through the action of sulfonamides strongly depends on the way bacteria can
regulate their pHi. Measurements of pHi and intracellular substance concentrations
in soil bacteria could provide further insight into underlying mechanisms determin-
ing pH- and pKa-dependency of effects.
In general, bioaccumulation behaviour of organic substances in food webs is mostly
described by model approaches mainly based on the octanol-water partitioning co-
efficient (log KOW ) (Watanabe et al., 2005; Armitage and Gobas, 2007; Norstrom
et al., 2007). Gobas et al. (2006) already pointed out that limits like log KOW >
5 and molecular weight > 500 g · mol−1 are not appropriate indicators to exclude
membrane permeation and thus a significant bioaccumulation. The authors claim
that four of ten chemicals in their BAF-BCF database fulfilling the above men-
tioned criteria are characterized by a BAF or BCF larger than 5000, also including
In this thesis it was shown that for dissociating compounds pH-dependent speciation
can be the decisive process determining the accumulation in bacterial cells. The log
KOW approach alone may lead to an underestimation of the accumulation of sub-
stances that are affected by the described ion trapping effect. This phenomenon may
also play a role for bioaccumulation of ionic substances in other biota. For example,
Lo and Hayton (1981) described a pH effect on the accumulation of sulfonamides in
fish which can be attributed to ion trapping if the absorption rate for the neutral
molecule is significantly larger (at least about two orders of magnitude) than the
absorption rate of the ion. The mechanistic model described in this chapter can thus
also contribute to an improvement of existing bioaccumulation models.
General Discussion and Final
This thesis is based on the investigations and results of the project FOR566 funded
by the German Research Foundation (DFG) with the project title "Veterinary
Medicines in Soil - Basic Research for Risk Analysis". What can finally be con-
cluded as far as risk is concerned? Risk is the probability that a possible adverse
effect occurs. In environmental sciences the risk which may arise from a specific
compound includes the adverse effect and the possibility of environmental exposure
of this compound. Predicted environmental concentrations (PEC) resulting from
exposure modelling are compared with predicted no effect concentrations (PNEC)
which are based on toxicity tests for a specific biological endpoint. If the PEC of a
chemical substance exceeds its PNEC this substance is supposed to constitute an
environmental risk.
Kim et al. (2007) investigated the aquatic toxicity of six sulfonamides and deter-
mined risk quotients indicating "potential environmental concern" (e.g. PEC/PNEC
= 6.3 for sulfamethoxazole). In contrast to an aquatic environment, however, soil
is definitely a more complex compartment comprising a gaseous phase, an aquatic
phase and the solid matrix which itself varies in composition over a wide range of
properties. Additionally, toxicity on the microbial community cannot be tested for
a specific endpoint. Instead sum parameters like soil respiration are often consulted
for an assessment of antibiotic induced effects on soil microorganisms and soil func-
In order to pose a risk to men and environment the substance has to be biologically
active and has to be available for uptake by organisms in soil. Sulfadiazine is, of
CHAPTER 6. FINAL CONCLUSIONS
course, an active substance since it is an antibiotic compound designed to inhibit
bacterial cell growth. This is most likely also the case for its metabolite 4-hydroxy-
sulfadiazine since the active substance group NH2 is not changed by hydroxylation.
But what can we say about availability? Based on estimations of apparent sorption
coefficients (Chapter 3) and on the mechanistic fate model (Chapter 4) the time-
dependent development of the dissolved concentration of SDZ in soils Kaldenkirchen
and Merzenhausen after application of fresh and aged manure can be estimated. Pore
water concentrations of sulfadiazine at the beginning of the central fate experiments
are calculated using equation 3.8 to be 4.5 mg L−1 and 4.7 mg L−1 in the two soils
Kaldenkirchen and Merzenhausen, respectively.
Tappe et al. (2008) experimentally determined effective SDZ concentration (EC50)for Pantoea agglomerans under different pH values in solution. Values of 2.2 mg
L−1 and 0.5 mg L−1 at pH 6 and 7 are reported, respectively. These pH values are
close to the pH conditions of the two soils (K: pH = 5.7, M: pH = 6.8). Hence,
the EC50 values can be used to compare pore water concentrations in the soil witheffect concentrations. The chemical fate model predicts pore water concentrations
to remain above the respective EC50 values for the first 9 days (soil K) and 16 days(soil M) after manure application. This points out that under realistic conditions
effective concentrations can be maintained for relatively long time periods. In or-
der to complete risk assessment for SDZ an analogous estimation for the effective
metabolite, i.e. the hydroxylated sulfadiazine (OH-SDZ), is necessary and possible
when inhibitory concentrations are known.
The results of this thesis confirm that bound residues, which are irreversibly incor-
porated into the soil matrix, e.g. by covalent binding to humic compounds, have to
be distinguished from a kinetically controlled translocation into a residual fraction.
The formation of bound residues in soils reduces the available fraction of the com-
pounds and it is unlikely that the bound molecules - although they persist in soil -
can be re-released in an active form. However, the residual fraction may have long
term effects on soil functions or organisms, because SDZ and OH-SDZ could still
be found more than 200 days after application to soil. Depending on the microor-
ganism species this fraction can still be effective and influence soil functions (Focks,
2008; Kotzerke et al., 2008). Model results indicate that the translocation of SDZ
and its metabolites into the residual fraction is kinetically controlled and may thus
be a diffusion-type physical entrapment process. This is a starting point for further
experimental investigations on sorption of SDZ, OH-SDZ and Ac-SDZ which seems
to be stronger in the residual fraction than in the available one. The model predicts
a long-term remobilization of antibiotic activity from the residual fraction.
Under environmental conditions the soil structure may be changed mechanically by
human impacts. Such "disturbing" processes may again induce a mobilization of
the compound. Several researchers describe a remobilization of sequestered or even
bound xenobiotics by incorporation of microorganisms (Barriuso et al., 2004), bio-
turbation by earthworms (Gevao et al., 2001), or plant growth (Olson et al., 2007).
In addition, plant growth inducing a higher microbial activity in the rhizosphere
and field conditions (temperature, moisture conditions) may influence the chemical
fate of the antibiotic compounds in general and sequestration dynamics in partic-
ular. Consequently, compared to laboratory experiments, the antibiotic activity in
soil may even be enhanced under field conditions. Further analyses of sequestra-
tion mechanisms and the formation of bound residues may allow for an improved
mechanistic understanding of the chemical fate processes. The presented modelling
approach describing the fate of SDZ and its main metabolites can be easily extended
for additional influence factors. Expanding the model by a mathematical description
for antibiotic uptake by plants may supplement the chemical fate by an additional
relevant process which leads to a contamination of the food chain. Finally, integra-
tion of the chemical fate model for homogenized soils into a transport model allows
for an application to the field scale.
H. Akaike. An information criterion. Mathematical Science, 14:5–9, 1976.
M. Alexander, C.-J. Estler, and F. Legler. Antibiotika und Chemotherapeutika. In
H. P. T. Ammon and C. Werning, editors, Medizinisch-pharmakologisches Kom-
pendium, volume 8. Wissenschaftliche Verlagsgesellschaft Stuttgart, 1990.
W. Amelung and M. Kaupenjohann. FOR 566: Veterinary Medicines in Soils -
Basic Research for Risk Analysis. Report to project A3 (Bonn): Sequestration of
veterinary medicines in soils. Technical report, INRES Division of Soil Science,
University of Bonn, 2007.
C. A. J. Appelo and D. Postma.
Geochemistry, Groundwater and Pollution.
Balkema, Rotterdam, NL, 4th edition, 1999.
J. M. Armitage and F. A. P. C. Gobas. A terrestrial food-chain bioaccumulation
model for POPs. Environ Sci Technol, 41(11):4019–4025, 2007.
P. W. Atkins. Physical Chemistry. Oxford University Press, Oxford, 4th edition,
J. B. Baronofsky, W. J. A. Schreurs, and E. R. Kashket. Uncoupling by acetic acid
limits growth of and acetogenesis by Clostridium thermoaceticum. Appl Environ
Microbiol, 48(6):1134–1139, 1984.
E. Barriuso, W. C. Koskinen, and M. J. Sadowsky. Solvent extraction characteri-
zation of bioavailability of atrazine residues in soils. J Agric Food Chem, 52(21):
6552–6556, 2004.
N. Beales. Adaptation of Microorganisms to Cold Temperatures, Weak Acid Preser-
vatives, Low pH, and Osmotic Stress: A Review. Compr Rev Food Sci F, 3:1–20,
H. M. Bialk and J. A. Pedersen. NMR investigation of enzymatic coupling of sulfon-
amide antimicrobials with humic substances. Environ Sci Technol, 42(1):106–112,
H. M. Bialk, A. J. Simpson, and J. A. Pedersen. Cross-coupling of sulfonamide
antimicrobial agents with model humic constituents. Environ Sci Technol, 39(12):
4463–6673, 2005.
P. A. Blackwell, P. Kay, and A. B. A. Boxall. The dissipation and transport of
veterinary antibiotics in a sandy loam soil. Chemosphere, 67:292–299, 2007.
R. B¨ohm. Auswirkungen von R¨
uckst¨anden von Antiinfektiva in tierischen Auss-
cheidungen auf die G¨
ullebehandlung und den Boden. Deut Tier¨arztl Woch, 103:
237–284, 1996.
A. B. A. Boxall, P. Blackwell, R. Cavallo, P. Kay, and J. Tolls. The sorption and
transport of a sulphonamide antibiotic in soil systems. Toxicol Lett, 131:19–28,
A. B. A. Boxall, D.W. Kolpin, B. Halling-Sørensen, and J. Tolls. Are veterinary
medicines causing environmental risks? Environ Sci Technol, 8:286–294, 2003.
K. Breivik and F. Wania. Expanding the applicability of multimedia fate models to
polar organic chemicals. Environ Sci Technol, 37:4934–4943, 2003.
G. E. Briggs, A. B. Hope, and R. N. Robertson. Electrolytes and Plant Cells.
In W. O. James, editor, Botanical Monographs, volume 1. Blackwell Scientific
Publications Oxford, 1961.
G. M. Brown. The biosynthesis of folic acid - II. Inhibition by sulfonamides. J Biol
Chem, 237(2):536–540, 1962.
M. Burkhardt and C. Stamm. Depth distribution of sulfonamide antibiotics in pore
water of undisturbed loamy grassland soil. J Environ Qual, 36:588–596, 2007.
uttner and H. B¨
uttner. Binding of 35S-sulfadiazine to cells and cell fractions of
Escherichia coli. Chemotherapy, 17:309–319, 1972.
uttner and H. B¨
uttner. pH dependency in uptake of sulfonamides by bacteria.
Chemotherapy, 26:153–163, 1980.
M.-P. Charnay, C. Mougin, A. Farrugia, and E. Barriuso. Incorporation of pesticides
by soil micro-organisms as a way of bound residues formation. Environ Chem Lett,
2:27–30, 2004.
T. Christian, R. J. Schneider, H. A. F¨arber, D. Skutlarek, M. T. Meyer, and H. E.
Goldbach. Determination of antibiotic residues in manure, soil, and surface waters.
Acta hydrochim hydrobiol, 31(1):36–44, 2003.
A. Craven and S. Hoy. Pesticide persistence and bound residues in soil - regulatory
significance. Environ Pollut, 133(1):5–9, 2005.
C. De Duve, T. De Barsy, B. Poole, A. Trouet, P. Tulkens, and F. Van Hoof. Com-
mentary. Lysosomotropic agents. Biochem Pharmacol, 23:2495–2531, 1974.
A. Delle Site. Factors affecting sorption of organic compounds in natural sor-
bent/water systems and sorption coefficients for selected pollutants. A review.
J Phys Chem Ref Data, 30(1):187–439, 2001.
U. D¨orfler, R. Haala, M. Matthies, and I. Scheunert. Mineralization kinetics of
chemicals in soils in relation to environmental conditions. Ecotox Environ Safe,
P. Drillia, K. Stamatelatou, and G. Lyberatos. Fate and mobility of pharmaceuticals
in solid matrices. Chemosphere, 60:1034–1044, 2005.
B. I. Escher, R. Hunziker, R. Schwarzenbach, and J. C. Westall. Kinetic model to
describe the intrinsic uncoupling activity of substituted phenols in energy trans-
ducing membranes. Environ Sci Technol, 33(4):560–570, 1999.
H. Felle, J. S. Porter, C. L. Slayman, and H. R. Kaback. Quantitative measurement
of membrane potential in Escherichia coli. Biochemistry, 19(15):3585–3590, 1980.
R. A. Figueroa, A. Leonard, and A. A. Mackay. Modeling tetracycline antibiotic
sorption to clays. Environ Sci Technol, 38(2):476–483, 2004.
A. Focks. PhD thesis, University of Osnabr¨
uck, 2008. in preparation.
J. Gao and J. A. Pedersen. Adsorption of sulfonamide antimicrobial agents to clay
minerals. Environ Sci Technol, 39(24):9509–9516, 2005.
C. T. Garten Jr. and J. R. Trabalka. Evaluation of models for predicting terrestrial
food chain behavior of xenobiotics. Environ Sci Technol, 17(10):590–595, 1983.
S. Gartiser, E. Urich, R. Alexy, and K. K¨
ummerer. Ultimate biodegradation and
elimination of antibiotics in inherent tests. Chemosphere, 67:604–613, 2007a.
S. Gartiser, E. Urich, R. Alexy, and K. K¨
Anaerobic inhibition and
biodegradation of antibiotics in ISO test schemes. Chemosphere, 66:1839–1848,
B. Gevao, C. Mordaunt, K. T. Semple, T. G. Piearce, and K. C. Jones. Bioavailability
of nonextractable (bound) pesticide residues to earthworms. Environ Sci Technol,
F. A. P. C. Gobas, M. M. Moore, J. L. M. Hermens, and J. A. Arnot. Bioaccumu-
lation reality check. setac globe, 5:40–41, 2006.
A. G¨obel, A. Thomsen, C.S. McArdell, A. Joss, and W. Giger. Occurence and sorp-
tion behavior of sulfonamides, macrolides, and trimethoprim in activated sludge
treatment. Environ Sci Technol, 39:3981–3989, 2005.
A. G¨obel, C. S. McArdell, A. Joss, H. Siegrist, and W. Giger. Fate of sulfonamides,
macrolides, and trimethroprim in different wastewater treatment technologies. Sci
Total Environ, 372:361–371, 2007.
K.-U. Goss and R.P. Schwarzenbach. Linear free energy relationships used to evalu-
ate equilibrium partitioning of organic compounds. Environ Sci Technol, 35:1–9,
M. Grote, A. Vockel, D. Schwarze, A. Mehlich, and M. Freitag. Fate of antibiotics
in food chain and environment originating from pigfattening (Part 1). Fresen
Environ Bull, 13(11 b):1216–1224, 2004.
A. M. Hammerum, D. Sandvang, S. R. Andersen, A. M. Seyfarth, L. J. Porsbo,
N. Frimodt-Møller, and O. E. Heuer.
Detection of sul1, sul2 and sul3 in
sulphonamide resistant Escherichia coli isolates obtained from healthy humans,
porks and pigs in Denmark. Int J Food Microbiol, 106(2):235–237, 2006.
G. Hamscher, H. T. Pawelzick, H. H¨oper, and H. Nau. Different behavior of tetra-
cyclines and sulfonamides in sandy soils after repeated fertilization with liquid
manure. Environ Toxicol Chem, 24(4):861–868, 2005.
R. E. W. Hancock. The bacterial outer membrane as a drug barrier. Trends Micro-
biol, 5(1):37–42, 1997.
C. Hansch, A. Leo, and D. Hoekman, editors. Exploring QSAR. Hydrophobic, Elec-
tronic, and Steric Constants, volume 2. American Chemical Society, Washington
R. J. Henry. The mode of action of sulfonamides. Bacteriol Rev, 7(4):175–262, 1943.
H. Heuer and K. Smalla. Manure and sulfadiazine synergistically increased bacterial
antibiotic resistance in soil over at least two month. Environ Microbiol, 9(3):
657–666, 2007.
H. Heuer, A. Focks, M. Lamsh¨oft, K. Smalla, M. Matthies, and M. Spiteller. Fate
of sulfadiazine administered to pigs and its quantitative effect on the dynamics
of bacterial resistance genes in manure and manured soil. Soil Biol Biochem,
(accepted), 2008.
N. Hirota, S. Matsuura, N. Mochizuki, N. Mutoh, and Y. Imae. Use of lipophilic
cation-permeable mutants for measurement of transmembrane electrical potential
in metabolizing cells of Escherichia coli. J Bacteriol, 148(2):399–405, 1981.
J. V. Holm, P. L. B R¨
ugge, and T. H. Christensen. Occurence and distribution of
pharmaceutical organic compounds in the groundwater downgradient of a landfill
(Grindsted, Denmark). Environ Sci Technol, 29(5):1415–1420, 1995.
utzhoft, W. H. J. Vaes, A. P. Freidig, B. Halling-Sørensen, and J. L. M.
Hermens. Influence of pH and other modifying factors on the distribution behavior
of 4-quinolones to solid phases and humic acids studied by "negligible depletion"
SPME-HPLC. Environ Sci Technol, 34:4989–4994, 2000.
S. H¨oltge and R. Kreuzig. Laboratory testing of sulfmethoxazole and its metabolite
acetyl-sulfmethoxazole in soil. Clean, 35(1):104–110, 2007.
B. Infante, M. Grape, M. Larsson, C. Kristiansson, L. Pallecchi, G. M. Rossolini, and
G. Kronvall. Acquired sulphonamide resistance genes in faecal Escherichia coli
from healthy children in Bolivia and Peru. Int J Antimicrob Ag, 25(4):308–312,
F. Ingerslev and B. Halling-Sørensen. Biodegradability properties of sulfonamides
in activated sludge. Environ Toxicol Chem, 19(10):2467–2473, 2000.
J. Josephson. The microbial "resistome". Environ Sci Technol, 40(21):6531–6534,
M. Kahle and C. Stamm. Sorption of the veterinary antimicrobial sulfathiazole to
organic materials of different origin. Environ Sci Technol, 41(1):132–138, 2007.
P. Kay, P.A. Blackwell, and A.B.A. Boxall. Transport of veterinary antibiotics in
overland flow following the application of slurry to arable land. Chemosphere, 59
P. Kay, P.A. Blackwell, and A.B.A. Boxall. Column studies to investigate the fate
of veterinary antibiotics in clay soils following slurry application to agricultural
land. Chemosphere, 60(4):497–507, 2005b.
Y. Kim, K. Choi, J. Jung, S. Park, P.-G. Kim, and J. Park. Aquatic toxicity of
acetaminophen, carbamazepine, cimetidine, diltiazem and six major sulfonamides,
and their potential ecological risks in Korea. Environ Int, 33(3):370–375, 2007.
T. Kitahara, H. Yoneyama, and T. Nakae. Antibiotic diffusion pathways in the outer
membrane of Pseudomonas aeruginosa. Biochem Bioph Res Co, 238(2):457–461,
H. Kobayashi, H Saito, and T. Kakegawa. Bacterial strategies to inhabit acidic
environments. J Gen Appl Microbiol, 46:235–243, 2000.
T. K¨ohler, M. Kok, M. Michea-Hamzehpour, P. Plesiat, N. Gotoh, T. Nishino, L. K.
Curty, and J.-C. Pechere. Multidrug efflux in intrinsic resistance to trimethoprim
and sulfamethoxazole in Pseudomonas aeruginosa. Antimicrob Agents Ch, 40(10):
2280–2290, 1996.
F. Korte, editor. Lehrbuch der ¨
Okologischen Chemie - Grundlagen und Konzepte
okologische Beurteilung von Chemikalien. Georg Thieme Verlag Stuttgart,
New York, 1992.
A. Kotzerke, S. Sharma, K. Schauss, H. Heuer, S. Thiele-Bruhn, K. Smalla, B.-M.
Wilke, and M. Schloter. Alterations in soil microbial activity and n-transformation
processes due to sulfadiazine loads in pig-manure. Environ Poll, 153(2):315–322,
R. Kreuzig and S. H¨oltge. Investigations on the fate of sulfadiazine in manured soil:
Laboratory experiments and test plot studies. Environ Toxicol Chem, 24:771–776,
R. Kreuzig, C. Kullmer, B. Matthies, S. H¨oltge, and H. Dieckmann. Fate and be-
haviour of pharmaceutical residues in soils. Fresen Environ Bull, 12(6):550–558,
R. Kreuzig, S. H¨oltge, J. Brunotte, N. Berenzen, J. Wogram, and R. Schulz. Test-
plot studies on runoff of sulfonamides from manured soils after sprinkler irrigation.
Environ Toxicol Chem, 24(4):777–781, 2005.
E. Kruger, B. Zhu, and R. Coats. Relative mobilities of atrazine, five atrazine
degradates, metolachor, and simazine in soils of Iowa. Environ Toxicol Chem, 15:
691–695, 1996.
K. Kumar, S. C. Gupta, S. K. Baidoo, Y. Chander, and C. J. Rosen. Antibiotic
uptake by plants from soil fertilized with animal manure. J Environ Qual, 34:
2082–2085, 2005.
S. T. Kurwadkar, C. D. Adams, M. T. Meyer, and D. W. Kolpin. Effects of sorbate
speciation on sorption of selected sulfonamides in three loamy soils. J Agric Food
M. Lamsh¨oft, P. Sukul, S. Z¨
uhlke, and M. Spiteller. Metabolism of 14C-labelled and
non-labelled sulfadiazine after administration to pigs. Anal Bioanal Chem, 388:
1733–1745, 2007.
G. Lancini and F. Parenti. Antibiotics - An Integrated View. Springer-Verlag New
J.-P. Langhammer.
Untersuchungen zum Verbleib antimikrobiell wirksamer
Arzneistoffe als R¨
ulle und im landwirtschaftlichen Umfeld. PhD
thesis, Rheinische Friedrich-Wilhelms-Universit¨at Bonn, 1989.
W. R. Lieb and W. D. Stein. Current Topics in Membranes and Transport, chapter
The Molecular Basis of Simple Diffusion within Biological Membranes, pages 1–18.
Academic Press, New York, 1971.
J.H. Lin and A.Y.H. Lu. Role of pharmacokinetics and metabolism in drug discovery
and development. Pharmacol Rev, 49 (4):403–449, 1997.
G. Liu and J. Yu. QSAR analysis of soil sorption coefficients for polar organic
chemicals: Substituted anilines and phenols. Water Res, 39:2048–2055, 2005.
L. Liu, J. A. Tindall, M. J. Friedel, and W. Zhang. Biodegradation of organic
chemicals in soil/water microcosms system: Model development. Water Air Soil
Pollut, 178:131–143, 2007.
I-H. Lo and W. L. Hayton. Effects of pH on the accumulation of sulfonamides by
fish. J Pharmacokinet Biop, 9(4):443–459, 1981.
M. T. Madigan, J. M. Martinko, and J. Parker. Brock Biology of Microorganisms.
Pearson Education International New Jersey, 10th edition, 2003.
T. M. Marsteller and B. Fenwick. Actinobacillus pleuropneumoniae disease and
serology. J Swine Health Prod, 7(4):161–165, 1999.
S. F. Mason. The tautomerism of N-heteroaromatic hydroxy-compounds. Part III.
Ionisation constants. Australian National University. Chemistry Department. The
University. Exeter, 1958.
M. Matthies, J. Witt, and J. Klasmeier. Determination of soil degradation half-
lives from simulation testing under aerobic laboratory conditions: A kinetic model
approach. Environ Pollut, 2008. in press.
M. J. B. Mengelers, P. E. Hougee, L. H. M. Janssen, and A. S. J. P. A. M. van Miert.
Structure-activity relationships between antibacterial activities and physicochemi-
cal properties of sulfonamides. J Vet Pharmacol Therap, 20:276–283, 1997.
A. Moat and J. W. Foster. Microbial Physiology. Wiley-Liss, New York, 3rd edition,
C. Moreau and C. Mouvet. Sorption and desorption of atrazine, deethylatrazine,
and hydroxyatrazine by soil and aquifer solids. J Environ Qual, 26:416–424, 1997.
T. H. Nguyen, K.-U. Goss, and W. P. Ball. Polyparameter linear free energy rela-
tionships for estimating the equilibrium partition of organic compounds between
water and the natural organic matter in soils and sediments. Environ Sci Technol,
R. J. Norstrom, T. P. Clark, M. Enright, B. Leung, K. G. Drouillard, and C. R.
MacDonald. ABAM, a model for bioaccumulation of POPs in birds: validation
for adult herring gulls and their eggs in lake Ontario. Environ Sci Technol, 41
J. F. M. Nouws, T. B. Vree, and Y. A. Hekster. In vitro antimicrobial activity of
hydroxy and N4-acetyl sulphonamide metabolites. Vet Quart, 7(1):70–72, 1985.
P. E. Olson, A. Castro, M. Joern, N. M. DuTeau, E. A. H. Pilon Smith, and K. F.
Reardon. Comparison of plant families in a greenhouse phytoremediation study
on an aged polycyclic aromatic hydrocarbon-contaminated soil. J Environ Qual,
A. Pagliara, P.-A. Carrupt, G. Caron, P. Gaillard, and B. Testa. Lipophilicity profiles
of ampholytes. Chem Rev, 97(8):3385–3400, 1997.
J. J. Pignatello and B. Xing. Mechanisms of slow sorption of organic chemicals to
natural particles. Environ Sci Technol, 30(1):1–11, 1996.
J.R. V. Pils and D. A. Laird. Sorption of tetracycline and chlortetracycline on K-
and Ca-saturated soil clays, humic substances, and clay-humic complexes. Environ
Sci Technol, 41(6):1928–1933, 2007.
A. Pruden, R. Pei, H. Storteboom, and K. H. Carlson. Antibiotic resistance genes
as emerging contaminants: Studies in Northern Colorado. Environ Sci Technol,
H. P. Rang, M. M. Dale, and J. M. Ritter. Pharmacology. Churchill Livingstone,
J. A. Raven. Transport of indoleacetic acid in plant cells in relation to pH and
electrical potential gradients, and its significance for polar IAA transport. New
Phytol, 74:163–172, 1975.
G. Rhodes, G. Huys, J. Swings, P. McGann, M. Hiney, P. Smith, and R. W. Pickup.
Distribution of oxytetracycline resistance plasmids between Aeromonads in hos-
pital and aquaculture environments: Implication of Tn1721 in dissemination of
the tetracycline resistance determinant Tet A. Appl Environ Microbiol, 66(9):
3883–3890, 2000.
T. R. Roberts, W. Klein, G. G. Still, P. C. Kearney, N. Drescher, J. Desmoras, H. O.
Esser, N. Aharonson, and J. W. Vonk. Non-extractable pesticide residues in soils
and plants. Pure Appl Chem, 56(7):945–956, 1984.
S. Roland, R. Ferone, R. J. Harvey, V. L. Styles, and R. W. Morrison. The charac-
teristics and significance of sulfonamides as substrates for Escherichia coli dihy-
dropteroate synthase. J Biol Chem, 254(20):10337–10345, 1979.
K. Saffih-Hdadi, L. Bruckler, and E. Barriuso. Modeling of sorption and biodegra-
dation of parathion and its metabolite paraoxon in soil. J Environ Qual, 32:
2207–2215, 2003.
H. Sakurai and T. Ishimitsu. Microionization constants of sulphonamides. Talanta,
27:293–298, 1980.
A. K. Sarmah, M. T. Meyer, and A. B. A. Boxall. A global perspective on the use,
sales, exposure pathways, occurrence, fate and effects of veterinary antibiotics
(VAs) in the environment. Chemosphere, 65(5):725–759, 2006.
P. Schachtschabel, H.-P. Blume, G. Br¨
ummer, K. H. Hartge, and U. Schwertmann.
Lehrbuch der Bodenkunde. Ferdinand Enke Verlag Stuttgart, 14th edition, 1998.
K. Schauss, A. Focks, S. Leininger, A. Kotzerke, Heuer. H., S. Thiele-Bruhn,
S. Sharma, B.-M. Wilke, M. Matthies, K. Smalla, J. C. Munch, W. Amelung,
M. Kaupenjohann, C. Schleper, and M. Schloter. Dynamics and functional rele-
vance of ammonia-oxidizing archaea in agricultural soils. 2008. submitted.
B. Schmidt, J. Ebert, M. Lamsh¨oft, B. Thiede, R. Schumacher-Buffel, R. Ji, F.-X.
Corvini, and A. Sch¨affer. Fate in soil of 14C-sulfadiazine residues contained in the
manure of young pigs treated with a veterinary antibiotic. J Environ Sci Health
Part B, 43(1):8–20, 2008.
M. Schneidereit. Antibiotikaeinsatz in der Veterin¨armedizin – Situation in Deutsch-
land und anderen europ¨aischen Veredelungsregionen. Presentation on the occa-
sion of the 20th annual conference of the Paul-Ehrlich-Society for chemotherapy
in Bonn, Germany, September 2006.
H. Schrempf. Personal communication, February 2007.
R. P. Schwarzenbach, Gschwend P. M., and D. M. Imboden. Environmental Organic
Chemistry. John Wiley & Sons, Inc., 2nd edition, 2003.
C.A. Seybold and W. Mersie. Adsorption and desorption of atrazine, deethylatrazine,
deisopropylatrazine, hydroxyatrazine, and metolachlor in two soils from Virginia.
J Environ Qual, 25:1179–1185, 1996.
B. B. Sithole and R. D. Guy. Models for Tetracycline in Aquatic Environments.
Water, Air and Soil Pollution, 32:315–321, 1987.
M. Spiteller. FOR 566: Veterinary Medicines in Soils - Basic Research for Risk
Analysis. Report to project A1: Metabolism and degradation kinetics of selected
veterinary drugs in manure. Technical report, Institute of Environmental Re-
search, University of Dortmund, 2007.
K. Stoob, H. P. Singer, S. Stettler, N. Hartmann, S. R. M¨
uller, and C. H. Stamm.
Exhaustive extraction of sulfonamide antibiotics from aged agricultural soils using
pressurized liquid extraction. J Chromatogr A, 1128:1–9, 2006.
K. Stoob, H. P. Singer, S. R. Mueller, R. P. Schwarzenbach, and C. H. Stamm. Dis-
sipation and transport of veterinary sulfonamide antibiotics after manure appli-
cation to grassland in a small catchment. Environ Sci Technol, 41(21):7349–7355,
G. Swedberg, S. Castensson, and O. Sk¨old. Characterization of mutationally al-
tered dihydropteroate synthase and its ability to form a sulfonamide-containing
dihydrofolate analog. J Bacteriol, 137(1):129–136, 1979.
T. L. Talarico, I. K. Dev, W. S. Dallas, R. Ferone, and P. H. Ray. Purification and
and 7,8-dihydropteroate synthase from Escherichia coli MC4100. J Bacteriol, 173
W. Tappe, C. Zarfl, S. Kummer, P. Burauel, H. Vereecken, and J. Groeneweg.
Growth-inhibitory effects of sulfonamides at different pH: Dissimilar susceptibil-
ity patterns of a soil bacterium and a test bacterium used for antibiotic assays.
Chemosphere, 72:836–843, 2008.
T. L. Ter Laak, W. A. Gebbing, and J. Tolls. Estimation of soil sorption coefficients
of veterinary pharmaceuticals from soil properties. Environ Toxicol Chem, 25(4):
933–941, 2006.
S. Thiele. Adsorption of the antibiotic pharmaceutical compound sulfspyridine by
a long-term differently fertilized loess chernozem. J Plant Nutr Soil Sci, 163(6):
589–594, 2000.
S. Thiele-Bruhn. Pharmaceutical antibiotic compounds in soils - a review. J Plant
Nutr Soil Sc, 166:145–167, 2003.
S. Thiele-Bruhn. Microbial inhibition by pharmaceutical antibiotics in different
soils-dose-response relations determined with the iron(III) reduction test. Environ
Toxicol Chem, 24(4):869–876, 2005.
S. Thiele-Bruhn and M.-O. Aust. Effects of pig slurry on the sorption of sulfonamide
antibiotics in soil. Arch Environ Con Tox, 47:31–39, 2004.
S. Thiele-Bruhn and I.-C. Beck. Effects of sulfonamide and tetracycline antibiotics
on soil microbial activity and microbial biomass. Chemosphere, 59:457–465, 2005.
S. Thiele-Bruhn, T. Seibicke, H.-R. Schulten, and P. Leinweber. Sorption of sul-
fonamide pharmaceutical antibiotics on whole soils and particle-size fractions. J
Environ Qual, 33:1177–1182, 2004.
J. Tolls. Sorption of veterinary pharmaceuticals in soils: A review. Environ Sci
Technol, 35(17):3397–3406, 2001.
S. Trapp. Plant uptake and transport models for neutral and ionic chemicals. Env-
iron Sci Pollut R, 11(1):33–39, 2004.
S. Trapp and R. W. Horobin. A predictive model for the selective accumulation of
chemicals in tumor cells. Eur Biophys J, 34:959–966, 2005.
S. Trapp and M. Matthies. Chemodynamics and Environmental Modeling. Springer,
B. Tras, M. Elmas, E. Yazar, A. L. Bas, E. Keskin, and Z. Dasci. Concentrations of
sulfadoxine and trimethoprim in plasma, lymph fluids and some tissues 24 h after
intramuscular administration to angora goats. Vet Quart, 20(2):62–64, 1998.
H. G. Vinnicombe and J. P. Derrick. Dihydropteroate synthase from streptococcus
pneumoniae: Characterization of substrate binding order and sulfonamide inhibi-
tion. Biochem Bioph Res Co, 258:752–757, 1999.
T.B. Vree and Y.A. Hekster. Clinical Pharmacokinetics of Sulfonamides and Their
Metabolites. In Antibiotics and Chemotherapy, volume 37. Karger, 1987.
R. W. Walters and R. G. Luthy. Equilibrium adsorption of polycyclic aromatic
hydrocarbons from water onto activated carbon. Environ Sci Technol, 18(6):395–
K. H. Watanabe, H.-I. Lin, H. L. Bart Jr., P. Martinat, J. C. Means, M. L. Kunas,
and D. A. Grimm. Bayesian estimation of kinetic rate constants in a food-web
model of polycyclic aromatic hydrocarbon bioaccumulation. Ecol Model, 181:229–
W. J. Weber Jr., P. M. McGinley, and L. E. Katz. A distributed reactivity model for
sorption by soils and sediments. 1. Conceptual basis and equilibrium assessments.
Environ Sci Technol, 26(10):1955–1962, 1992.
A. Wehrhan, R. Kasteel, J. Simunek, J. Groeneweg, and H. Vereecken. Transport of
sulfadiazine in soil columns - Experiments and modelling approaches. J Contam
Hydrol, 89:107–135, 2007.
P. L. Weiden, W. Epstein, and S. G. Schultz. Cation transport in Escherichia coli.
VII. Potassium requirement for phosphate uptake. J Gen Physiol, 50:1641–1661,
C. Zarfl, J. Klasmeier, and M. Matthies. Transformation reaction kinetics and for-
mation of bound residues of sulfadiazine in manure-amended soils. 2008a. in
C. Zarfl, M. Matthies, and J. Klasmeier. A mechanistical model for the uptake of
sulfonamides by bacteria. Chemosphere, 70:753–760, 2008b.
D. Zilberstein, V. Agmon, S. Schuldiner, and E. Padan. Escherichia coli intracellular
pH, membrane potential, and cell growth. J Bacteriol, 158(1):246–252, 1984.
S. Zimmermann. Sorption of sulfonamides to compost and manure. Master's thesis,
University of Trier, 2006.
Beiträge des Instituts für Umweltsystemforschung
der Universität Osnabrück
Eberhard Umbach: Umweltverträgliches Wirtschaftssystem in den Bereichen Abfall und Emissionen. März 1997.
Stefan Trapp, Bernhard Reiter, Michael Matthies: Überprüfung und Fortentwicklung der Bodenwerte für den Boden-Pflanze-Pfad - Teilprojekt Transferfaktoren Boden-Pflanze. August 1997.
Michael Matthies (Hrsg.): Stoffstromanalyse und Bewertung. September 1997.
Dirk Melcher: Quantifizierung, Klassifizierung und Modellierung der Phytotoxizität organischer Chemikalien. Oktober 1997.
Stefan Schwartz: Organische Schadstoffe in der Nahrungskette - Vorstudie zur Validierung von Expositionsmodellen. November 1997.
Volker Berding: Private Hausbrunnen - Vergleichende Bewertung von Maßnahmen zur Verbesserung der Trinkwasserqualität. Oktober 1997.
Horst Malchow (Hrsg.): Modellbildung und -anwendung in den Wissenschaften I. Januar 1998.
Birgit Radtke: Bifurkationen in einem Modell mariner Planktondynamik. Januar 1998.
Werner Berens: Konzeption eines Umweltinformationssystems für die Universität Osnabrück. Juni 1998.
10. Michael Matthies (Hrsg.): Studienprojekte 1998. September 1998.
11. Michael Matthies (Hrsg.): Globaler Wandel. September 1998.
12. Klaus Brauer (Hrsg.): Institutsbericht. September 1998.
13. Klaus Brauer, Horst Malchow, Michael Matthies, Eberhard Umbach (Hrsg.):
Materialien des Arbeitstreffens Systemwissenschaft in der Lehre, Universität Osnabrück, 29./30.9.1998. Dezember 1998.
14. Horst Malchow (Hrsg.): Modellbildung und -anwendung in den Wissenschaften II.
15. Horst Malchow (Hrsg.): Modellbildung und -anwendung in den Wissenschaften III.
16. Michael Matthies (Hrsg.): Regionale Nachhaltigkeit. September 2000.
17. Markus Klein: Langjähriger Wasserhaushalt von Gras- und Waldbeständen.
Entwicklung, Kalibrierung und Anwendung des Modells LYFE am Groß-Lysimeter St. Arnold. Juni 2000.
18. Markus Brune: Multimediale Umweltmodellierung mit Fuzzy-Mengen. Juli 2000.
19. Michael Matthies (Hrsg.): Fraktale in Hydrologie und Biologie. Oktober 2000.
20. Stefan Fuest (Dissertation): Regionale Grundwassergefährdung durch Nitrat. Dezember
21. Carsten Schulze (Dissertation): Modelling and evaluating the aquatic fate of detergents.
Die Beiträge können gegen einen Selbstkostenpreis (ca. 10 EUR pro Exemplar) beim Institut für Umweltsystemforschung, Universität Osnabrück, 49069 Osnabrück bestellt werden. Alle folgenden Beiträge sind herunterzuladen unter http://www.usf.uos.de/usf/literatur/beitraege/
22. Horst Malchow (Hrsg.): Modellbildung und -anwendung in den Wissenschaften IV.
23. Horst Malchow (Hrsg.): Modellbildung und -anwendung in den Wissenschaften V.
24. Kai Lessmann (Diplomarbeit): Probabilistic Exposure Assessment. Parameter
Uncertainties and their Effects on Model Output. November 2002.
25. Frank M. Hilker (Diplomarbeit): Parametrisierung von Metapopulationsmodellen. März
26. Nadja Rüger (Diplomarbeit): Habitat suitability for Populus euphratica in the Northern
Amudarya delta - a fuzzy approach. Juni 2003.
27. Claudia Pahl-Wostl, Eva Ebenhöh (Hrsg.): Komplexe Adaptive Systeme. Juli 2003.
28. Horst Malchow (Hrsg.): Chaos und Ordnung in Natur und Gesellschaft. Dezember
29. Andreas Focks (Diplomarbeit): Modeling the transfer of antibiotic drug resistance genes
between E. coli strains. Juni 2005.
30. Christiane Zarfl (Diplomarbeit): Modellierung von Arsen in der Mulde. Juni 2005.
31. Sven Lautenbach (Dissertation): Modellintegration zur Entscheidungsuntersttzung für
die Gewässergütebewirtschaftung im Einzugsgebiet der Elbe. November 2005.
32. Frank M. Hilker and Frank H. Westerhoff: Control of chaotic population dynamics:
Ecological and economic considerations. November 2005.
33. Harold Fellermann (Diplomarbeit): Micelles as containers for protocells. Dezember
34. Jens Newig, Oliver Fritsch (Hrsg.): Effektivität von Beteiligungsprozessen. Mai 2006.
35. Ba Kien Tran (Diplomarbeit): Modellierung biologischer Invasionen mit Reaktions-
Diffusionsgleichungen. Juli 2006.
Persönlichkeitsunterschieden auf der Grundlage der PSI-Theorie. Juli 2006.
vierkomponentigen populationsdynamischen Modellen. September 2006.
38. Horst Malchow (Hrsg.): Komplexe Systeme und nichtlineare Dynamik in Natur und
Gesellschaft. Dezember 2006.
39. Jens Newig, Veronika Gaube, Karin Berkhoff, Kai Kaldrack, Britta Kastens, Juliana
Lutz, Bianca Schlußmeier. Heidelinde Adensam, Helmut Haberl, Claudia Pahl-Wostl, Armand Colard, Bettina Aigner, Rudolf Maier, Wolfgang Punz: Partizipative Modellbildung, Akteurs-.und Ökosystemanalyse in Agrarintesivgebieten. Juli 2007.
40. Bert Wecker, Bakthiyor Karimov, Bakhtiyar Kamilov, Uwe Waller, Michael Matthies,
Helmut Lieth: Sustainable Aquaculture in Recirculating Systems – Feasibility Study for the Catchment Area of the Aral Sea. März 2007.
41. Michael Matthies (Hrsg.): Klimawandel. Oktober 2007.
42. Nina Hüffmeyer (Diplomarbeit): Modellierung von Zink in der Ruhr - Emissionspfade
und Belastungsanalyse. August 2006.
43. Jutta Wissing (Diplomarbeit): Georeferenzierte hydromorphologische Charakterisierung
von Flussgebieten. November 2006.
44. Jan Priegnitz (Diplomarbeit): Analyse von Koffein als Abwassermarker in
Fließgewässern. April 2007.
45. Johannes Witt (Diplomarbeit): Pharmacokinetics of sulfadiazine in pigs. Mai 2006.
46 Wibke Avenhaus (Diplomarbeit): Implementation von GREAT-ER mit ArcGIS und Arc
Hydro. August 2007.
47. Horst Malchow (Hrsg.): Komplexe Systeme und nichtlineare Dynamik in Natur und
Gesellschaft. Juli 2008.
48. Christian Ehling (Diplomarbeit): Abschätzung des Bioakkumulationspotentials
organischer Verbindungen in der menschlichen Nahrungskette. August 2008.
49. Michael Matthies (Hrsg.): Neue Problemstoffe (Emerging Pollutants). Oktober 2008.
50. Christiane Zarfl (Dissertation): Chemical Fate of Sulfadiazine in Soil: Mechanisms and
Modelling Approaches. November 2008.
Source: https://www.usf.uni-osnabrueck.de/fileadmin/DE/Institut/Publikationen/Schriftenreihe/050-zarfl.pdf
Human Reproduction Vol.19, No.10 pp. 2395–2400, 2004 Advance Access publication August 19, 2004 An increase in the absolute count of CD56dimCD161CD691NK cells in the peripheral blood is associated with a poorerIVF treatment and pregnancy outcome M.Y.Thum1,2,3,4, S.Bhaskaran2, H.I.Abdalla1, B.Ford2, N.Sumar2, H.Shehata3and A.S.Bansal2 1Lister Fertility Clinic, Lister Hospital, Chelsea Bridge Road, London SW1W 8RH, 2Immunology Department and 3Women HealthDepartment, Epsom and St Helier University Hospitals NHS Trust, Surrey, UK
August 2009 THE NEWSLETTER OF THE WASHINGTON STATE PSYCHOLOGICAL ASSOCIATION • VOLUME 64 • ISSUE 4 The Future of Psychology at Tri-State Bob Grubbs, PhD, WSPA Vice-President A s a relative newcomer to both the and practice styles to the lack of common protocols. Pacific Northwest and the language and patient treatment preferences. The keynote address for the second day of WSPA, a road trip to Idaho for In order to establish a good working the meeting, "Looking Forward: Future the Tri-State Psychological